Abstract
Analytical methodology to detect active ricin in food matrices is important because of the potential use of foodborne ricin as a terrorist weapon. Monoclonal antibodies (mAbs) that bind ricin were used as both capture and detection ligands in sandwich enzyme-linked immunosorbent assay (ELISA) and electrochemiluminescent (ECL) immunosorbent assays. ELISA employed two types of substrate for colorimetric or chemiluminescent detection. Although both fat content and protein content of samples influenced the recovery of ricin, the lower limit of detection (LOD) in ELISA and ECL systems permitted detection of 0.1 ng/mL for milk samples containing 0–4% fat. The assay systems detect pure ricin- or crude ricin-containing castor extract, but do not significantly respond to isolated ricin chains, heat-denatured ricin or the related agglutinin, Ricinus communis agglutinin 1 (RCA-1). Using the standard 96-well-plate formats, the assays detect less than 0.01% of an adult human lethal dose in a typical serving of milk.
Introduction
The toxic protein ricin (also known as RCA-II and RCA-60) is a Category B biothreat agent (CDC, Citation2000) found in the seeds (beans) of the castor plant, Ricinus communis. Ricin is a dimeric ribosome-inactivating protein (RIP), with a cell receptor-binding B chain that facilitates the uptake of the toxic, enzymatically active A chain (reviewed by Olsnes & Kozlov, Citation2001). It can be easily extracted from castor seeds or the widely available press-cake byproduct of castor oil production (Auld, Rolfe, & McKeon, Citation2001). The lethal dose (LD) is estimated as 2 mg for adult humans by enteric exposure (Ishiguro, Tanabe, Matori, & Sakakibara, Citation1992; reviewed by Doan, Citation2004). However, ricin is extremely toxic by parenteral routes of administration (LD50 about 3 µg/kg), and has been responsible for both intentional and apparently accidental poisoning (Misra, Citation2010). The documented criminal use of ricin and the evidence of bulk production of ricin for apparent terrorist use have been discussed (Olsnes, Citation2004), and analyses of milk and other targets for foodborne terrorism have been presented (Liu & Wein, Citation2008; Wein & Liu, Citation2005). One so-called ‘white powder incident’ involving ricin poisoning was extensively investigated using immunochemical and molecular screening techniques, as well as mass spectroscopic structural and activity assays for ricin (Schieltz et al., Citation2011). Because ricin, whether purified or as a crude powder, could be readily concealed in bulk foods by a terrorist, rapid screening assays for such matrices are needed.
Antibodies and enzyme-linked immunosorbent assays (ELISAs) have been reported previously (e.g., Brandon & Hernlem, Citation2009; Poli, Rivera, Hewetson, & Merrill, Citation1994; Shyu, Shyu, Liu, & Tang, Citation2002). Some methodology has been commercialised and incorporated into ELISA kits (e.g. Tetracore, Inc., Rockville, MD, USA). Other platforms for ricin analysis include immunosorbent systems with electrochemiluminescent (ECL) detection (Brandon, Citation2011, Citation2012; Brandon, Korn, & Yang, Citation2012; Garber & O'Brien, Citation2008), lateral flow devices (catalogued by Emanuel & Fruchey, Citation2007) and amplified or enhanced detection platforms such as immuno-PCR (He, McMahon, McKeon, & Brandon, Citation2010; He, McMahon, & Rasooly, Citation2012).
