Abstract
Using the direct competitive chemiluminescent enzyme immunoassay (CLEIA), a rapid, highly selective and sensitive method for carbofuran determination was developed. Several physicochemical parameters such as chemiluminescent sensitiser, assay buffer, blocking substance and working concentration were optimised. Under the optimum conditions, the CLEIA method for carbofuran determination was generated successfully. The detection sensitivity of the IC50 value was 4.2 ng/mL at a practical working concentration ranging from 0.4 to 50 ng/mL, and the limit of detection (LOD) for carbofuran was 0.5 ng/mL. Recoveries of carbofuran spiked into vegetable, fruit and environmental samples were determined by CLEIA after sample matrix effect testing. Finally, the confirmation test between CLEIA and LC-MS/MS was finished. The results showed that CLEIA method had higher sensitivity than enzyme-linked immunosorbent assay method, and the LOD of a variety of samples reached the carbofuran's maximum residue limit value laid down by Codex Alimentarius Commission.
1. Introduction
Carbofuran (2,3-dihydro-2,2-dimethyl-7-benzofurany1 methylcarbamate) is a broad-spectrum carbamate used worldwide for the control of insects, acaroids and nematodes on a great variety of fruit, vegetables, forage, cotton and other crops (Yen, Hsiao, & Wang, Citation1997). As a cholinesterase inhibitor (IC50 in rats is 1.2–3.3×10−8 M), however, carbofuran is highly toxic to humans and wildlife through the oral and nasal routes of exposure (acute oral LD50 in rats is 8 mg/kg) (Gupta, Citation1994). Because of its widespread application and the resulting residue in the environment and food products, there is actually a growing concern about the related potential risk to human and non-target species.
Current analytical methods involving gas chromatography and high-performance liquid chromatography (Cochrane, Lanouette, & Trudeau, Citation1982; Concepcion, Gonzalo, Fernando, & Eugenio, Citation1993; Han & Wang, Citation2001) with mass spectrometry (MS) (Fu et al. Citation2005; Petropoulou, Gikas, Tsarbopoulos, & Siskos, Citation2006) for the detection of carbofuran are sensitive and reliable. However, these approaches require time-consuming sample cleanup, skilled analysts and expensive instruments which are available only in well-equipped laboratories, and they can hardly meet the need for the analysis of a large number of samples. On the other hand, immunochemical assay, alternative to those traditional methods, has been demonstrated as a simple, rapid and cost-effective tool for high sample throughout and on-site screening analysis in pesticide-monitoring programmes (Deng, Kolar, Ulrich, & Franek, Citation2002; Liang, Xie, Wang, Gui, & Zhu, Citation2012).
At present, reports on immunoassays for the determination of pesticide residues mainly focus on the enzyme-linked immunosorbent assay (ELISA) (Abad, Moreno, & Montoya, Citation1997; Gui, Jin, Sun, Guo, & Zhu, Citation2009; Javier, Francisco, Nuria, & Marco, Citation2009; Kondo et al. Citation2009; Lu et al. Citation2010; Piao et al., Citation2009; Wang et al., Citation2009; Zhu et al., Citation2008). Chemiluminescent immunoassay performs better than ELISA in assay sensitivity on account of signal enhancement, and thereby it plays a considerable role in medical testing and environmental monitoring nowadays (Klaus, Reinhard, & Michael, Citation2011; Knight, Crum, & Hardy, Citation2007; Quan, Chen, Zhan, & Zhang, Citation2011; Yu, Vdovenko, Wang, & Sakhorov, Citation2011). However, researches on chemiluminescent immunoassay for pesticide detection are rarely reported in the literature.
In this paper, an enhanced chemiluminescent enzyme immunoassay (CLEIA) was developed based on a monoclonal antibody (MAb) with high specificity against carbofuran. The concentration of MAb immobilised to the microplate and the concentration of BFNP-horseradish peroxidase (HRP) were optimised. After optimisation, the CLEIA was performed to detect carbofuran spiked in vegetable, fruit and environmental samples. Furthermore, ultra performance liquid chromatography–tandem mass spectrometry (UPLC-MS/MS) was applied for validation and evaluation of this immunoassay.
