Abstract
The biological properties of Agaricus brasiliensis mainly include immunostimulating and antitumour activities. This study evaluated the immunomodulating effect of A. brasiliensis mycelium (LPB) and its exopolysaccharides (EPS) on immunological parameters, such as phagocytosis of Candida albicans and nitric oxide (NO) production by infected macrophages, splenocytes proliferation in response to C. albicans. It also assessed the effects of the intake of LPB on the number of lymphocytes in the lymph node and spleen of treated animals and the TNF-α production. It was showed that the LPB and EPS had opposite effects; LPB had antiproliferative action and also reduced the NO levels produced by macrophages. However, the EPS showed an immune-stimulating effect potentiating the splenocytes proliferation and NO production from macrophages after stimulation with C. albicans. In addition, it also protected the macrophages from death induced by yeast. These data confirmed the immunomodulatory properties of LPB and its polysaccharides.
Introduction
Agaricus brasiliensis, also known as Agaricus blazei Murill (AbM), is an edible mushroom with medicinal properties, which exhibit both in vivo and in vitro antitumour activity, based mainly on modulation of the immune system (Santa et al., Citation2010; Wasser, Citation2002). Mushrooms are also known to have effective substances for antifungal, antiinflammatory, antiviral, antibacterial, hepatoprotective, antidiabetic, hypolipedemic, antithrombotic and hypotensive activities (Santa et al., Citation2010; Wasser, Citation1999; Wasser, Citation2002). This genus is rich in polysaccharides, which can be extracted from the mushroom fruiting bodies, mycelium and/or broth from submerged culture (Fan et al., Citation2003; Fujimiya et al., Citation1998). Polysaccharides are the best known and most potent mushroom-derived substances with antitumour and immunomodulating properties (Borchers, Keen, & Gershwin, Citation1999; Mizuno, Minato, & Ito, Citation1999; Ooi & Liu, Citation1999; Reshetnikov, Wasser, & Tan, Citation2001; Tzianabos, Citation2000; Wasser, Citation1999; Wasser, Citation2002). The major immunomodulatory effects of the polysaccharides include mitogenicity and activation of immune cells, such as lymphocytes, macrophages, dendritic cells and natural killer (NK) cells, resulting in the cytokines production (Bernardshaw, Hetland, Ellertsen, Tryggestad, & Johnson, Citation2005; Fujimiya et al., Citation1998; Lull, Wichers, & Savelkoul, Citation2005; Sorimachi, Akimoto, Ikehara, Inafuku, & Yamazaki, Citation2001; Wasser, Citation2002).
Some studies have reported that fungal polysaccharides increase the phagocytic activity, the production of reactive oxygen intermediates (ROI) and inflammatory mediators which are important during infections and tumours (Chen & Seviour, Citation2007; Wasser, Citation2002). On the other hand, another study demonstrated that peritoneal macrophages obtained from animals treated with the AbM mycelium showed unchanged phagocytic activity and lysosomal volume although reduced levels of nitric oxide (NO) and superoxide anion were found when compared to untreated animals (Santa et al., Citation2010). Phagocytosis is essential for the destruction of some pathogens, including Candida albicans, and during this process the macrophages produce ROI and NO, which are highly toxic to micro-organisms (Sasada, & Johnston, Citation1980). Resistance to C. albicans depends on a coordinated action of innate and adaptive immune defences, a process where phagocytosis by granulocytes and macrophages is crucial (Sasada & Johnston, Citation1980; Villar & Bagtzoglou, Citation2008).
Whilst it is known that different components of a mushroom extract may have synergistic activities, a major approach to therapeutic application has been the isolation, characterisation and administration of pure active mushroom constituents (Borchers, Keen, & Gershwin, Citation2004; Vickers, Citation2002). Thus, the present study was designed to evaluate the immunomodulatory effect of A. brasiliensis mycelium (LPB) and its polysaccharides on immune response induced by C. albicans in vitro, and also to assess the effects of long-term intake of LPB on the number of lymphocytes and plasmatic TNF-α levels in uninfected swiss mice.
Materials and methods
Chemicals and medium
Chemicals and culture medium were obtained from Sigma Chemical Co. (St. Louis, MO, USA), Merck (Darmstadt, Germany), Oxoid (Hampshire, England), Himedia (Mumbai, India). The wheat grains were bought in the local market. Fluorescent-labelled monoclonal antibodies – fluorescein isothiocyanate (FITC)-CD3 (clone 17A2), phycoerythrin (PE)-CD4 (clone H129.19), PE-CD8 (clone 53–6.7) and PE-CD19 (clone 1D3) – were purchased from BD Biosciences (California, CA).
C. albicans suspension
Yeast cells of C. albicans, strain ATCC 40175 obtained from INCQS (National Institute for Health Quality Control, Brazil) and maintained at −70°C, were defrosted and grown in Sabouraud-Dextrose-Agar medium (Himedia, India), at 35°C for 24 h. Cells were collected and washed with sterile pyrogen-free salt solution and adjusted to 1×106 yeasts ml−1. The viability of yeast cells was evaluated by phase microscopy (99% of viable cells).
