Abstract
Glycinin and β-conglycinin are major soybean allergens involved in food hypersensitivity. The study was aimed to investigate the soybean protein-induced intestinal immune responses and its possible mechanism. Balb/c mice were sensitised intragastrically with glycinin and β-conglycinin without an adjuvant for five weeks. Results showed that the sensitised mice displayed diarrhoea symptoms and jejunal morphological changes, including decreased villous height and thickened crypt depth. Both the histamine and immunoglobulin A levels in jejunum were increased in soybean allergen-sensitised mice. Moreover, the number of IgA+B cells and CD4+T cells in the jejunal lamina propria of sensitised mice were significantly increased. The expression levels of interleukin-4 and interferon-γ were increased, whereas the levels of IL-10 and transforming growth factor-β (TGF-β) were decreased in the jejunum mucosa. In conclusion, glycinin and β-conglycinin induce a mixed Th1/Th2 immune response. The suppression of IL-10 and TGF-β may play important roles in the development of intestinal allergic reactions.
1. Introduction
Soybean protein is a major source of dietary protein in food and feed industry because of its nutritional properties (Golubovic, van Hateren, Ottens, Witkamp, & van der Wielen, Citation2005; Hancock, Cao, Kim, & Li, Citation2000). However, allergic reactions induced by soybean protein are common in both human (Foucard & Yman, Citation1999) and farm animals (Dreau, Lalles, Philouzerome, Toullec, & Salmon, Citation1994; Guo, Piao, Cao, Ou, & Li, Citation2008). Glycinin and β-conglycinin are the two major storage proteins in soybean, constituting approximately 40% of the total seed protein (Friedman & Hilleman, Citation2001). Food allergy caused by the ingestion of glycinin and β-conglycinin has been documented in many reports, which ranged from mild gastrointestinal distress to suspected anaphylaxis (Dreau et al., Citation1994; Helm et al., Citation2000; Sun, Li, Dong, Qiao, & Ma, 2008a).
Several animal models have recently been built to investigate the possible mechanisms of these clinical symptoms (Guo et al., Citation2008; Sun et al., Citation2008a, Citation2008b; Zhao, Qin, Sun, Zhang, & Wang, Citation2010). The gastrointestinal mucosa is important in inducing the allergic responses to food proteins (Bannon, Citation2004). Previous studies have demonstrated that soybean protein contributes to disorder of immunological functions and intestinal damage in piglets (Hao, Zhan, Guo, Piao, & Li, Citation2009; Huang, Xu, Yu, Gao, & Liu, Citation2010) and rats (Guo et al., Citation2008). A combined T-helper cell (Th)1/Th2 response of the intestinal mucosa has been observed in glycinin-sensitised piglets (Hao et al., Citation2009). However, the relationship between immune responses and soybean protein-induced food allergy in the gut mucosal system is still not fully understood. It is also not obvious whether the differentiation of gut mucosal lymphoid cells involves in the immune responses induced by soybean allergens.
The objective of the current study was to investigate the intestinal immune responses induced by glycinin and β-conglycinin in a mice model established in our previous study (Liu, Feng, Xu, Wang, & Liu, Citation2008). Mice were gavaged with purified glycinin or β-conglycinin to prevent the influence of other allergenic components and were subjected to the assessment of diarrhoea. Levels of jejunal immunoglobulin A (IgA) and histamine, differentiation of intestinal lymphocytes and expression profile of cytokines were also measured for evaluation. Our study may provide valuable information in the study of soybean protein-induced allergy.
2. Materials and methods
2.1. Preparation of soybean protein
Purified soybean glycinin and β-conglycinin were offered by Prof. Shuntang Guo of the Food Institute of China Agricultural University (proteins were purified by fractional salting-out method with sodium and potassium salts of different pH, Patent No. 200410029589.4, China) and were used without further purification. The contents of glycinin and β-conglycinin were assayed by a protein assay kit (Product No. 500-0001, Bio-Rad, Hercules, USA) according to the manufacturer's instructions. The purity of protein was measured by sulphate polyacrylamide gel electrophoresis. Gels stained with Coomassie Brilliant Blue R-250 were scanned and analysed by Quantity One™ Software (Bio-Rad, Hercules, USA). The result confirmed that the samples contained more than 95% glycinin or β-conglycinin (Liu, Feng, Xu, Wang, & Liu, 2008). No endotoxin could be detected by the method of Hao et al. (Citation2009), which suggested that the protein samples were not contaminated.