2. Experimental
2.1. Chemicals and instruments of immunoassay
Carbofuran standard (99.8%) was obtained from Hangzhou Geling Scientific Instrument (Hangzhou, China). Bovine serum albumin (BSA), ovalbumin (OVA), Tween 20, HRP, p-iodo-pheno (PIP) and Trometamol (Tris) were purchased from Sigma-Aldrich (St Louis, MO, USA). Luminol was supplied by Fluka Tryptone, and yeast extractor was obtained from Oxoid (Basingstoke, Hampshire, UK). All other chemicals and organic solvents were of analytical grade or better.
Phosphate-buffered saline (PBS), Tris–HCl buffer and PBST (0.01 M PBS, pH 7.4, with 0.05% Tween 20) were self-prepared. The synthesis of hapten [(3-[[2,3-dihydro-2,2-dimethyl-7-benzofuranyloxy]carbonyl]amino) propanic acid, BFNP] and the preparation of MAb have been described in the previous paper (Jin, Wu, Zhu, Chen, & Shou, Citation2006), based on the method of Abad and co-workers (Abad, Moreno, & Montoya, 1999). BFNP-HRP was prepared using the mixed-anhydride method (Rajkowski, Cittanova, Desfosses, & Jayle, Citation1977). The CLEIA was carried out in 96-well white polystyrene microplates (Corning, Lowell, Massachusetts, USA). Microplates were washed with a Dynex Plus microplate washer (Dynex Technologies, Inc., Chantilly, Virginia, USA), and chemiluminescent intensity (CI) was measured with a multifunctional plate reader (Tecan Genios, Hombrechtikon, Zurich, Switzerland).
2.2. CLEIA protocol
Microtitre plates were coated with 100 µL/well of the anti-BFNP MAb (6 µg/mL) in 100 mM Tris–HCl buffer (pH 7.2) at 37 °C for 2 h. Plates were washed four times with PBST and were then blocked by incubation with 300 µL/well of 1% tryptone in 50 mM Tris–HCl buffer (pH 7.2) for 30 min at 37 °C. After another wash step, 50 µL/well of standards or samples was added, followed by an addition of 50 µL/well of BFNP-HRP (0.006 µg/mL, 100 µL/well) in Tris–HCl buffer containing 1% tryptone. After incubation at 37 °C for 1 h, the plates were washed as before, and 200 µL/well of chemiluminescent enhancement solution was then added. The microtitre plates were placed for 5 min in darkness at room temperature, and immediately the CI was determined using the Tecan Genios multifunctional machine (Liu, Mu, & Zheng, Citation2007; Zhang, Wang, & Li, Citation2009).
2.3. Preparation of carbofuran standards and samples
From the 80 ng/mL stock solution of carbofuran in methanol, standards in the range of 0.08–80 ng/mL were prepared daily by serial dilution in the mixed solution of 0.05 M Tris–HCl buffer (pH 7.2) involving 2.5% methanol (v/v). Agricultural products (pear, cabbage, lettuce, carrot, bamboo shoot and green soybean) were purchased from a local market, and water sample was collected from Tiesha River in Hangzhou and soil was collected from Huajiachi campus, Zhejiang University. The extraction and purification of carbofuran in water, soil, pear, cabbage, lettuce, carrot, bamboo shoot and green soybean were performed by the following procedures. To 10 g of pre-dollied sample, 1 mL of carbofuran standard in acetonitrile was added, and after 1 h, 9 mL of acetonitrile was added. Following the oscillation on a vortex mixer for 1 min, the mixture was added with 1 g of sodium chloride and 4 g of magnesium sulphate, and was shaked again for 1 min. After centrifugation (5 min, 3,000 rpm), 1 mL of the supernatant was transferred and evaporated at 40 °C under a nitrogen stream. The dry residue was properly diluted with the mixed solution above-mentioned and oscillated on the vortex mixer for 1 min. At last 50 µL portions were used for the CLEIA test (Lehotay, Kok, Hiematra, & Bodegraven, Citation2005). Determination of spiked samples was conducted by interpolating their mean inhibition rates in the standard curve run on a same plate.