Microorganism, pre-inoculum, inoculum preparation and submerged fermentations
All steps involved in the maintenance of A. brasiliensis LPB03 before the production of wheat cultivated with the mycelium were carried out as described previously Santa et al., Citation2010). A sample of the wheat grains was analysed by high-performance liquid chromatography, to confirm the absence of mycotoxins (aflatoxin B1, aflatoxin B2, aflatoxin G1, aflatoxin G2 and ochratoxin) (Harwitz, Citation2000).
Chow preparation
The cultured wheat grains were milled (particle size, <2 mm), and 950 g was supplemented with lipids (soy oil, 22 mL), proteins (egg albumin, 50 g), vitamins and minerals to fulfil nutritional needs (Reeves, Neilsen, & Fahey, Citation1993) and obtain the mushroom-cultivated chow. The wheat grains in natura were subjected to the same procedures, but without inoculation, to formulate the control chow.
All diets contained the same amounts of protein (230 g/kg), carbohydrate (660 g/kg), fat (40 g/kg), fibre (60 g/kg) and vitamins and minerals (10 g/kg) (Nuvilab®CR-1, Curitiba, Paraná, Brazil). The chows were prepared weekly and stored at 4°C until utilisation.
Animals and study design
Six-week-old male mice (Swiss strains) weighing approximately 17–24 g were used in all experiments in vivo and in vitro. In the in vivo experiments, Swiss mice were divided into three groups, with 10 mice per group. The animals were housed under temperature-controlled (22–25°C) conditions, with access to water and food ad libitum. Body weight was monitored weekly. The groups received the formulated chow for 14 weeks as follows: the control group (C) received control chow; the A100 group received 100% mushroom-cultivated chow and the A50 group received mixed chow (50% mushroom-cultivated chow and 50% control chow). To in vitro experiments were used two Swiss mice fed on a normal diet. All experiments were conducted in accordance with National Institutes of Health (NIH) guidelines on the welfare of experimental animals and with the approval of the Ethics Committee of the Federal University of Paraná (N. 70).
Flow cytometric analysis of cell-surface phenotype
Samples of 106 cells, obtained from spleen and lymph nodes, were suspended and incubated for 30 min at 4°C in PBS containing 2% PBS-S and FcγIIR block monoclonal antibody (mAb) (CD16/CD32) to avoid non-specific background staining. After the blocking step, the cells were labelled with 1 µL of FITC-CD3 and PE-CD4, PE-CD8 or PE-CD19 – conjugated mAb diluted in PBS-S for another 30 min at 4°C. After labelling, the cells were washed and suspended in PBS/0.1% formaldehyde for data acquisition in a FACSCalibur apparatus (Becton Dickinson, NJ). Forward scatter (FSC) and side scatter (SSC) were set to exclude erythrocytes and dead cells, and at least 104 lymphocyte subtypes were gated, based on FSC/SSC light scattering and positive staining with CD3 by using CellQuest software. Positive and negative populations were determined based on the control staining with an irrelevant IgG isotype labelled with FITC or PE. Once determined, quadrants were rigorously maintained for all analyses.
Cytokine determination
Levels of TNF-α in the plasma of animals were determined by flow cytometry using a FACSCalibur with CELLQuest software (BD Biosciences, California, CA). The results were expressed as picograms of cytokine per millilitre.
Peritoneal macrophage isolation and cell viability
Macrophages were harvested from Swiss mice three days after intraperitoneal (i.p.) injection of 3 ml of 3% thioglycolate medium. Recruited peritoneal macrophages were obtained by i.p. lavage with 5 ml of sterile phosphate buffer saline (PBS buffer – pH 7.2, NaCl 0.8% and KCl 0.02%). The peritoneal macrophages were collected by centrifugation (290 g, 4°C for 5 min), washed, and re-suspended in PBS after counting in a Neubauer chamber by optical microscopy using a Trypan blue solution (1%); with viability higher than 95%. A macrophage pool was obtained from 2 animals. Peritoneal macrophages were further purified by incubating peritoneal cells in tissue culture plates for 2 h and then washing three times with PBS to remove the non-adherent cells and used for the in vitro assays (Pizato et al., Citation2006).
Phagocytosis assays
Aliquots (1×106 cells in a final volume of 0.1 ml) of the peritoneal macrophage suspension were added to the wells of a 96-well, flat-bottomed tissue culture plate and left to adhere for 60 min. Adherent cells were then challenged with 1×106 yeast cells of C. albicans for 1 h at 37°C under 5% CO2. After this period, the slides were washed with complete culture medium, dyed with May-Grünwald-Giemsa and analysed under light microscopy. Results were expressed as phagocytic index calculated as the average number of attached plus ingested yeasts per phagocytising cell multiplied by proportion of phagocytising cells (Martins et al., Citation2008).
NO production
Peritoneal macrophages (1×106 in a final volume of 0.2 ml) were incubated for 24 h in the absence or presence of 1×106 yeast cells of C. albicans. Nitrite concentration was measured using Griess reagent according to Green, Tannenbaum, and Goldman (Citation1981) Equal volumes of cell culture supernatant and Griess reagent were incubated for 10 min at room temperature and the absorbance measured at 550 nm. NO2 concentration was determined from a standard curve generated using NaNO2. Nitrite production was expressed per micro-mol.