2.2. Mice sensitisation and experimental protocol
Mice were sensitised with glycinin or β-conglycinin according to our previous study (Liu et al., Citation2008). Briefly, thirty second-generation female Balb/c mice (six-week-old, body weight, 22.0±2.41 g) were obtained form Zhejiang Chinese Medical University (Hangzhou, China). Mice were kept in stainless-steel wire cages (1 mice/cage) with soy-free feed and water ad libitum. Cages were put in a specific pathogen-free room maintained at 22±3.1°C, with a relative humidity of 55–65% and controlled lighting (10–12 h/day).
Mice were divided into three groups (n=10) and sensitised by an intragastric administration of 1 mg of glycinin or β-conglycinin [per mouse, 1.0 ml of a filter-sterilised solution containing 1 mg/ml glycinin or β-conglycinin, dissolved in phosphate buffered saline solution (PBS), pH 7.4] for 35 days without the use of an adjuvant. Control animals received an equal volume of sterile saline. The daily oral administration was performed using a 10-gauge stainless-steel animal feeding needle at 9 a.m. and was finished within 30–60 min by two assistants. Faecal samples were daily collected and immediately classified to determine the cases of diarrhoea. Three hours after the last sensitisation on day 35, all mice were anaesthetised and killed. Small intestinal tissue from the middle part of the jejunum was thoroughly flushed with saline to remove the intestinal contents and subsequently cut into 2 cm sections. One part of the samples was fixed in 4% paraformaldehyde/0.1 mol/l PBS, pH 7.2–7.4 at room temperature for 24 h and embedded in paraffin before cutting into 5 µm-thick sections. The remaining segments of the jejunum were quickly frozen in liquid nitrogen and stored at - 80°C until needed for histamine, IgA and cytokines analysis. The experimental procedures followed the guidelines of the Animal Care and Use Ethics Committee of Zhejiang University (Hangzhou, China).
2.3. Diarrhoea evaluation
Mice faeces were classified by the methods of Sun et al. (Citation2008b) and recorded daily throughout the entire experimental period.
2.4. Histology
Intestinal samples were prepared according to previously described histological procedures (Nakajima-Adachi et al., Citation2006). Jejunal sections of 5 µm thickness were stained using haematoxylin and eosin. Villus height and crypt depth were determined at a 40× magnification under a light microscope (BX-51, Olympus, Japan). Only villi fully attached to the lamina propria and with well-defined tips were measured. Ten villus heights and crypt depths of each sample were recorded from each section on randomly selected microscopic fields.
2.5. Determination of intestinal histamine level
The histamine level in the jejunum was measured by high-performance liquid chromatography (e2695, Waters, USA) according to Sun et al. (Citation2008a). Standard sample of histamine was purchased from Sigma (St. Louis, USA). The data were analysed by integration software (Empower2™, Waters, USA).
2.6. Immunohistochemistry
Intestinal tissue sections (5 µm thick) were dewaxed in xylene and rehydrated by graded ethanol (70%, 80%, 95% and 100%). Sections were heated at 100°C in Tris–HCl buffer with pH 9.0 for 100 s, and then washed 3×5′ (3 times, 5 min each) with PBS. Endogenous peroxidase activity was depleted with 3% hydrogen peroxide followed by washing for 15 min in PBS (5 min×3 times). The sections were incubated overnight at 4°C with rat monoclonal antibodies, directed anti-mouse IgA (BD Pharmingen™, Franklin Lakes, USA) diluted 1:100, anti-mouse cluster of differentiation (CD)4 (Dako, Glostrup, Denmark) diluted 1:50, anti-mouse CD8 (Dako) diluted 1:200 in PBS containing 0.05% bovine serum albumin, and PBS as negative control. After washing for 15 min in PBS (5 min×3 times), the sections were subsequently incubated with horseradish-peroxidase-labelled goat anti-rat IgG (Dako, Glostrup, Denmark) for 40 min at 37°C. Sections were washed again in PBS and incubated in liquid diaminabenzidin solution (Dako) for 3 min for the colour reaction. Sections were then counterstained with Harris' haematoxylin for 1 min, dehydrated by a graded series of alcohols, cleared with xylene, and finally covered. Lymphocytes stained for IgA+, CD4+and CD8+in the lamina propria were counted by using an image analysis system (Canon, Cambridge, England) at five randomly selected areas (about 0.04 mm2 each). Brownish-yellow stained lymphocytes were recognised as positive cells. The immune cells were expressed as cells/mm2 tissue. All the counting were performed and confirmed by a single observer.