Approaches to shape-based detection of proteins include the use of aptamers, binding molecules based on random polymers. For example, constructs of DNA (Lamont, He, Warriner, Labuza, & Sreevatsan, Citation2011) or stabilised RNA (Forster et al., Citation2012) offer detection capability without the use of experimental animals in the generation or maintenance of the binding molecules. Other binders have been derived from antibodies, including single-chain camelid antibodies, and incorporated into analytical tools for ricin as well (Anderson, Bernstein, Swain, Zabetakis, & Goldman, Citation2010). Functional assays for ricin and other RIPs in food matrices have been developed, based on the inhibition of protein synthesis (Hale, Citation2001; He, Lu, Cheng, Rasooly, & Carter, Citation2008; Mei, Fredrickson, Lian, Jin, & Fan, Citation2006). Significant matrix effects on activity have been observed, and, in addition to impacting activity, food matrices and thermal processing can also affect the bioavailability of biothreat toxins (Cheng et al., Citation2008). Components of nonfat dry milk have been shown to bind to ricin and affect its uptake into cells (Rasooly, He, & Friedman, Citation2012). In the case of ricin, a related protein, Ricinus communis agglutinin 1 (RCA-1, also denoted RCA-120) is homologous to ricin at the DNA (Chen, He, & McKeon, Citation2005) and protein (Cawley, Hedblom, & Houston, Citation1978; Roberts, Lamb, Pappin, & Lord, Citation1985) levels, and cross-reactive in some commercially available ELISAs. Nonetheless, indirect structural assays have been shown to provide valid estimates of activity for toxins such as ricin (Lumor, Hutt, Ronningen, Diez-Gonzalez, & Labuza, Citation2011).
Previously, we prepared a panel of monoclonal antibodies (mAbs), including ricin-specific and RCA-1-specific nAbs, as well as generic antibodies that were equal in apparent affinity for ricin and RCA-1 (Brandon & Hernlem, Citation2009), and demonstrated their utility in ground beef and liquid egg matrices using ECL (Brandon, Citation2011, Citation2012; Brandon et al., Citation2012). In this study, several of the mAbs were used in sandwich format in ELISA and ECL immunosorbent assays and shown to provide reliable analyses of fresh milk samples for pure and crude ricin, while discriminating against non-toxic analogues.
Materials and methods
Buffers
Buffers used in this study were phosphate-buffered saline (PBS, 5 mM Na2PO4, 0.15 M NaCl, pH 7.0), PBS-0.05% Tween®-20 (PBST), and PBST plus 1% bovine serum albumin (BSA), 100 mM galactose and 0.01% thimerosal (BPTG).
Toxins
Ricin and RCA-1 were obtained from Vector Laboratories (Burlingame, CA, USA). Heat-denatured ricin was prepared by inactivating ricin (100 µg/mL) for 30 min at 90°C. Crude ricin was prepared from castor seeds as described previously (Brandon, Citation2011) and contained 2.4 mg/mL ricin. Analytical standards were diluted in BPTG from stock solutions to minimise binding via ricin lectin sites as well as nonspecific binding.
Milk analysis
Sample preparation
Five types of milk (products of Clover Stornetta Farms, Petaluma, CA, USA) were obtained from local retailers, labelled and abbreviated as follows: fat-free milk (0% milk), low-fat milk (1% milk), reduced fat milk (2% milk), whole milk with vitamin D (4% milk) and heavy whipping cream containing 40% fat (cream).
Spiking and analysis
Samples were spiked with ricin preparations using working dilutions (1–100 µg/mL) in PBS. Samples were diluted in BPTG for analysis. We verified that the inclusion of galactose in the buffer was sufficient to abrogate binding of ricin via its lectin sites to asialofetuin, without affecting the binding to the high-affinity capture mAbs used in this study.
ELISA plates
Colorimetric ELISAs were performed on Immulon 2HB or 4HBX polystyrene plates (Dynex, Chantilly, VA, USA). Chemiluminescent (CL) ELISAs utilised black polystyrene assay plates or strips (Fluotrac 600, Greiner Bio-One, Longwood, FL, USA). The black plates were preferred over conventional white plates to minimise cross-talk between wells. Plates or strip assemblies were coated by adsorption of proteins at 5 µg/mL in PBS, then blocked with BSA–PBS–Tween, treated with 2% sucrose, dried and stored desiccated as described previously (Brandon & Hernlem, Citation2009). In some assays, to compare formats, CL ELISA plates were rinsed with water after reading and developed a second time, using colorimetric reagents. The plate contents were then transferred to clear plates for colorimetric reading.