Preparation of spleen cells
BALB/C mice were killed and spleens were removed immediately and placed in cold Roswell Park Memorial Institute-1640 (RPMI-1640) media. Spleen cells were extracted and separated using a 5 ml syringe. Cell suspensions were washed three times in cold RPMI-1640 media, counted after the addition of 1% Trypan blue solution and adjusted to concentration. T and B lymphocytes were separated using the nylon wool enrichment method (Matthias, Carsten, Lourdes, & Stephan, Citation2001).
Lymphoproliferation assay
Lymphocyte cultures were prepared using a modification of the method described by Kim, Rasmussen, and Rudensky (Citation2006). Spleen cells from BABL/C mice at a density of 1×106 cells/ml were incubated or not with 1×106 yeast cells of C. albicans in 5% CO2 at 37°C for 24 or 48 hours in 96-well, flat-bottomed culture plates, washed twice with RPMI-1640 media. Three replicates of each group were made. Ten microlitres of methylthiazoletetrazolium (MTT – 5 mg/mL) was added 4 h prior to the termination of culture. After this incubation, 40 µl of 10% HCl solution was added to each well. The plates were incubated overnight at room temperature and optical densities were measured at 540 nm using an ELISA reader as described by Mosmann (Citation1983).
Statistical analysis
All data are reported as mean±SEM and are representative of three independent experiments. The means from different groups were compared by analysis of variance followed by the Bonferroni or Tukey test for unpaired values. p<0.05 was considered to be statistically significant.
Results
Agaricus is an important fungal genus that contains polysaccharides, which have several biological activities, especially antitumour action and immunomodulatory functions (Santa et al., Citation2010; Wasser, Citation2002). In order to determine the immunomodulatory action of the LPB and its exopolysaccharides (EPS), we evaluated the ability of these compounds to modulate phagocytosis, the viability and NO production by macrophages and the lymphoproliferative response induced by C. albicans. In vivo, the number of lymphocytes in lymphoid organs and systemic production of TNF-α were analysed. We have demonstrated that with the lower doses both compounds LPB and EPS were able to significantly inhibit the phagocytosis of C. albicans by macrophages in vitro (). The EPS was more effective than LPB, since similar inhibition was observed using a concentration 1000 times smaller. showed that highest concentrations of EPS (6.25 and 3.12 µg/mL) were able to potentiate the NO production induced by C. albicans, while the LPB-3 significantly inhibited it when assessed 24 hours after stimulation. In proliferation assay, in the presence of C. albicans, it was observed that the LPB significantly inhibited the splenocyte proliferation within 24 h, but this effect was stronger after 48 hours incubation with mycelium, showing antiproliferative action of the LPB in vitro (). On the other hand, the EPS at a concentration of 6.2 µg/mL was able to potentiate the proliferation induced by C. albicans within 24 h, but not in 48 h. Furthermore, incubation of splenocytes only with EPS (6.25 µg/mL) also caused cell proliferation in 24 h, but not in 48 hours (). shows the effect of LPB and EPS in cell death induced by C. albicans; our results demonstrated that higher concentrations of LPB (6.25 and 3.12 mg/mL) did not alter cell viability after 24 hours of stimulation. Only the lowest concentration (1.56 mg/mL) was able to protect cells from death. However, all concentrations of EPS were able to protect cells from the cytotoxicity induced by C. albicans.
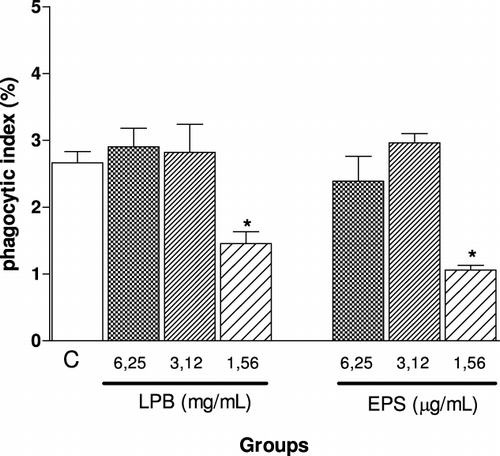
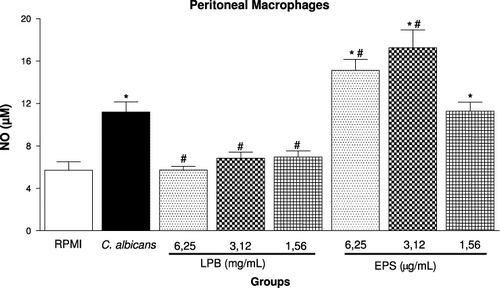
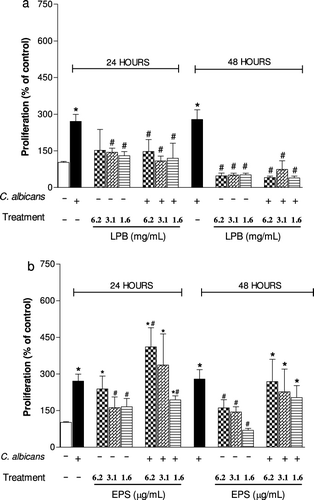
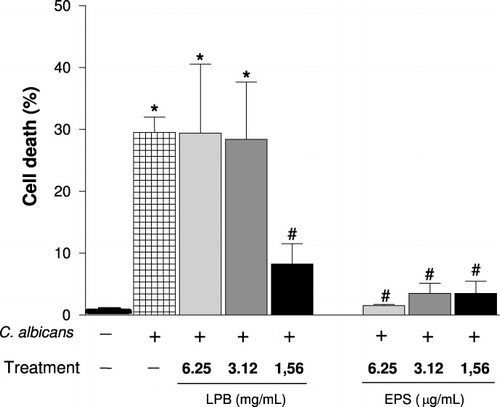
Regarding the in vivo experiments, we observed that there was a marked decrease in the number of T lymphocytes present in the lymph nodes, but not in the spleen of animals treated with dietary supplements (100 or 50%) from A mycelium for 14 weeks (). Moreover, the A100 group showed increase of B lymphocytes in both the lymph nodes and spleen, while the 50% Agaricus mycelium treatment was able to increase the B lymphocytes only in the spleen (). shows the subpopulations of T lymphocytes in the studied groups. Treatment with 100 and 50% Agaricus mycelium reduced the percentage of CD4+ cells () but not CD8+ cells (), in the lymph nodes, while the TNF-α level in the plasma was significantly higher in 100 and 50% Agaricus mycelium dietary supplemented animals, mainly with 50% supplementation ().