2.7. Detection of intestinal IgA
About 0.5 g of jejunal mucosal samples was resolved in 1 ml of PBS, centrifuged at 5000 g for 15 min at 4°C after homogenisation. The supernatant was recovered and the protein content was quantified by a protein assay kit (500-0001, Bio-Rad, Hercules, USA).
IgA levels were detected by ELISA. Ninety-six-well polystyrene ELISA plates (Corning, New York, USA) were coated with 50 µl/well of goat anti-mouse IgA (Sigma, St. Louis, USA) and washed three times with PBS. Supernatants (100 µl) from washings of intestine were plated in serial twofold dilutions at 37°C for 1 h and washed five times. Wells were then incubated with a horseradish peroxidase-conjugated anti-mouse IgA (Sigma, St. Louis, USA) and 0.5 mg/ml O-phenylenediamine dihydrochloride for detection of the absorbance at 500 nm. Purified mouse IgA (Sigma, St. Louis, USA) was used as the standard and all samples were accessed for a minimum of three times. The results are expressed as the amount of IgA (µg) in 1 mg of total protein from intestinal mucus homogenate.
2.8. Quantification of cytokines interleukin-4, interleukin-10, interferon-γ and transforming growth factor-β
Total jejunum RNA was isolated using the Trizol reagent (Gibco BRL, Scotland, UK) according to the manufacturer's instructions. The complementary DNA was synthesised from 1 µg of total RNA in a 20 µl reaction mix by MultiScribe reverse transcriptase and random hexamers (Applied Biosystems, Carlsbad, USA) according to Vaali et al. (Citation2006). The primers of interferon-γ (IFN-γ) (forward primer: TCA AGT GGC ATA GAT GTG GAA GAA; reverse primer: TGG CTC TGC AGG ATT TTC ATG), interleukin-4 (IL-4) (forward primer: ACA GGA GAA GGG ACG CCA T; reverse primer: GAA GCC CTA CAG ACG AGC TCA), IL-10 (forward primer: GGT TGC CAA GCC TTA TCG GA; reverse primer: ACC TGC TCC ACT GCC TTG CT) and transforming growth factor-β (TGF-β) (forward primer: AGA GGT CAC CCG CGT GCT AA; reverse primer: TCC CGA ATG TCT GAC GTA TTG A) were according to Zaheer et al. (Citation2007). The real-time quantitative PCR was performed with the ABI Prism™ 7500 sequence detection system using SYBR™ green fluorescence (Applied Biosystems, Warrington, UK). The standard PCR conditions were used as described by Cardoso et al. (Citation2008). Relative values of expression were normalised using 18S rRNA (forward primer: TAA GTC CCT GCC CTT TGT ACA CA; reverse primer: GAT CCG AGG GCC TCA CTA AAC) as an endogenous internal standard and calculated using the formula x=2−ΔΔCt, where x=fold difference in amount of starting material between two groups (allergen-sensitised group versus non-sensitised group), ΔΔCt=ΔE−ΔC, and ΔE=Ctexp−Ct18Sexp, ΔC=Ctcontrol−Ct18Scontrol. ‘Ct’ stands for threshold cycle that is the fractional cycle number at which the amount of amplified target reaches a fixed threshold. Negative controls without RNA were also performed and analyses were determined in triplicate.
2.9. Statistical analysis
All data were expressed as the mean±SEM and analysed by the general linear model of SAS system (SAS Institute, Cary, USA). Polynomial contrasts were performed using Turkey's post test. Values of p<0.05 were considered as significant.
3. Results
3.1. Diarrhoea occurrence in Balb/c mice sensitised with soybean glycinin and β-conglycinin
To test if the oral administration of glycinin and β-conglycinin resulted in clinical manifestation of allergy, mice were checked daily for the occurrence of diarrhoea. Three of the glycinin-sensitised mice and four of the β-conglycinin-sensitised mice showed diarrhoea symptoms (pasty, semi-liquid, and liquid feces). The symptom appeared two days after oral sensitisation and lasted for less than five days. Control mice dosed with saline failed to present any symptoms.
3.2. Morphological structure and histamine content in the jejunum of mice sensitised with soybean proteins
To investigate the effects of glycinin and β-conglycinin on the small intestine after oral sensitisation, changes in the morphologic structure of the jejunum were determined (). Noticeable histological changes were observed with decreased villous height (p<0.01) and thickened crypt depth (p<0.01) in the glycinin- and β-conglycinin-sensitised mice compared to the control. The jejunal histamine levels were increased (p<0.01) in mice sensitised with glycinin and β-conglycinin compared with the saline-treated mice.
Table 1. Effects of soybean glycinin and β-conglycinin on jejunum morphology and histamine concentration in Balb/c mice.