ECL plates
For ECL immunosorbent assays, standard 96-well (bare) plates and streptavidin (SA)-coated plates were obtained from Meso Scale Discovery [(MSD), Gaithersburg, MD, USA, Cat. Nos. L15XA-3 and L15SA-2, respectively]. Coating and storage procedures for both types of plates were as described previously (Brandon et al., Citation2012).
Antibodies and assays
mAbs and their conjugates
Antibodies were prepared, purified, characterised and biotinylated as described previously (Brandon & Hernlem, Citation2009). MAbs were designated by the corresponding hybridoma clone numbers. Tris(2,2′-bipyridyl)ruthenium (II) (Ru[bpy]3) conjugates of antibodies were prepared as described previously (Brandon, Citation2012; Brandon et al., Citation2012).
Immunosorbent assay procedures
ELISA
Direct-binding ELISAs on toxin-coated plates were conducted as described previously (Brandon & Hernlem, Citation2009). Sandwich ELISAs were conducted with 30-min incubations for samples, biotinylated detection mAb (b-mAb) and streptavidin-HRP, with intervening removal of well contents and five washes (Brandon et al., Citation2012). Unless noted, sandwich ELISA utilised mAb 1797 for capture and b-mAb 1443 (100 ng/mL) for detection. For CL detection, 200 µL of PS-Atto® substrate (Lumigen, Southfield, MI, USA) were added to each well, followed by incubation in the dark (2 min) and reading (0.1 s count/well). 3,3′,5,5′-Tetramethylbenzidine (TMB) substrate (Neogen, Lexington, KY, USA; 100 µL/well) was allowed to develop for 20 min, then stopped with 100 µL of 0.3 N HCl.
ECL
ECL assays utilised incubation times as for ELISA and were conducted as described previously (Brandon, Citation2012; Brandon et al., Citation2012), using mAb 1797 for capture on standard ECL plates and biotinylated mAb (b-mAb) 2147 for capture on SA plates. For detection, (Ru[bpy] 3)-mAb 1795 was utilised at 1 µg/mL or biotinylated mAb 1443 and (Ru[bpy]3) streptavidin as described previously (Brandon, Citation2011, Citation2012).
Plate reading and data analysis
For colorimetric assays, absorbance was read at 450 nm, with subtraction of the absorbance at 650 nm, using a SpectraMax M2 plate reader and SoftMax Pro 5.3 software (Molecular Devices, Sunnyvale, CA, USA). CL assays were read on a Wallac Victor 2 luminometer with Wallac 1420 Manager software (v3 rev 5, PerkinElmer, Shelton, CT, USA). ECL plates were read on a model Sector 2400 Imager, with Discovery Workbench v3.0 software (MSD). CL and ECL data were exported to Excel (Microsoft, Redmond, WA, USA) or SoftMax Pro for data analysis. Confidence intervals (CIs) were calculated using SlideWrite (Advanced Graphics Software, Rancho Santa Fe, CA, USA) to determine the lower limit of detection (LOD) graphically, and t-tests were evaluated using Excel or SlideWrite.
Other materials and methods
Asialofetuin was obtained from Sigma-Aldrich (St. Louis, MO, USA). Protein concentration was analysed using bicinchoninic acid (Smith et al., Citation1985), or spectrophotometrically for purified IgG (A280, 1 mg/mL=1.35).