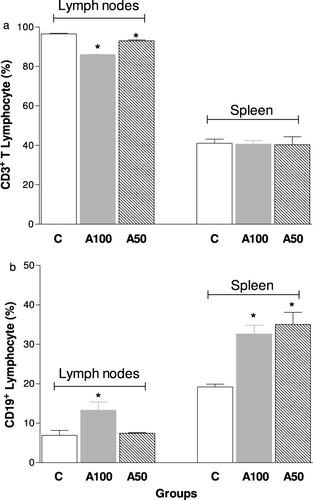
Discussion
Several studies have been already reported the medicinal actions of mushrooms including antitumour, immunomodulating, antioxidant, radical scavenging, cardiovascular, antihypercholesterolemia, antiviral, antibacterial, antiparasitic, antifungal, detoxification, hepatoprotective and antidiabetic effects (Gao, Zhou, Chen, Dai, & Ye, Citation2002; Gao, Zhou, Huang, & Xu, Citation2003; Gao, Lan, Dai, Ye, & Zhou, Citation2004; Ichinohe et al., Citation2010; Santa et al., Citation2010; Sullivan, Smith, & Rowan, Citation2006; Wasser, Citation2010; Zhang, Cui, Cheung, & Wang, Citation2007). The AbM is a well-known stimulant of the immune system, and it specifically targets NK cells, T-cells, B-cells and macrophages (Fujimiya et al., Citation1998; Lull et al., Citation2005; Santa et al., Citation2010; Wasser, Citation2002). Mushrooms contain large amounts of carbohydrates, including polysaccharides such as glucans and glycogen, monosaccharides and disaccharides (such as trehalose), sugar alcohols (such as mannitol) and chitin (Beelman, Daniel, & Chikthimmah, Citation2003; Zhang, Cui, Cheung, & Wang, Citation2007). Polysaccharides have shown particularly strong antitumour and immunostimulatory effects, being used in treating and preventing cancer (Fujimiya et al., Citation1998; Mizuno, Morimoto, Minato, & Tsuchida, Citation1998; Osaki, Kato, Yamamoto, Okubo, & Miyazaki, Citation1994; Zhang, Cui, Cheung, & Wang, Citation2007). Thus, this study evaluated the effect of the Agaricus mushroom mycelium (LPB) and its exopolysaccharide (EPS) in some in vitro immune functions and in vivo immunological parameters.
Macrophages are important source of cytokines, such as IL-1, IL-6, TNF-α, IL-10, IL-12 and NO (Sasada, & Johnston, Citation1980; Villar, & Bagtzoglou, Citation2008). Some studies related that polysaccharides from the mushroom with potent antitumour activity are also potent activators of macrophages in vitro and in vivo (Borchers, Krishnamurthy, Keen, Meyers, & Gershwin, Citation2008). In this study, it was showed that the LPB and EPS caused opposite effects on macrophage and splenocyte functions (NO production and proliferation, respectively). The LPB had antiproliferative action and also reduced the levels of NO produced by macrophages, while the EPS showed an immune-stimulating effect, potentiating the splenocytes proliferation at 24 hours and NO production from macrophages after stimulation by C. albicans. In addition, it also protected the macrophages from death induced by this yeast.
The antiproliferative effect of Agaricus mycelium has been described in other investigations, demonstrating protective effects against genetic damage (Barbisan et al., Citation2003; Bellini et al., Citation2006; Delmanto et al., Citation2001; Luiz, Jordao, Eira, Ribeiro, & Mantovani, Citation2003; Machado, Filho, Terezan, Ribeiro, & Mantovani, Citation2005; Martins, Jordao, Ribeiro, Ferreira, & Mantovani, Citation2002) and inhibitory effects on tumour cell growth, cell migration or tumour-induced neovascularisation by mushroom (Kimura, Kido, Takaku, Sumiyoshi, & Baba, Citation2004; Kobayashi et al., Citation2005; Takaku, Kimura, & Okuda, Citation2001). Regarding this, Kawamura and Kasai (Citation2007) reported that hemicellulase-treated A. blazei showed a strong antiproliferative effect and induced p38 mitogen-activated protein kinase-mediated cell growth delay and apoptosis in some cell lines. Thus, the data that the LPB inhibited splenocyte proliferation stimulated by yeast suggested that LPB has a general effect on cell growth, perhaps inhibiting a part of the intracellular signalling pathway or nutrient uptake. The inhibitory effect of LPB on NO production observed in this work () has been published in another in vivo study by our group. Recently, we have related that ex vivo assay with peritoneal macrophages obtained from A. brasiliensis biocultured-fed mice showed reduced levels of NO and anion superoxide, but did not show change in phagocytosis, lysosomal volume and H2O2 (Santa et al., Citation2010). Similarly, Wu et al. (Citation2007) reported that the dietary supplementation with 2 or 10% white button mushroom (Agaricus bisporus) did not alter the production of IL-6, TNF-α, prostaglandin E2, NO and H2O2 by peritoneal macrophage.