3.3. Differential accumulation of lymphocytes in the jejunum
To have a quantitative characterisation of the allergic reactions induced in the damaged intestinal tissue, the number of IgA+, CD4+and CD8+lymphocytes in the jejunal lamina propria were counted. Numbers of IgA+B cells and CD4+T cells were increased (p<0.01) in the mice sensitised with glycinin or β-conglycinin ( and ). However, the number of CD8+T cells were not affected (p>0.05) in glycinin- and β-conglycinin-sensitised mice.
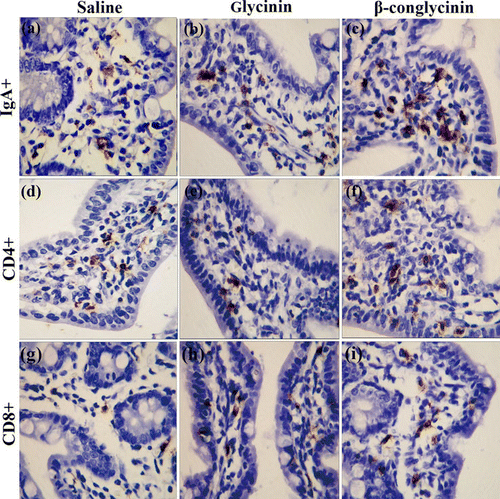
* represents p<0.05 and ** represents p<0.01 versus saline-dosed group.
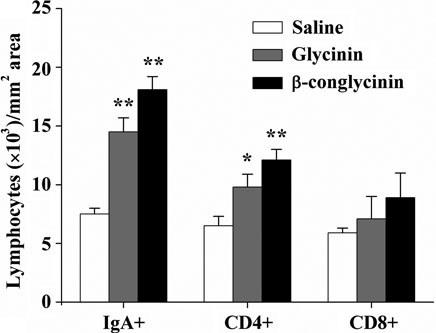
3.4. Level of intestinal mucus IgA
The increase of IgA+plasma cells in small intestine highlighted the role of IgA that participated in the pathogenesis of the food-induced intestinal inflammation. shows that the intestinal IgA levels in the sensitised mice were increased (p<0.01) compared with those of the control group (0.27 µg/mg and 0.37 µg/mg, respectively).
3.5. Differential cytokine expression in the jejunum
To investigate cytokine changes in the intestinal mucosa of glycinin- and β-conglycinin-sensitised mice, IL-4, IL-10, IFN-γ and TGF-β levels were measured (). mRNA levels of IL-4 and IFN-γ were increased (p<0.05) in the mice sensitised with glycinin and β-conglycinin, whereas levels of IL-10 and TGF-β mRNA expression were decreased (p<0.01).
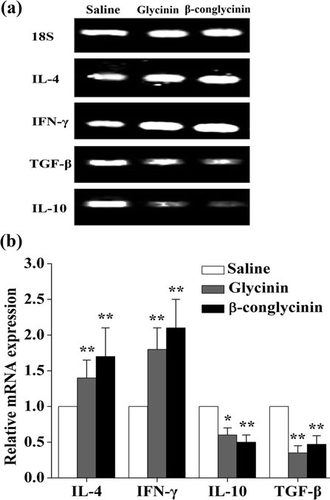
4. Discussion
Numerous immune cells and molecules scatter throughout the intestinal mucosa to provide efficient protection against pathogens and macromolecules. Abrogation of this barrier might be associated with intestinal inflammation and may promote food allergy (Chehade & Mayer, Citation2005). Animal models provide an effective method to evaluate allergens in vivo. We previously reported IgE-mediated anaphylactic reactions and intestinal damages induced by purified glycinin and β-conglycinin in a Balb/c mice model (Liu et al., Citation2008). In this study, the same Balb/c mice model was used to characterise the intestinal immune responses by intragastric gavage with glycinin and β-conglycinin. We also analysed the cytokine and lymphocyte subsets in intestinal mucosa to explore its potential mechanisms.
Diarrhoea is a common symptom of food allergies in infants and adults, which is also taken to evaluate the degree of sensitisation (Sun et al., Citation2008b). Many studies have showed reduced performance and increased occurrence of diarrhoea in soybean protein-sensitised piglet models (Dreau et al., Citation1994; Huang et al., Citation2010; Sun et al., Citation2008b). Similarly, our result showed increased diarrhoea in Balb/c mice after glycinin and β-conglycinin ingestion.