Results and discussion
Antibodies used for sandwich ELISA
summarises the specificity of the mAbs used in this study and typical concentration of analyte resulting in 50% maximal response in sandwich ELISA (EC50) using the mAbs as capture reagents. It is notable that a good sandwich assay can be obtained with both capture and detection mAbs apparently directed against A-chain. All of the mAbs in this study were elicited with initial immunisations against A-chain and none of the selected antibodies showed significant binding to isolated B-chain immunosorbents. We have not determined specific epitopes for these antibodies, and it is possible that detection mAb(s) can bind to B-chain in the intact toxin because of the significant sequence homology between A- and B-chains (Roberts et al., Citation1985). It also seems likely that some epitopes of the isolated chains are less stable than those of the AB dichain toxin, stabilised by the interchain disulphides and other bonds that maintain the tertiary and quaternary structures of intact toxin. Dierks, Butler, & Richerson (Citation1986) documented that structural alteration of some proteins can occur when absorbed onto solid phases, and we speculate that plate coating may affect epitopes of the isolated chains selectively.
Table 1. Specificity of mAbs used in this study.Footnotea
shows that the sandwich ELISA system based on mAb 1797 capture and detection with biotinylated mAb 1443 was highly selective for ricin, distinguishing native ricin from heat-denatured toxin. Similarly, the ECL assay was highly selective for ricin (), and there was no measurable cross-reactivity with heat-denatured ricin (data not shown).
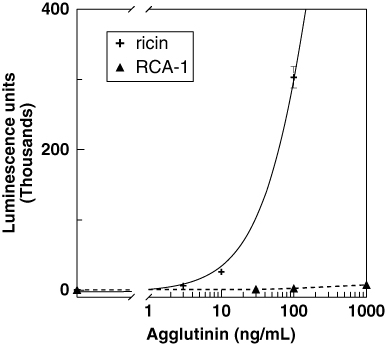
Table 2. Cross-reactivity of ricin analogues.Footnotea
Recovery of ricin from milk
In preliminary studies of recovery of pure and crude ricin from 4% milk, we compared samples analysed at various dilutions. Undiluted milk analysed yielded a lower recovery (39±18%), compared to 75±20% for a 1: 5 dilution (). The effect of dilution was similar for recovery of crude ricin in ELISA and for ECL assay as well; differences were significant (p<0.01). Further analyses were generally conducted with low dilution factors, 1:2–1:5, unless otherwise noted below. summarises the results of analysing spiked milk, using mAbs 1797 and 1443 in a colorimetric sandwich ELISA. In initial experiments, milk was spiked with ricin in BPT, but the recovery (74±20%) was not significantly different from that found using PBS as a diluent (67±20%, n=11, p>0.1).
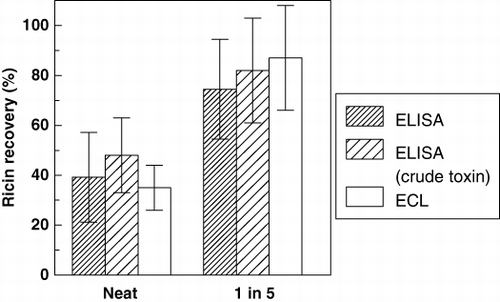
Table 3. Recovery of ricin in colorimetric ELISA.Footnotea
presents results obtained using CL detection. The recoveries were generally slightly higher than those obtained in the colorimetric assay, but the differences between the detection systems were not significant (p>0.1, paired t-test).
Table 4. Recovery of ricin in CL ELISA.Footnotea
We investigated the basis of the suppressed recovery of ricin from milk. and show that changes in the matrix – for example, concentration of BSA – influence the apparent recovery of pure ricin in both ELISA and ECL systems. The calibration curves are shifted to the left as the protein concentration is lowered, approximately a two-fold shift for a four-fold reduction in protein. Typical milk samples diluted 1:2 have 22 g/L protein compared to 10 g/L for BPTG. It seems likely that higher recoveries could be obtained by matching the matrix for the standards to that of the milk samples with respect to protein content and possibly other factors. However, for detection of potential contamination of milk, precise quantification of the analyte seemed unnecessary. and also show that high fat content suppressed the recovery of ricin. Recoveries from cream (40% fat) were 35–39%, compared to 58–87% for milk samples containing 0–4% fat.