The polysaccharide from the mushroom is a potent stimulator of several cells; and the ability of bioactive polysaccharides and polysaccharide-bound proteins to modulate so many important immune cells may be due to the structural diversity and variability of these macromolecules (Ooi & Liu Citation1999; Zhang, Cui, Cheung, & Wang, Citation2007). Our data showed that the polysaccharides (EPS) enhanced the splenocyte proliferation () and inhibited the macrophages death induced by C. albicans (). These results are consistent with those reported by Sorimachi Ikehara et al. (Citation2001), who found that the AbM mycelium has also been shown to inhibit the cytopathic effects of Western equine encephalitis virus on VERO cells in culture. Also, studies had shown that the i.p. administration of the polysaccharide extracted (D fraction) from Grifola frondosa (Kodama, Murata, & Nanba, Citation2004) and of a polysaccharide peptide isolated from Coriolus versicolor mycelia (Chan & Yeung, Citation2006) enhanced macrophage activation and cytokine production. Furthermore, components or fractions of the Agaricus extract showed an increase in NO production in vitro (Mizuno, & Kawakami, Citation2006; Mizuno, Kawakami, Sakamoto, & Fujitake, Citation2003; Sorimachi, Akimoto, et al., Citation2001). Regarding this, fractions obtained by the precipitation of AbM fruiting body extracts with high ethanol concentrations stimulated macrophages to produce significantly high levels of NO (Sorimachi, Akimoto, et al., Citation2001). Moreover, A. brasiliensis-purified polysaccharides with molecular weight of approximately 6200 dalton enhanced the production of TNF-α and NO from a murine macrophage-like cell line, RAW264.7 (Mizuno et al., Citation2003). These data corroborate our results; we showed that the EPS induced a significant increase in NO production in macrophages stimulated with C. albicans (). Furthermore, Mizuno and Kawakami (Citation2006) suggested the putative mechanism of TNF-α and NO production by macrophages stimulated with the purified polysaccharide from A. brasiliensis, a hetero-glycan (ABHG). Theses authors demonstrated that the ABHG was recognised by TLR4 in macrophages similarly to LPS, and the NF-κB was activated by releasing from IkB and then transferred to the nucleus to bind a specific consensus sequence of DNA; then TNF-α mRNA was transcribed and the produced TNF-α was recognised by TNF-α receptor through an autocrine pathway, which led to enhanced NO production (Mizuno & Kawakami, Citation2006). This finding suggested that the increase of NO production by EPS-treated macrophage could be due to the fact that the EPS also stimulates TNF-α secretion in vitro (data not shown).
Concerning in vivo analysis, our data showed reduction in the number of CD4+ T-cells () and expansion of B lymphocytes () in the peripheral organs of mice receiving dietary supplementation with 100% and 50% Agaricus mycelium when compared to control animals. Other studies reported the immunomodulatory effects of AbM in mice, revealing an increase in the number of antibody-producing spleen cells (Nakajima et al., Citation2002), elevated serum IgG levels and T-cell number in spleen, as well as increased phagocytic capacity of polymorphonuclear leukocyte (PMN) (Chan et al., Citation2007). In addition, some researchers reported that the antitumour action of polysaccharides requires an intact T-cell component; their activity is mediated through a thymus-dependent immune mechanism. Mushroom compounds may enhance B- and T-cells’ activation, while there are also some that suppress these cells’ functions. Regarding this, a study showed that CD4 and CD8 positive cell population was significantly higher in mice orally administered with polysaccharide from AbM as compared with untreated animals (Mizuno et al., Citation1998). On the other hand, a polysaccharide isolated from the spores of Ganoderma lucidum significantly decreased the number of antibody-producing cells in mouse spleen and suppressed mitogen-induced proliferation of both B- and T-cells, when the polysaccharide was given i.p. for four days following the injection of the antigen (Bao, Fang, & Li, Citation2001). These discrepancies on in vivo models might be due to administration of a single component of the fungus, as polysaccharides, or whole fungus, and different methods of administration and concentration of AbM extract.
Moreover, our data showed that TNF-α levels in serum were increased in mice receiving dietary supplementation with 100% and 50% AbM mycelium, especially in 50% of supplemented (). These observations showed that an AbM moderate level supplementation (50%) exhibited a more improved stimulation of the immune response than a diet rich in AbM (100%). Other studies also showed this effect, where uninfected mice orally treated with the A. blazei extract exhibited an increase in serum levels of TNF-α and MIP-2, the murine analogue of IL-8, compared with the untreated animals (Bernardshaw et al., Citation2005). Oral administration of an aqueous extract of A. blazei fruiting body to normal Balb/cByJ mice markedly enhanced serum IgG levels and increased TNF-α secretion of splenocytes and the OVA-induced delayed-type hypersensitivity response, suggesting that oral intake of A. blazei stimulated both humoral and cellular immune responses (Bao et al., Citation2001).