Our result demonstrated villous atrophy and crypt hypertrophy in jejunum of the mice sensitised with soybean proteins. Histological change of the intestine is a common phenomenon in animals fed soybean allergens (Dreau et al., Citation1994; Hao et al., Citation2009; Liu et al., Citation2008). The precise reason for such phenomenon is still not known. Liu et al. (Citation2008) indicated that IgE and IgG1 were involved in the allergic reactions of Balb/c mice. These antibodies activate mast cells, resulting in an excessive release of histamine, which may contribute to the intestinal damage and diarrhoea (Brandt et al., Citation2003; Guo et al., Citation2008). Additionally, substantial amounts of glycinin polypeptides escape digestion in the small intestine (Perez, Mills, Lambert, Johnson, & Morgan, Citation2000). The undigested remaining may damage the intestinal mucosa, disturb the absorption of nutrients and lead to diarrhoea (Dunsford, Knabe, & Haensly, Citation1989).
The intestinal damage allows antigens to pass rapidly into the systemic circulation and induces specific mediator release (Li, Schofield, Huang, Kleiner, & Sampson, Citation1999). Several studies have reported a significantly increased histamine level resulting from mast cell degranulation after antigen challenge in the sensitised animals (Guo et al., Citation2008; Liu et al., Citation2008; Sun et al., Citation2008b). Consistent with these reports, we found an increased histamine release in mice sensitised with soybean allergens. Histamine influences immune reactions through the activation of different T-cell cytokine profiles (Jutel et al., Citation2001), which drives the CD4+T cells towards either Th1 or Th2 subset. Therefore, we further examined the differentiation of intestinal lymphocyte subsets.
One important finding is that the numbers of CD4+T lymphocyte subpopulations were markedly increased in the jejunal lamina propria of mice gavaged with glycinin or β-conglycinin. This may partly agree with Sun et al. (Citation2008a), who demonstrated an increased number of peripheral blood CD4+lymphocytes in glycinin-sensitised piglets. However, differentiation of peripheral blood T lymphocytes mainly presents systemic immune responses. The measurement of lymphocytes in the intestinal mucosa provides a more precise picture of the local immune reactions. Since CD4+T cells are helper T cells which secret cytokines to control the immune response, the increased number of these cells may affect the intestinal inflammation. Interestingly, our study also demonstrated that the number of IgA+plasma cells elevated in the jejunum after oral sensitisation. IgA+cells in the intestinal lamina propria are a major source of IgA precursor cells for IgA production (Xu, Ayala, Monfils, Cioffi, & Chaudry, Citation1997). The development of IgA+B cells is dependent on the activation of T cells (Wittig & Zeitz, Citation2003). Therefore, the significantly increased proportions of CD4+T cells in jejunal lamina propria could, by itself, explain the increased density of IgA-producing cells.
IgA is well known as the major class of antibody present in the mucosal secretions. At least 80% plasma cells located in the intestinal lamina propria secret IgA, which defenses against ingested food antigens (Brandtzaeg, Citation2001). Our result showed an increase of intestinal IgA level in the jejunal mucosal sample, which is in agreement with the results obtained in pig models (Hao et al., Citation2009; Sun et al., Citation2008a).
IL-4 belongs to Th2 cytokines, which enhances humoral immunity while IFN-γ is Th1 cytokine, promoting cell-mediated immunity (Wittig & Zeitz, Citation2003). Our data showed that mRNA expression of IL-4 and IFN-γ were significantly increased in mice sensitised with soybean proteins compared with the saline-treated group. A previous study has revealed that serum IgE level and mRNA expression of IL-4 are correspondingly depended, suggesting that IgE-mediated hypersensitive responses partly depend on the production of IL-4 (Romagnani, Citation1990). Therefore, the increased expression of IL-4 may provide evidence for the elevated IgE level observed in our previous study (Liu et al., Citation2008). IL-4 and IFN-γ produced in spleen cells may play important roles in systemic immune responses to glycinin and β-conglycinin in sensitised mice (data not shown). Results of this study indicate that the production of Th2-type cytokines is also related to intestinal mucosal regulation mode (Huang et al., Citation2010; van Wijk & Knippels, Citation2007). In addition, although food allergy is recognised as Th2-type immune reactions, a mixed Th1/Th2 regulation mode in animal models has recently been described (Cardoso et al., Citation2008; Hao et al., Citation2009). Our data also suggest that a Th1/Th2 combined intestinal hypersensitive reaction was developed after oral administration of soybean proteins. Since the effects of Th1 or Th2 cytokines are counter-regulatory (Wittig & Zeitz, Citation2003), the results may indicate that continuous exposure to soybean allergens induced tolerance, which prevents persistent tissue injury (Cardoso et al., Citation2008). Future studies will be needed to identify the specific T cells activated by these cytokines.