Comparison of ELISA and ECL systems
shows the recovery of pure ricin from four milk samples by ECL immunosorbent sandwich analysis. A comparison of the mAb 1797/b1443 sandwich ELISA vs. ECL immunosorbent assays is summarised in . The mean recoveries were not significantly different in the three assay systems (p>0.05 for all pairs).
Table 5. Recovery of ricin in ECL immunosorbent assay.Footnotea
Table 6. Recovery of ricin in four milk types.Footnotea
Limits of detection
The LOD for ricin in the milk matrix was determined by graphical analysis of assay results for samples spiked with ricin or crude ricin. – show representative results in the ELISAs and ECL assay. The LOD in all three systems, for pure or crude ricin, was generally 0.1–0.2 ng/mL, with 0.1 ng/mL the lowest spiking level. When CL assays were redeveloped using colorimetric reagents, a higher LOD was obtained (0.146±0.088 for CL detection; 0.246±0.18 for colorimetric, n=24, paired t-test, p<0.01).
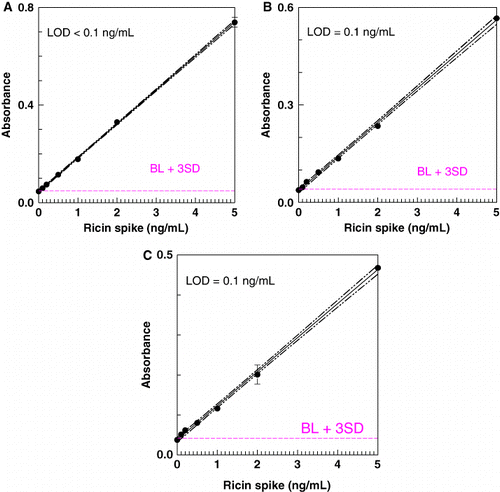
Conclusion
The high apparent affinity of capture antibodies enables reproducible binding and reliable measurement of ricin in milk matrices, despite the presence of cross-reactive and interfering components. The results indicate that ELISA and ECL immunosorbent assays provide comparable sensitivities, as configured in this study. It is likely that recoveries can be improved by adjusting the assay buffer to be more similar to the sample or by fractionating the samples to remove interferences. However, reproducible results are obtained without any adjustments. In the present implementation of immunosorbent detection, an equal volume of analyte is added to wells containing a buffer that minimises nonspecific and lectin-related binding of ricin. Based on an estimated adult human LD of 2-mg ricin, and assuming no additional matrix or processing effect on ricin toxicity and bioavailability, the required assay sensitivity to detect one human LD/serving (250 mL) is 8 µg/mL. The assays described in this paper have LODs of ≤ 0.1 ng/mL, providing a sensitivity 5- to 10,000-fold greater than this level.
Acknowledgements
The technical assistance of Uyen Tran and the participation of students Gordon C. Yang (American Chemical Society SEED Project) and Paweena Lizarraga and Christina Nguyen (City College of San Francisco) in these studies are greatly appreciated. Mark Smith (Meso Scale Discovery) is gratefully acknowledged for facilitating the loan of a Sector 2400 Imager. M. Travis Harrison (SRI Intl., Palo Alto, CA, USA) kindly facilitated our initial ECL studies of the milk matrix using his Imager. Mention of trade names or commercial products in this article is solely for the purpose of providing specific information and does not imply recommendation or endorsement by USDA. USDA is an equal opportunity provider and employer.