In conclusion, the present study showed that LPB exhibited antiproliferative action and reduced the NO levels, while the exopolysaccharide showed a strong immune-stimulating effect, potentiating the splenocytes proliferation, NO production and phagocytic cells protected from death induced by C. albicans. Moreover, the dietary supplementation with LPB inhibited CD4+ T cells and expanded B lymphocytes in the peripheral organs, and increased the plasmatic levels of TNF-α production in the plasma. These data confirmed the immunomodulatory properties of LPB and its polysaccharides.
Acknowledgements
We are grateful to Conselho Nacional de Pesquisa (Process N° 475798/2003-1), Universidade Federal do Paraná, Paraná Tecnologia, Universidade Estadual do Centro-Oeste/PR and Universidade Federal do Pará/PA and FAPESPA for granting financial support.
References
- Bao, X., Fang, J., & Li, X. (2001). Structural characterization and immunomodulating activity of a complex glucan from spores of Ganoderma lucidum. Bioscience, Biotechnology, and Biochemistry, 65, 2384–2391.
- Barbisan, L.F., Scolastici, C., Miyamoto, M., Salvadori, D.M., Ribeiro, L.R., Eira, A.F., et al. (2003). Effects of crude extracts of Agaricus blazei on DNA damage and on rat liver carcinogenesis induced by diethylnitrosamine. Genetics and Molecular Research, 2, 295–308.
- Beelman, R.B., Daniel, J.R., & Chikthimmah, N. (2003). Bioactive components in Button mushroom Agaricus bisporus (J. Lange) Imbach (Agaricomycetidae) of nutritional, medicinal, and biological importance. International Journal of Medicinal Mushrooms, 5, 1–92.
- Bellini, M.F., Angeli, J.P., Matuo, R., Terezan, A.P., Ribeiro, L.R., & Mantovani, M.S. (2006). Antigenotoxicity of Agaricus blazei mushroom organic and aqueous extracts in chromosomal aberration and cytokinesis block micronucleus assays in CHO-k1 and HTC cells. Toxicology In Vitro, 20, 355–360.
- Bernardshaw, S., Hetland, G., Ellertsen, L.K., Tryggestad, A.M., & Johnson, E. (2005). An extract of the medicinal mushroom Agaricus blazei Murill differentially stimulates production of pro-inflammatory cytokines in human monocytes and human vein endothelial cells in vitro. Inflaammation, 29, 4–6.
- Borchers, A.T., Keen, C.L., & Gershwin, M.E. (1999). Mushrooms, tumors, and immunity. Proceedings of the Society for Experimental Biology and Medicine, 221, 281–193.
- Borchers, A.T., Keen, C.L., & Gershwin, M.E. (2004). Mushrooms, tumors and immunity (An update). Proceedings of the Society for Experimental Biology and Medicine, 229, 393–406.
- Borchers, A.T., Krishnamurthy, A., Keen, C.L., Meyers, F.J., & Gershwin, M.E. (2008). The immunobiology of mushrooms. Experimental Biology and Medicine, 233, 259–276.
- Chan, Y., Chang, T., Chan, C.H., Yeh, Y.C., Chen, C.W., Shieh, B., et al. (2007). Immunomodulatory effects of Agaricus blazei Murill in Balb/cByJ mice. Journal of Microbiology, Immunology and Infection, 40, 201–208.
- Chan, S.L., & Yeung, J.H. (2006). Polysaccharide peptides from COV-1 strain of Coriolus versicolor induce hyperalgesia via inflammatory mediator release in the mouse. Life Science, 78, 2463–2470.
- Chen, J., & Seviour, R. (2007). Medicinal importance of fungal b-(1/3), (1/6)-glucans. Mycological Research, 111, 635–652.
- Delmanto, R.D., Lima, P.L., Sugui, M.M., Eira, A.F., Salvadori, D.M., Speit, G., et al. (2001). Antimutagenic effect of Agaricus blazei Murrill mushroom on the genotoxicity induced by cyclophosphamide. Mutation Research, 496, 15–21.
- Fan, L., Soccol, A.T., Pandey, A., Germano, S., Rau, R., Pedroso, A., et al. (2003). Production of polysaccharide by culinary-medicinal mushroom Agaricus brasiliensis S. Wasser et al. LPB 03 (Agaromycetidae) in submerged fermentation and its antitumor effect. International Journal of Medicinal Mushrooms, 5, 17–23.
- Fujimiya, Y., Suzuki, Y., Oshiman, K., Kobori, H., Moriguchi, K., & Nakashima, H. (1998). Selective tumoricidal effect of soluble proteoglucan extracted from the basidiomycete, Agaricus blazei Murill, mediated via natural killer cell activation and apoptosis. Cancer Immunology, Immunotherapy, 46, 147–159.
- Gao, Y., Lan, J., Dai, X., Ye, J., & Zhou, S.H. (2004). A phase I/II study of Ling Zhi mushroom Ganoderma lucidum (W.Curt.:Fr.) Lloyd (Aphyllophoromycetideae) extract in patients with type II diabetes mellitus. International Journal of Medicinal Mushrooms, 6, 96–107.