TGF-β and IL-10 play a central role in mucosal immunity. TGF-β inhibits the proliferation of naive T cells, whereas IL-10 blocks antigen presentation and T cell activation; thus, they both function as immunosuppressive cytokines (Weiner, Citation2001). In the present study, we found a significant decrease of TGF-β and IL-10 mRNA expression in mice gavaged with soybean proteins. The result is in accordance with Cardoso et al. (Citation2008), who reported a reduced expression of both TGF-β and IL-10 mRNA in gut mucosal inflammatory induced by peanuts. The result suggests that the modulation of gut immune response and induction of inflammation by soybean proteins could be, at least, a consequence of the loss of regulatory mechanisms as demonstrated by the low expression of TGF-β and IL-10 (Hori, Haury, Coutinho, & Demengeot, Citation2002).
Ma, He, Sun, and Han (Citation2010) suggested that the cytokine production by lymphocytes from the mesenteric lymph nodes (MLN) played an important role in regulating the gut immunity of the glycinin-sensitised rats. However, considering the increased numbers of IgA+plasma cells and CD4+T cells, we determined the expression profile of cytokines in intestinal lamina propria rather than in the MLN. Similar studies have showed that cytokines secreted by T lymphocytes in the mucosa sections of the small intestine may control the immune response in humoral immunity (Bjersing, Telemo, Dahlgren, & Hanson, Citation2002; Liu et al., Citation2009). Moreover, Hao et al. (Citation2009) demonstrated that both IFN-γ and IL-4 were unregulated in jejunum tissues of piglets after oral β-conglycinin challenge. Additionally, CD4+effector T cells in the intestinal mucosa are mainly developed from the naive CD4+T cells in the MLN, which were activated by antigen-presenting cells via the afferent lymph (van Wijk & Knippels, Citation2007). Therefore, the soybean protein-induced local immune responses could be associated with cytokine production and lymphocyte differentiation in the MLN, which requires further investigation.
Generally, allergic reactions are difficult to induce by allergens without an adjuvant. However, the use of adjuvant may undermine the ability to discriminate between proteins concerning allergenic potential, resulting in false positives (Penninks & Knippels, 2001). Therefore, no adjuvant was used in the present trial. Recent studies have showed an increased level of allergen-specific IgE and histamine after administration of soybean allergens without the addition of an adjuvant in pig and rat models (Hao et al., Citation2009; Ma et al., Citation2010; Sun et al., Citation2008a, Citation2008b). Since the purities of glycinin or β-conglycinin used in these studies were 85–95%, we cannot rule out that the remaining part of soy impurities has adjuvant functions, which needs further research. It also seems difficult to induce allergic reactions without challenge after oral sensitisation (Guo et al., Citation2008; Ma et al., Citation2010). However, Akiyama et al. (Citation2001) reported typical allergic responses in both mice and rat models induced by daily ovalbumin administration. Our precious study also demonstrated allergy syndrome and anaphylactic reactions in BALB/c mice caused by long-term gavage with soybean proteins (Liu et al., 2007). Our result confirms that an animal model of allergy could be built after oral administration with soybean proteins.
The lack of data in other parts of intestine (duodenum, ileum and colon) may be acknowledged as a limitation in this study. However, Capobianco et al. (Citation2008) suggested that jejunum might be the main gut site in which allergen sensitisation occurs. Several studies have used jejunum to investigate the immune responses after exposure to allergenic food antigens (Hao et al., Citation2009; Sun et al., Citation2008a). The effects of soybean proteins on other intestinal segments would be assessed in future studies.
Taking together, our study demonstrates that oral sensitisation with soybean glycinin or β-conglycinin has induced intestinal allergic responses in the mice model, including histological changes of the intestine, increased levels of histamine and IgA antibodies, and an increase in IgA+and CD4+lymphocyte number in jejunal lamina propria. The results also suggest that the mixed Th1/Th2 immune response along with suppression of IL-10 and TGF-β production may play an important role in the development of intestinal hypersensitive reactions in soybean protein-sensitised mice.
Acknowledgements
The authors thank Jiang-Wu Tang and Xin-Ming Wu for their valuable technical support to this study. The work was financially supported by grants from National Science and Technology Committee and Zhejiang Provincial Natural Science Foundation, P R China (Grant No. Q12C200002).
References
- Akiyama, H., Teshima, R., Sakushima, J., Okunuki, H., Goda, Y., Sawada, J., et al. (2001). Examination of oral sensitization with ovalbumin in Brown Norway rats and three strians of mice. Immunology Letters, 78(1), 1–5.