References
- Anderson, G. P., Bernstein, R. D., Swain, M. D., Zabetakis, D., & Goldman, E. R. (2010). Binding kinetics of antiricin single domain antibodies and improved detection using a B chain specific binder. Analytical Chemistry, 82, 7202–7207. 10.1021/ac100961x
- Auld, D. L., Rolfe, R. D., & McKeon, T. A. (2001). Development of castor with reduced toxicity. Journal of New Seeds, 3, 61–69. 10.1300/J153v03n03_03
- Brandon, D. L. (2011). Detection of ricin contamination in ground beef by electrochemiluminescence immunosorbent assay. Toxins, 3, 398–408. 10.3390/toxins3040398
- Brandon, D. L., Korn, A. M., & Yang, L. L. (2012). Detection of ricin contamination in liquid egg by electrochemiluminescence immunosorbent assay. Journal of Food Science, 77, T83–T88. 10.1111/j.1750-3841.2012.02627.x
- Brandon, D. L. (2012). Electrochemiluminescence immunosorbent assay of ricin in ground beef: Biotinylated capture antibodies and matrix effects. Food and Agricultural Immunology, 23, 329–337. 10.1080/09540105.2011.629315
- Brandon, D. L., & Hernlem, B. J. (2009). Development of monoclonal antibodies specific for Ricinus agglutinins. Food and Agricultural Immunology, 20, 11–22. 10.1080/09540100802626487
- Cawley, D. B., Hedblom, M. L., & Houston, L. L. (1978). Homology between ricin and Ricinus communis agglutinin: Amino terminal sequence analysis and protein synthesis inhibition studies. Archives of Biochemistry and Biophysics, 190, 744–755. 10.1016/0003-9861(78)90335-1
- CDC. 2000. Biological and chemical terrorism: Strategic plan for preparedness and response. Recommendations of the CDC Strategic Planning Workgroup 517. MMWR Recommendations and Reports, 49, 1–14.
- Chen, G. Q., He, X., & McKeon, T. A. (2005). A simple and sensitive assay for distinguishing the expression of ricin and Ricinus communis agglutinin genes in developing castor seed (R. communis L.). Journal of Agricultural and Food Chemistry, 53, 2358–2361. 10.1021/jf040405t
- Cheng, L. W., Onisko, B., Johnson, E. A., Reader, J. R., Griffey, S. M., Larson, A. E., Carter, J. M. (2008). Effects of purification on the bioavailability of botulinum neurotoxin type A. Toxicology, 249, 123–129. 10.1016/j.tox.2008.04.018
- Dierks, S. E., Butler, J. E., & Richerson, H. B. (1986). Altered recognition of surface-adsorbed compared to antigen-bound antibodies in the ELISA. Molecular Immunology, 23, 403–411. 10.1016/0161-5890(86)90138-0
- Doan, L. G. (2004). Ricin: Mechanism of toxicity, clinical manifestations, and vaccine development. A review. Journal of Toxicology. Clinical Toxicology, 42, 201–208. 10.1081/CLT-120030945
- Emanuel, P. A., & Fruchey, I. R. (2007). Biological detectors: Market survey. Aberdeen Proving Ground, MD: Edgewood Chemical Biological Center, 1–316.
- Forster, C., Zydek, M., Rothkegel, M., Wu, Z., Gallin, C., Gessner, R., Furste, J. P. (2012). Properties of an LNA-modified ricin RNA aptamer. Biochemical and Biophysical Research Communications, 419, 60–65. 10.1016/j.bbrc.2012.01.127
- Garber, E. A. E., & O'Brien, T. W. (2008). Detection of ricin in food using electrochemiluminescence-based technology. Journal of AOAC International, 91, 376–382.
- Hale, M. L. (2001). Microtiter-based assay for evaluating the biological activity of ribosome-inactivating proteins. Pharmacology & Toxicology, 88, 255–260. 10.1034/j.1600-0773.2001.d01-113.x
- He, X., Lu, S., Cheng, L. W., Rasooly, R., & Carter, J. M. (2008). Effect of food matrices on the biological activity of ricin. Journal of Food Protection, 71, 2053–2058.
- He, X., McMahon, S., McKeon, T. A., & Brandon, D. L. (2010). Development of a novel immuno-PCR assay for detection of ricin in ground beef, liquid chicken egg and milk. Journal of Food Protection, 73, 695–700.