2.4. UPLC conditions
The carbofuran standards and sample extracts were chromatographed by Acquity UPLC system (Waters, Milford, MA, USA), using a UPLC BEH C18 column (1.7 µm, 50 mm×2.1 mm ID, Waters). A gradient mobile phase consisted of 0.1% (v/v) formic acid in water (solvent A) and acetonitrile (solvent B) as follows: 10% B (initial), 10–90% B (0–2 min), 90–100% B (2–4 min), 100% B (4–4.2 min) and 100–10% B (4.2–4.5 min). A subsequent re-equilibration time (3 min) should be performed before next injection. The eluate flow rate was 0.3 mL/min and the injection volume was 10 µL. Moreover, the column and sample temperatures were maintained at 30 and 25 °C, respectively.
2.5. MS/MS conditions
MS/MS analysis was performed using a Quattro Premier triple-quadrupole mass spectrometer equipped with an ESI source (Micromass, Manchester, UK). The parameters used for the mass spectrometry under the ESI+ mode were as follows: capillary voltage 1.0 kV, cone voltage 25 V, source temperature 120 °C, RF lens 0 V, cone gas 40 L/h, desolvation temperature 350 °C, desolvation gas 500 L/h. LM lens1 14 V, HM lens1 14 V, ion energy1 0 eV, entrance lens voltage 3.0 V, exit voltage 5.0 V, LM lens2 14 V, HM lens2 14 V and ion energy2 1.0 eV. The precursor ion/product ion transitions m/z 221.9/164.9 and m/z 221.9/122.8 were monitored, and m/z 164.9 was used for quantification.
3. Results and discussion
3.1. Optimisation of chemiluminescent enhancement solution
To get the better enhancement in chemiluminescent detection, the concentrations of luminol, PIP and H2O2 were optimised by evaluating the effects on the ratio of CI and noise (CI/N). The PIP can greatly enhance the CI of luminol because of the HRP-catalysed oxidation. The results indicated that the concentrations of luminol, H2O2 and PIP at 1.25, 2.5 and 0.15/mmol, respectively, were optimal for chemiluminescent enhancement solution.
3.2. CLEIA optimisation
Aiming at the improvement of immunoassay performance, the influence of several non-specific parameters on assay characteristics was examined. I50 was used as the primary criterion to evaluate the immunoassay performance, and maximum CI (CImax) analytical characteristics of standard curves and limit of detection (LOD) were also concerned.
3.2.1. Optimisation of coating buffer
Previous researches showed that non-specific adsorption would increase when the pH of coating buffer was acidic. Thus, to achieve a good coating effect, the preferred pH range for coating buffer is from alkalescence to alkaline. Besides, the relatively low concentration of coating buffer was good for the physical adsorption between protein and chemiluminescent plate (Gui, Citation2007). Accordingly, a concentration of 0.01 or 0.05 M buffer system was common in numerous papers (Casino, Morais, Puchades, & Maquieira, Citation2001; Harrison, Brimfield, & Nelson, Citation1989). In this experiment, the antibody was diluted with different coating buffer, and other conditions of CLEIA method were fixed. Subsequently, CLEIA tests were carried out. The results of comparison of standard curves and CI with different coating buffers are given in .
Table 1. Comparison of coating effect with different coating buffers.
As given in , the neutral distilled water coated plate had the highest CImax among all the coating system, and values of CImax based on buffers with pH of 7.2 and 7.4 were much higher than these derived from the alkalescence or weak-acidic buffers. These results did agree with the reported deduction that pH of the coating system had a significant effect on the antigen–antibody interaction (Liang, Jin, Gui, & Zhu, Citation2007).
When the deionised water was used for the test, the linear correlation coefficient (estimated by R2 value) of the standard curve was less than 0.85, which indicated that a certain concentration of the buffer system had a great balance on the reaction between the antibody and antigen.