- Bannon, G.A. (2004). What makes a food protein an allergen? Current Allergy and Asthma Reports, 4(1), 43–46.
- Bjersing, J.L., Telemo, E., Dahlgren, U., & Hanson, L.A. (2002). Loss of ileal IgA+plasma cells and of CD4+lymphocytes in ileal peyer's patches of vitamin A deficient rats. Clinical and Experimental Allergy, 130(3), 404–408.
- Brandt, E.B., Strait, R.T., Hershko, D., Wang, Q., Muntel, E.E., Scribner, T.A., et al. (2003). Mast cells are required for experimental oral allergen-induced diarrhea. Journal of Clinical Investigation, 112(11), 1666–1677.
- Brandtzaeg, P. (2001). Inflammatory bowel disease: clinics and pathology - Do inflammatory bowel disease and periodontal disease have similar immunopathogeneses? Acta Odontologica Scandinavica, 59(4), 235–243.
- Capobianco, F., Butteroni, C., Barletta, B., Corinti, S., Afferni, C., Tinghino, R., et al. (2008). Oral sensitization with shrimp tropomyosin induces in mice allergen-specific IgE, T cell response and systemic anaphylactic reactions. International Immunology, 20(8), 1077–1086.
- Cardoso, C.R., Teixeira, G., Provinciatto, P.R., Godoi, D.F., Ferreira, B.R., Milanezi, C.M., et al. (2008). Modulation of mucosal immunity in a murine model of food-induced intestinal inflammation. Clinical and Experimental Allergy, 38(2), 338–349.
- Chehade, M., & Mayer, L. (2005). Oral tolerance and its relation to food hypersensitivities. Journal of Allergy and Clinical Immunology, 115(1), 3–12.
- Dreau, D., Lalles, J.P., Philouzerome, V., Toullec, R., & Salmon, H. (1994). Local and systemic immune-responses to soybean protein ingestion in early-weaned pigs. Journal of Animal Science, 72(8), 2090–2098.
- Dunsford, B.R., Knabe, D.A., & Haensly, W.E. (1989). Effect of dietary soybean-meal on the microscopic anatomy of the small-intestine in the early-weaned pig. Journal of Animal Science, 67(7), 1855–1863.
- Foucard, T., & Yman, I.M. (1999). A study on severe food reactions in Sweden - is soy protein an underestimated cause of food anaphylaxis? Allergy, 54(3), 261–265.
- Friedman, M., & Hilleman, D.E. (2001). Economic burden of chronic obstructive pulmonary disease-Impact of new treatment options. Pharmacoeconomics, 19(3), 245–254.
- Golubovic, M., van Hateren, S.H., Ottens, M., Witkamp, G.J., & van der Wielen, L.A.M. (2005). Novel method for the production of pure glycinin from soybeans. Journal of Agricultural and Food Chemistry, 53(13), 5265–5269.
- Guo, P.F., Piao, X.S., Cao, Y.H., Ou, D.Y., & Li, D.F. (2008). Recombinant soybean protein beta-conglycinin alpha'-subunit expression and induced hypersensitivity reaction in rats. International Archives of Allergy and Immunology, 145(2), 102–110.
- Hancock, J.D., Cao, H., Kim, I.H., & Li, D.F. (2000). Effects of processing technologies and genetic modifications on nutritional value of full-fat soybeans in pigs. Asian-Australlasian Journal of Animal Sciences, 13(Special Issue), 356–375.
- Hao, Y., Zhan, Z.F., Guo, P.F., Piao, X.S., & Li, D.F. (2009). Soybean beta-conglycinin-induced gut hypersensitivity reaction in a piglet model. Archives of Animal Nutrition, 63(3), 188–202.
- Helm, R.M., Cockrell, G., Connaughton, C., Sampson, H.A., Bannon, G.A., Beilinson, V., et al. (2000). A soybean G2 glycinin allergen - 1. Identification and characterization. International Archives of Allergy and Immunology, 123(3), 205–212.
- Hori, S., Haury, M., Coutinho, A., & Demengeot, J. (2002). Specificity requirements for selection and effector functions of CD25(+)4(+) regulatory T cells in anti-myelin basic protein T cell receptor transgenic mice. Proceedings of the National Academy of Sciences of the United States of America, 99(12), 8213–8218.
- Huang, Q., Xu, H.B., Yu, Z., Gao, P., & Liu, S. (2010). Inbred Chinese Wuzhishan (WZS) minipig model for soybean glycinin and beta-conglycinin allergy. Journal of Agricultural and Food Chemistry, 58(8), 5194–5198.