- He, X., McMahon, S., & Rasooly, R. (2012). Evaluation and comparison of three enzyme-linked immunosorbent assay formats for the detection of ricin in milk and serum. Biocatalysis and Agricultural Biotechnology, 1, 105–109. 10.1016/j.bcab.2011.08.016
- Ishiguro, M., Tanabe, S., Matori, Y., & Sakakibara, R. (1992). Biochemical studies on oral toxicity of ricin. IV. A fate of orally administered ricin in rats. Journal of Pharmacobio-Dynamics, 15, 147–156. 10.1248/bpb1978.15.147
- Lamont, E. A., He, L., Warriner, K., Labuza, T. P., & Sreevatsan, S. (2011). A single DNA aptamer functions as a biosensor for ricin. Analyst, 136, 3884–3895. 10.1039/c1an15352h
- Liu, Y., & Wein, L. M. (2008). Mathematically assessing the consequences of food terrorism scenarios. Journal of Food Science, 73, M346–M353. 10.1111/j.1750-3841.2008.00874.x
- Lumor, S. E., Hutt, A., Ronningen, I., Diez-Gonzalez, F., & Labuza, T. P. (2011). Validation of immunodetection (ELISA) of ricin using a biological activity assay. Journal of Food Science, 76, C112–C116. 10.1111/j.1750-3841.2010.01943.x
- Mei, Q., Fredrickson, C. K., Lian, W., Jin, S., & Fan, Z. H. (2006). Ricin detection by biological signal amplification in a well-in-a-well device. Analytical Chemistry, 78, 7659–7664. 10.1021/ac0610006
- Misra, N. (2010). Deaths on a ship due to inhalation of ricin – A case report. Indian Journal of Forensic Medicine & Toxicology, 4, 36–37.
- Olsnes, S., & Kozlov, J. V. (2001). Ricin. Toxicon, 39, 1723–1728. 10.1016/S0041-0101(01)00158-1
- Olsnes, S. (2004). The history of ricin, abrin and related toxins. Toxicon, 44, 361–370. 10.1016/j.toxicon.2004.05.003
- Poli, M. A., Rivera, V. R., Hewetson, J. F., & Merrill, G. A. (1994). Detection of ricin by colorimetric and chemiluminescence ELISA. Toxicon, 32, 1371–1377. 10.1016/0041-0101(94)90409-X
- Rasooly, R., He, X., & Friedman, M. (2012). Milk inhibits the biological activity of ricin. Journal of Biological Chemistry, 287, 27924–27929. 10.1074/jbc.M112.362988
- Roberts, L. M., Lamb, F. I., Pappin, D. J., & Lord, J. M. (1985). The primary sequence of Ricinus communis agglutinin. Comparison with ricin. Journal of Biological Chemistry, 260, 15682–15686.
- Schieltz, D. M., McGrath, S. C., McWilliams, L. G., Rees, J., Bowen, M. D., Kools, J. J., Barr, J. R. (2011). Analysis of active ricin and castor bean proteins in a ricin preparation, castor bean extract, and surface swabs from a public health investigation. Forensic Science International, 209, 70–79. 10.1016/j.forsciint.2010.12.013
- Shyu, R. H., Shyu, H. F., Liu, H. W., & Tang, S. S. (2002). Colloidal gold-based immunochromatographic assay for detection of ricin. Toxicon, 40, 255–258. 10.1016/S0041-0101(01)00193-3
- Smith, P. K., Krohn, R. I., Hermanson, G. T., Mallia, A. K., Gartner, F. H., Provenzano, M. D., … Klenk, D. C. (1985). Measurement of protein using bicinchoninic acid. Analytical Biochemistry, 150, 76–85. 10.1016/0003-2697(85)90442-7
- Wein, L. M., & Liu, Y. (2005). Analyzing a bioterror attack on the food supply: The case of botulinum toxin in milk. Proceedings of the National Academy of Sciences of the United States of America, 102, 9984–9989. 10.1073/pnas.0408526102