What is more, the effect of the ionic strength of the coating media on the immunoassay performance was predominant. By comparison, Tris–HCl buffer (pH 7.2) at the concentrations of 0.05 and 0.1 M was relatively appropriate as a coating buffer, and so was PBS buffer (pH 7.4) at the concentrations of 0.01 and 0.05 M. Taking into account both standard curve and CImax, 0.1 M Tris–HCl buffer (pH 7.2) was selected as the optimal coating buffer for this immunoassay.
3.2.2. Optimisation of reaction buffer
A variety of reacting buffers were selected, and the results of comparison of different reaction buffer are showen in . The results indicated that the preferred pH range for reacting buffer was from neutral to alkalescence. And 0.01 M Tris–HCl buffer had no good cushion to the immunoassay, as the R2 value of the standard curves was below or around 0.92. On the basis of the result, it can be seen that CImax increased with pH to reach the maximum at comfort zone for the immunoassay and then diminished as pH increased.
Table 2. Comparison of effect with different reacting buffers.
In the comparison experiment, 0.05 M Tris–HCl buffer with pH 7.2, 0.1 M Tris–HCl buffer with pH 7.2 and 0.01 M PBS buffer with pH 7.4 had better performance in establishment of standard curves and CImax. However, there was no doubt that 0.05 M Tris–HCl buffer with pH 7.2 had advantage at the two indicators. Therefore, 0.05 M Tris–HCl buffer with pH 7.2 was chosen as reaction buffer for the further investigation.
3.2.3. Optimisation of blocking substance
Blocking substance is usually added to enhance the sensitivity, reduce well-to-well variability or to lower background for pesticide immunoassays. Their influence on carbofuran CLEIA method was also studied in the present study. In the experiment, 2% skimmed milk, 0.1% BSA, 10% FCS, 0.1% OVA, 1% tryptone, 1% gelatin and 2% yeast extractor were dissolved in 0.05 M Tris–HCl (pH 7.2) buffer as blocking solutions and the influence of which was investigated. The results are given in .
Table 3. Comparison of effect with different blocking substances.
The results indicated that the presence of blocking substance would affect the CI and the difference of blocking substances would impact the standard curves. Therefore, blocking substance which had better performance in the establishment of standard curves and minimal impact on the CI would be selected. Of these, 2% skimmed milk and 1% tryptone had better performance in the two indicators, and 1% tryptone was selected as blocking substance.
3.3. Determination of dynamic range and detection limit of carbofuran
Serial dilutions of carbofuran standard were prepared at concentrations of 0.08, 0.16, 0.31, 0.62, 1.25, 2.5, 5, 10, 20, 40 and 80 ng/mL and each was tested 10 times under the optimised assay conditions. By plotting the average value of inhibition rates against the logarithm of related concentration, a smooth sigmoid curve was obtained (see ). The analyte concentration from 0.31 to 20 ng/mL was selected for the linear dynamic range of this analytical method. Consequently, the regression equation between these seven concentrations and their corresponding inhibitory rate was established as: y=16.249 Ln(x) + 26.513, R2=0.9886.
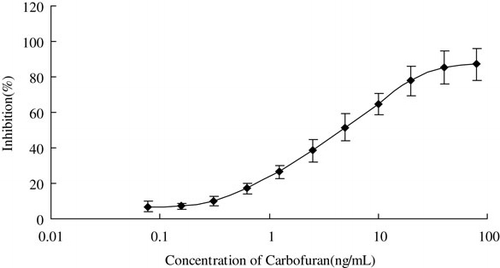
The LOD of carbofuran of CLEIA was estimated by analysing the blank solution. LOD was calculated based on a standard deviation (SD) value of the 10 replicate determinations. The detailed calculation is as follows: the inhibition rate (3 SD/RLU0×100%) was obtained first. Then the inhibition rate values were put in the regression equation to obtain the corresponding concentrations to the values of 3 SD/RLU0×100%, which was the value of LOD (RLU0 means the RLU value of blank solution). On the basis of the calculation, the LOD of carbofuran of CLEIA was 0.5 ng/mL.