- Jutel, M., Watanabe, T., Klunker, S., Akdis, M., Thomet, O.A.R., & Malolepszy, J., et al. (2001). Histamine regulates T-cell and antibody responses by differential expression of H1 and H2 receptors. Nature, 413(6854), 420–425.
- Li, X.M., Schofield, B.H., Huang, C.K., Kleiner, G.I., & Sampson, H.A. (1999). A murine model of IgE-mediated cow's milk hypersensitivity. Journal of Allergy and Clinical Immunology, 103(2), 206–214.
- Liu, X., Feng, J., Xu, Z.R., Wang, Y.Z., & Liu, J.X. (2008). Oral allergy syndrome and anaphylactic reactions in BALB/c mice caused by soybean glycinin and beta-conglycinin. Clinical and Experimental Allergy, 38(2), 350–356.
- Liu, C., Li, A., Weng, Y.B., Duan, M.L., Wang, B.E., & Zhang, S.W. (2009). Changes in intestinal mucosal immune barrier in rats with endotoxemia. World Journal of Gastroenterology, 15(46), 5843–5850.
- Ma, X., He, P., Sun, P., & Han, P. (2010). Lipoic acid: an immunomodulator that attenuates glycinin-induced anaphylactic reactions in a rat model. Journal of Agricultural and Food Chemistry, 58(8), 5086–5092.
- Nakajima-Adachi, H., Ebihara, A., Kikuchi, A., Ishida, T., Sasaki, K., Hirano, K., et al. (2006). Food antigen causes T(H)2-dependent enteropathy followed by tissue repair in T-cell receptor transgenic mice. Journal of Allergy and Clinical Immunology, 117(5), 1125–1132.
- Penninks, A.H., & Knippels, L.M. (2001). Determination of protein allergenicity: studies in rats. Toxicology Letters, 120(1–3), 171–180.
- Perez, M.D., Mills, E.N.C., Lambert, N., Johnson, I.T., & Morgan, M.R.A. (2000). The use of anti-soya globulin antisera in investigating soya digestion in vivo. Journal of the Science of Food and Agriculture, 80(4), 513–521.
- Romagnani, S. (1990). Regulation and deregulation of human ige synthesis. Immunology Today, 11(9), 316–321.
- Sun, P., Li, D.F., Dong, B., Qiao, S.Y., & Ma, X. (2008a). Effects of soybean glycinin on performance and immune function in early weaned pigs. Archives of Animal Nutrition, 62(4), 313–321.
- Sun, P., Li, D.F., Li, Z.J., Dong, B., & Wang, F.L. (2008b). Effects of glycinin on IgE-mediated increase of mast cell numbers and histamine release in the small intestine. Journal of Nutritional Biochemistry, 19(9), 627–633.
- Vaali, K., Puumalainen, T.J., Lehto, M., Wolff, H., Rita, H., Alenius, H., et al. (2006). Murine model of food allergy after epicutaneous sensitization: Role of mucosal mast cell protease-1. Scandinavian Journal of Gastroenterology, 41(12), 1405–1413.
- van Wijk, F., & Knippels, L. (2007). Initiating mechanisms of food allergy: Oral tolerance versus allergic sensitization. Biomedicine & Pharmacotherapy, 61(1), 8–20.
- Weiner, H.L. (2001). Induction and mechanism of action of transforming growth factor-beta-secreting Th3 regulatory cells. Immunological Reviews, 182, 207–214.
- Wittig, B.M., & Zeitz, M. (2003). The gut as an organ of immunology. International Journal of Colorectal Disease, 18(3), 181–187.
- Xu, Y.X., Ayala, A., Monfils, B., Cioffi, W.G., & Chaudry, I.H. (1997). Mechanism of intestinal mucosal immune dysfunction following trauma-hemorrhage: Increased apoptosis associated with elevated Fas expression in Peyer's patches. Journal of Surgical Research, 70(1), 55–60.
- Zaheer, A., Sahu, S.K., Wu, Y.H., Zaheer, A., Haas, J., Lee, K., et al. (2007). Diminished cytokine and chemokine expression in the central nervous system of GMF-deficient mice with experimental autoimmune encephalomyelitis. Brain Research, 1144, 239–247.
- Zhao, Y., Qin, G.X., Sun, Z.W., Zhang, B., & Wang, T. (2010). Effects of glycinin and beta-conglycinin on enterocyte apoptosis, proliferation and migration of piglets. Food and Agricultural Immunology, 21(3), 209–218.