- Gao, Y., Zhou, S., Chen, G., Dai, X., & Ye, J.A. (2002). Phase I/II study of a Ganoderma lucidum extract (Ganopoly) in patients with advanced cancer. International Journal of Medicinal Mushrooms, 24, 207–214.
- Gao, Y., Zhou, S.H., Huang, M., & Xu, A. (2003). Antibacterial and antiviral value of the genus Ganoderma P. Karst. Species (Aphyllophoromycetideae): A review. International Journal of Medicinal Mushrooms, 5, 235–246.
- Green, L.C., Tannenbaum, S.R., & Goldman, P. (1981). Nitrate synthesis in the germfree and conventional rat. Science, 212, 56–58.
- Harwitz, W. (2000). Official methods of analysis of the Association of Official Analytical Chemists. Gaitherburg, MD: Association of Official.
- Ichinohe, T., Ainai, A., Nakamura, T., Akiyama, Y., Maeyama, J., Odagiri, T., et al. (2010). Induction of crossprotective immunity against influenza A virus H5N1 by intranasal vaccine with extracts of mushroom mycelia. Journal of Medical Virology, 82, 128–137.
- Kawamura, M., & Kasai, H. (2007). Delayed cell cycle progression and apoptosis induced by hemicellulase-treated Agaricus blazei. Evidence-based Complementary and Alternative Medicine, 4, 83–94.
- Kim, M.J., Rasmussen, P. J., & Rudensky, A.Y. (2006). Regulatory T cells prevent catastrophic autoimmunity throughout the lifespan of mice. Nature Immunology, 8, 191–197.
- Kimura, Y., Kido, T., Takaku, T., Sumiyoshi, M., & Baba, K. (2004). Isolation of an antiangiogenic substance from Agaricus blazei Murill: its antitumor and antimetastatic actions. Cancer Science, 95, 758–764.
- Kobayashi, H., Yoshida, R., Kanada, Y., Fukuda, Y., Yagyu, T., Inagaki, K., et al. (2005). Suppressing effects of daily oral supplementation of beta-glucan extracted from Agaricus blazei Murill on spontaneous and peritoneal disseminated metastasis in mouse model. Journal of Cancer Research and Clinical Oncology, 131, 527–538.
- Kodama, N., Murata, Y., & Nanba, H. (2004). Administration of a polysaccharide from Grifola frondosa stimulates immune function of normal mice. Journal of Medicinal Food, 7, 141–145.
- Luiz, R.C, Jordao, B.Q., Eira, A.F., Ribeiro, L.R., & Mantovani, M.S. (2003). Mechanism of anticlastogenicity of Agaricus blazei Murill mushroom organic extracts in wild type CHO (K(1)) and repair deficient (xrs5) cells by chromosome aberration and sister chromatid exchange assays. Mutation Research, 528, 75–79.
- Lull, C., Wichers, H., & Savelkoul, H. (2005). Antiinflamatory and immunomodulating properties of fungal metabolites. Mediators of Inflammation, 2, 63–80.
- Machado, M.P., Filho, E.R., Terezan, A.P., Ribeiro, L.R., & Mantovani, M.S. (2005). Cytotoxicity, genotoxicity and antimutagenicity of hexane extracts of Agaricus blazei determined in vitro by the comet assay and CHO/HGPRT gene mutation assay. Toxicology In Vitro, 19, 533–539.
- Martins, P.R., Gameiro, M.C., Castoldi, L., Romagnoli, G.G., Lopes, F.C., Silva, A.V., et al. (2008). Polysaccharide-rich fraction of Agaricus brasiliensis enhances the candidacidal activity of murine macrophages. Memórias do Instituto Oswaldo Cruz, 103, 244–250.
- Martins, J.O., Jordao, B.Q., Ribeiro, L.R., Ferreira, A.E., & Mantovani, M.S. (2002). Anti-genotoxic effect of aqueous extracts of sun mushroom (Agaricus blazei Murill lineage 99/26) in mammalian cells in vitro. Food and Chemical Toxicology, 40, 1775–1780.
- Matthias, G., Carsten, W., Lourdes, P., & Stephan, G. (2001). Two-step negative enrichment of CD4+ and CD8+ T cells from murine spleen via nylon wool adherence and an optimized antibody cocktail. Journal of Immunological Methods, 258, 55–63.
- Mizuno, M., & Kawakami, S. (2006). An Immunomodulating Polysaccharide in Agaricus brasiliensis S. Wasser et al. (Agaricomycetideae) Activates Macrophages through Toll-like Receptor 4. International Journal of Medicinal Mushrooms, 8, 223–229.
- Mizuno, M., Kawakami, S., Sakamoto, Y., & Fujitake, N. (2003). Macrophages Stimulated by Polysaccharide Purified from Agaricus brasiliensis S. Wasser et al. (Agaricomycetideae) Enhance mRNA Expression of Th1 Cytokine Including IL-12 and 18. International Journal of Medicinal Mushrooms, 5, 383–389.
- Mizuno, M., Minato, K., & Ito, H. (1999). Anti-tumor polysaccharide from the mycelium of liquid-cultured Agaricus blazei Murrill. Biochemistry & Molecular Biology International, 47, 704–714.