3.4. Analysis of spiked samples
Recovery test is an effective approach to check the accuracy of a method. According to it, the immunoassay established above was performed to detect the carbofuran spiked in water, soil, pear, cabbage, lettuce, carrot, bamboo shoot and green soybean samples. For the sake of decreasing the interference of sample matrix on the assay performance, matrix dilution was considered as an effective means. But considering that too much dilution would simultaneously reduce the assay sensitivity due to the resulting shift of the dynamic range, suitable matrix dilutions for each kind of sample were chosen and used in the recovery tests (listed in ).
Table 4. Reproducibility and recovery of carbofuran from spiked samples.
A variety of samples were, respectively, fortified with carbofuran at different concentrations (see ), and non-spiked samples were set as negative controls. As shown in , the mean recoveries were 122, 111, 70, 140, 91, 89, 150 and 140% for cabbage, lettuce, carrot, pear, bamboo shoot, green soy bean, water and soil, respectively. Control samples without carbofuran were also systematically included in the analysis, and values were lower than those of the assay detection limit of corresponding sample. However, false positives were probably observed so that CLEIA-positive results should be confirmed by other methods using other instruments.
The results suggest that the detection sensitivity of CLEIA method was found to have improved fivefold than that of ELISA test based on the same MAb (Zhu et al., Citation2008), so that the detection limits for the majority of samples could meet Codex Alimentarius Commission's requirement. That is, the developed CLEIA displayed a great significance in the sensitivity promotion of immunoassay for carbofuran determination, which manifested that in food safety and environmental monitoring it would be a powerful screening tool for detecting carbofuran-positive samples.
However, owing to the enhanced sensitivity (the I10 value is much lower), the spike recovery and its coefficient of variation from this CLEIA applications were not satisfactory enough, compared with that from ELISA method (Zhu et al., Citation2008). The possible reason was that the matrix effect was amplified, and the chemiluminescent enhancement solution was not good enough. Further researches on the precision and the reproducibility of this CLEIA, as well as the deep optimisation, should be pursued.
3.5. Method validation
For method validation, water and carrot were stochastically selected for spiked recovery tests by means of both CLEIA and UPLC-MS/MS. Thirty-six water samples were spiked with 3.0, 5.0 and 10 ng/g of carbofuran, and 36 carrot samples were spiked with 15, 30 and 45 ng/g of carbofuran. Half of the samples were analysed by UPLC-MS/MS, while the remainder half were tested with the CLEIA method. The correlation results between UPLC-MS/MS and CLEIA are given in and .
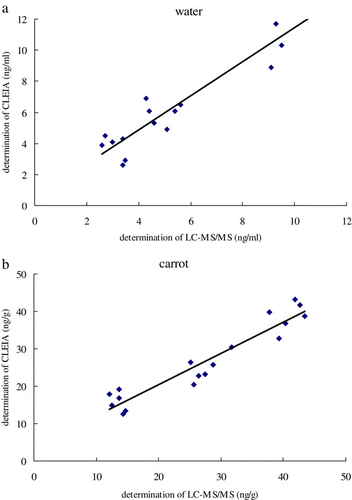
Table 5. Carbofuran residues in river water and carrot by LC-MS/MS and CLEIA.
Regression equation between assay results from CLEIA and UPLC-MS/MS was, respectively, established as follows: y=1.096x+0.460 (R2=0.925) for water sample and y=0.836x+3.680 (R2=0.906) for carrot samples. This means that the developed CLEIA displayed a good linear correlation to UPLC-MS/MS.
The validation of the method demonstrated that it showed good linear relationship to UPLC-MS/MS, which confirmed that the reported CLEIA provided an acceptable monitoring technique for routine analysis of carbofuran in agricultural and environmental samples. Meanwhile, in the prospective work, chemiluminescent tracer and enhancement solution were the key focus of our research to reduce the variation coefficient of CLEIA method.
Acknowledgements
This study was supported by Program of International Science & Technology Cooperation of Chinese Ministry of Science & Technology (2012DFA31140), Chinese Special Fund for Agro-scientific Research in the Public Interest (201203094), World Bank Loan Project in Jilin Province Agro-product Quality and Safety Project (2011-Z5), and Fundamental Research Funds for Chinese Central Public Interest Institution (0032011011). The authors gratefully thank Key Laboratory for Agro-Products Quality and Safety, Institute of Quality Standards & Testing Technology for Agro-Products, Chinese Academy of Agricultural Sciences for financial support.
References
- Abad, A., Moreno, M.J., & Montoya, A. (1997). A monoclonal immunoassay for carbofuran and its application to the analysis of fruit juices. Analytica Chimica Acta, 347, 103–110.
- Abad, A., Moreno, M.J., & Montoya, A. (1999). Development of monoclonal antibody based immunoassays to the N-methylcarbamate pesticide carbofuran. Journal of Agricultural and Food Chemistry, 47, 2475–2485.
- Casino, P., Morais, S., Puchades, R., & Maquieira, A. (2001). Evaluation of enzyme-linked immunoassays for the determination of chloroacetanilides in water and soils. Environmental Science & Technology, 35, 4111–4119.
- Cochrane, W.P., Lanouette, M., & Trudeau, S. (1982). Determination of aldicarb, aldicarb sulfoxide, aldicarb sulfone and carbofuran residues in water using high-performance liquid chromatography. Journal of Chromatography A, 243, 307–314.
- Concepcion, F., Gonzalo, P., Fernando, J., & Eugenio, R. (1993). High-performance liquid chromatographic analysis of carbofuran residues in tomatoes grown in hydroponics. Journal of Chromatography A, 643, 351–355.
- Deng, A., Kolar, V., Ulrich, R., & Franek, M. (2002). Direct competitive ELISA for the determination of polychlorinated biphenyls in soil samples. Analytical and Bioanalytical Chemistry, 373, 685–690.
- Fu, Z.M., Jin, M.C., Jin, Y.G., Yang, Y., Fu, C., & Jiao, X.L. (2005). Study on the determination of carbofuran residues in vegetable by GC/MS. Chinese Journal of Healthy Laboratory Technology, 15, 421–422.
- Gui, W.J. (2007). Industrial technique for pesticide rapid test ELISA kit – Preparation of triazophos and carbofuran ELISA kits (PhD thesis), Zhejiang University, China, 52 pp.
- Gui, W.J., Jin, M.J., Sun, L.F., Guo, Y.R., & Zhu, G.N. (2009). Residues determination of carbofuran in vegetables based on sensitive time-resolved fluorescence immunoassay. Food and Agricultural Immunology, 20, 49–56.
- Gupta, R.C. (1994). Carbofuran toxicity. Journal of Toxicology and Environmental Healthy, 43, 383–418.
- Harrison, R.O., Brimfield, A.A., & Nelson, J.O. (1989). Development of a monoclonal antibody based enzyme immunoassay method for analysis of maleic hydrazide. Journal of Agricultural and Food Chemistry, 37, 958–964.
- Han, L.J., & Wang, L.M. (2001). Determination of methamidophos, flolimat and carbofuran by GC method in two vegetables. Chinese Journal of Pesticide Science, 3, 84–92.
- Klaus, W., Reinhard, N., & Michael, S. (2011). Simultaneous determination of four different antibiotic residues in honey by chemiluminescence multianalyte chip immunoassays. Microchimica Acta, 173, 1–9.
- Knight, C.S., Crum, M.A., & Hardy, R.W. (2007). Evaluation of the LIAISON chemiluminescence immunoassay for diagnosis of syphilis. Clinical and Vaccine Immunology, 14, 710–713.
- Kondo, M., Yamashita, H., Uchigashima, M., Kono, T., Takemoto, T., & Miyake, S. (2009). Development of an enzyme-linked immunosorbent assay for residue analysis of the insecticide emamectin benzoate in agricultural products. Journal of Agricultural and Food Chemistry, 57, 359–364.
- Javier, R.A., Francisco, S.B., Nuria, S., & Marco, M.P. (2009). Development of an enzyme-linked immunosorbent assay for determination of the miticide bromopropylate. Journal of Agricultural and Food Chemistry, 57, 375–384.