- Mizuno, M., Morimoto, M., Minato, K., & Tsuchida, H. (1998). Polysaccharide from Agaricus blazei stimulate T-cell subsets in mice. Bioscience, Biotechnology, and Biochemistry, 62, 434–437.
- Mosmann, T. (1983). Rapid colorimetric assay for cellular growth and survival: Application to proliferation and cytotoxicity assays. The Journal of Immunological Methods, 16, 55–63.
- Nakajima, A., Ishida, T., Koga, M., Takeuchi, T., Mazda, O., & Takeuchi, M. (2002). Effect of hot water extract from Agaricus blazei Murill on antibody-producing cells in mice. International Immunopharmacology, 2, 1205–1211.
- Ooi, V.E., & Liu, F. (1999). A review of pharmacological activities of mushroom polysaccharides. International Journal of Medicinal Mushrooms, 1, 196–206.
- Osaki, Y., Kato, T., Yamamoto, K., Okubo, J., & Miyazaki, T. (1994). Antimutagenic and bactericidal substances in the fruit body of a Basidiomycete Agaricus blazei. Yakugaku Zasshi, 114, 342–350.
- Pizato, N., Bonatto, S., Piconcelli, M., Souza, L.M., Sassaki, G.L., Naliwaiko, K., et al. (2006). Fish oil alters T-lymphocyte proliferation and macrophage responses in Walker 256 tumor-bearing rats. Nutrition, 22, 425–432.
- Reeves, P.G., Neilsen, F.H.G., & Fahey, C.J. (1993). AIN-93 purified diets for laboratory rodents: final report of the American Institute of Nutrition ad hoc writing committee on the reformation of the AIN-76A rodent diet. Journal of Nutrition, 123, 1939–1951.
- Reshetnikov, S.P., Wasser, S.P., & Tan, K.K. (2001). Higher basidiomycota as a source of antitumor and immunostimulating polyssaccharides (Review). International Journal of Medicinal Mushrooms, 3, 361–394.
- Santa, H.S.D., Rubel, R., Fernandes, L.C., Bonatto, S.J.R., Bello, S.R., Monteiro, M.C., et al. (2010). Agaricus brasiliensis-enriched functional product promotes in mice increase in HDL levels and immunomodulate to Th1 CD4 + T subsets. Current Trends in Biotechnology and Pharmacy, 4, 957–970.
- Sasada, M., & Johnston Jr, R.B. (1980). Macrophage microbicidal activity. Correlation between phagocytosis-associated oxidative metabolism and the killing of Candida by macrophages. Journal of Experimental Medicine, 152, 85–98.
- Sorimachi, K., Akimoto, Y., Ikehara, K., Inafuku, A., & Yamazaki, S. (2001). Secretion of TNF-alpha, IL-8 and nitric oxide by macrophages activated with Agaricus blazei Murill fractions in vitro. The Cell Structure and Function, 26, 103–108.
- Sorimachi, K., Ikehara, Y., Maezato, G., Okubo, A., Yamazaki, S., Akimoto, K., et al. (2001). Inhibition by Agaricus blazei Murill Fractions of Cytopathic Effect Induced by Western Equine Encephalitis (WEE) Virus on VERO Cells in Vitro. Bioscience, Biotechnology, and Biochemistry, 65, 1645–1647.
- Sullivan, R., Smith, J. E., & Rowan, N.J. (2006). Medicinal mushrooms and cancer therapy. Translating a traditional practice into Western medicine. Perspectives in Biology and Medicine, 49, 159–170.
- Takaku, T., Kimura, Y., & Okuda, H. (2001). Isolation of an antitumor compound from Agaricus blazei Murill and its mechanism of action. Journal of Nutrition, 131, 1409–1413.
- Tzianabos, A.O. (2000). Polysaccharide immunomodulators as therapeutic agents: Structural aspects and biologic function. Clinical Microbiology Reviews, 13, 523–533.
- Vickers, A. (2002). Botanical medicines for the treatment of cancer: Rationale, overview of current data, and methodological considerations for phase I and II trials. Cancer Investigation, 20, 1069–1079.
- Villar, C.C., & Bagtzoglou, A.D. (2008). Immune defense mechanisms and immune enhancement strategies in oropharyngeal candidiasis. Expert Reviews in Molecular Medicine, 10, 1–18.
- Wasser, S.P. (2002). Medicinal mushrooms as a source of antitumor and immunomodulating polysaccharides. Applied Microbiology and Biotechnology, 60, 258–274.
- Wasser, S.P. (2010). Medicinal mushroom science: History, current status, future trends, and unsolved problems. International Journal of Medicinal Mushrooms, 12, 1–16.
- Wasser, S.P., & Weis, A.L. (1999). Medicinal properties of substances occurring in higher basidiomycetes mushrooms (review). International Journal of Medicinal Mushrooms, 1, 31–62.
- Wu, D., Pae, M., Re'n, Z., Guo, Z., Smith, D., & Meydani, S.N. (2007). Dietary supplementation with white button mushroom enhances natural killer cell activity in C57BL/6 mice. Journal of Nutrition, 137, 1472–1477.
- Zhang, M., Cui, S.W., Cheung, P.C.K., & Wang, Q. (2007). Antitumor polysaccharides from mushrooms: A review on their isolation, structural characteristics and antitumor activity. Trends in Food Science & Technology, 18, 4–19.