- Jin, R.Y., Wu, J.X., Zhu, G.N., Chen, Z.L., & Shou, L.F. (2006). Development of the monoclonal antibody to carbofuran. Chinese Journal of Agro-Environment Science, 25, 1276–1280.
- Lehotay, S.J., Kok, A.D., Hiematra, M., & Bodegraven, P. (2005). Validation of a fast and easy method for the determination of residues from 229 pesticides in fruits and vegetables using gas and liquid chromatography and mass spectrometric detection. Journal of AOAC International, 88, 595–613.
- Liang, C.Z., Jin, R.Y., Gui, W.J., & Zhu, G.N. (2007). Enzyme-linked immunosorbent assay based on a monoclonal antibody for the detection of the insecticide triazophos: assay optimization and application to environmental samples. Environmental Science & Technology, 41, 6783–6788.
- Liang, X., Xie, R., Wang, C.M., Gui, W.J., & Zhu, G.N. (2012). Development of a broad-selective immunoassay for multi-residue determination of type II pyrethroids in West Lake water. Food and Agricultural Immunology. DOI: 10.1080/09540105.2011.641169
- Liu, Y.M., Mu, H.B., & Zheng, Y.L. (2007). Capillary electrophoretic immunoassay for alpha-fetoprotein with chemiluminescence detection. Journal of Chromatography B, 855, 280–285.
- Lu, Y., Xu, N., Zhang, Y., Liu, B., Song, Y., & Wang, S. (2010). Development of general immunoassays for pyrethroids: a new approach for hapten synthesis using pyrethroid metabolite analogue and application to food samples. Food and Agricultural Immunology, 21, 27–45.
- Petropoulou, S.E., Gikas, E., Tsarbopoulos, A., & Siskos, P. (2006). Gas chromatographic–tandem mass spectrometric method for the quantitation of carbofuran, carbaryl and their main metabolites in applicators' urine. Journal of Chromatography A, 1108, 99–110.
- Piao, Y.Z., Kim, Y.J., Kim, Y.A., Lee, H.S., Hammock, B.D., & Lee, Y.T. (2009). Development of ELISAs for the Class-specific determination of organophosphorus pesticides. Journal of Agricultural and Food Chemistry, 57, 10004–10013.
- Quan, Y., Chen, M., Zhan, Y., & Zhang, G. (2011). Development of an enhanced chemiluminescence ELISA for the rapid detection of acrylamide in food products. Journal of Agricultural and Food Chemistry, 59, 6895–6899.
- Rajkowski, K.M., Cittanova, N., Desfosses, B., & Jayle, M.F. (1977). The conjugation of testosterone with horseradish peroxidase and a sensitive enzyme assay for the conjugate. Steroids, 29, 701–713.
- Wang, C.M., Liu, Y.H., Guo, Y.R., Liang, C.Z., Li, X.B., & Zhu, G.N. (2009). Development of a McAb-based immunoassay for parathion and influence of the competitor structure. Food Chemistry, 115, 365–370.
- Yen, J.H., Hsiao, F.L., & Wang, Y.S. (1997). Assessment of the insecticide Carbofuran's potential to contaminate groundwater through soils in the subtropics. Ecotoxicology and Environmental Safety, 38, 260–265.
- Yu, F., Vdovenko, M., Wang, J., & Sakhorov, I. (2011). Comparison of enzyme linked-immunosorbent assays with chemiluminescent and colorimetric detection for determination of ochratoxin A in food. Journal of Agricultural and Food Chemistry, 59, 809–813.
- Zhang, Q., Wang, X., & Li, Z. (2009). Evaluation of alpha-fetoprotein (AFP) in human serum by chemiluminescence enzyme immunoassay with magnetic particles and coated tubes as solid phases. Analytica Chimica Acta, 631, 212–217.
- Zhu, G.N., Jin, M.J., Gui, W.J., Guo, Y.R., Jin, R.Y., Wang, C.M., et al. (2008). Development of a direct competitive enzyme-linked immunoassay for carbofuran in vegetables. Food Chemistry, 17, 1737–1742.