Abstract
A direct competitive enzyme-linked immunosorbent assay (dc-ELISA) has been developed for the quantitative detection of the dicyclohexyl phthalate (DCHP) in some liquid food. Specific polyclonal antisera to DCHP was raised, using the hapten-bovine serum albumin conjugates as the immunogen. The conjugate of horseradish peroxidase (HRP) with antibody was used as the detectable probe to provide the direct measurement of the antigen and analyte, which was synthesised by a modified glutaraldehyde method. Under the optimised assay, the quantitative working range was from 0.1 to 100 ng mL−1 (R 2=0.9989), with a limit of detection (LOD) of 0.03 ng mL−1, and a recovery of 96.6–112.4%. The specificity and accuracy of developed method were evaluated. The cross-reactivities of antibody with structurally related phthalate esters were less than 10%. Results obtained indicated that the dc-ELISA was a sensitive, low expense method to improve the routine monitoring of trace constituents in some liquid food.
Introduction
Phthalates are industrial chemicals that are added to plastics to impart flexibility and resilience, which are often referred to as plasticisers, and also are used as solubilising or stabilising agents in other applications. Extensive use of these human-made chemicals resulted in their appearance in our daily lives, such as water (drinking water, surface water and waste water), toys, beverages, food packaging and plastic raincoats, lubricating oils, some medical devices, painting, vinyl tiles and flooring (Shen, Citation2005; Stringer et al., Citation2000; Yuan, Liu, Liao, & Chang, Citation2002). However, several manufactures capitalised on these advantages of phthalates to substitute for more expensive ingredients like palm oil, which were used as a filler to make items more opaque or richer in colour. For example, the occurrence of Taiwan's plasticiser food scandal captured the attention of the world in 2011. The Nation's Department of Health in Taiwan ordered that these contaminated food/beverages products were removed from store shelves, which laced with the plasticiser di(2-ethylhexyl) phthalate. Due to illegal use, adverse effects have shown that certain phthalate esters are potentially hazardous to the male reproductive system (McKee, Butala, David, & Gans, Citation2004; Staples, Parkerton, & Peterson, Citation2000). All evidence available to date strongly reveals that phthalates have to be recognised as priority pollutants and an important risk for target species (Kamrin, Citation2009). The European Commission (European Union, Citation1999), for example, is proposing a ban on the use of phthalate esters in childcare articles and toys. The Centers for Disease Control and Prevention (Fourth National Report, Citation2009) has shown that a number of phthalates appear in biomonitoring surveys of human, evidencing widespread human exposure. So there is this kind of two-facedness to the phthalate esters.
Dicyclohexyl phthalate (DCHP) belongs to the phthalates family. As mentioned earlier, it is mainly used to stabilise some rubbers, reins, barrier material, adhesive and other plastic film types but also a potential pollutant emitted from home and office environments (Destaillats, Maddalena, Singer, Hodgson, & McKone, Citation2008). Since they are not chemically but physically bound to the plastics to which they are added, phthalates can be migrated into the environment and food during use or disposal of the product, for example when plastic wrap placed over foods to be cooked in the microwave. So even if its acute toxicity of DCHP is not high, it has a strong function of enrichment in vivo. Otake, Yoshinag, and Yanagisawa (Citation2004) have detected phthalate esters in samples of indoor air from 27 houses in the Tokyo Metropolitan area; the median concentration of DCHP was 0.11 µg m−3. On the other hand, a small retail survey (47 samples) of confectionery, snack products and biscuits wrapped in printed polypropylene film showed the presence of one or more plasticisers at levels from 0.01 to 18.6 mg kg−1 for DCHP (Castle, Mayo, & Gilbert, Citation1989). Administration of some DCHP to feed of rats causes the reproductive toxicological effects on the parental animals including the endocrine system (Hoshino, Iwai, & Okazaki, Citation2005). On 1 June 2011, the Ministry of Health issued a public notice will be listed as phthalate plasticisers sixth installment of ‘food may be illegal and easy to add non-food substances abuse of food additives list’. According to the announcement, DCHP is listed as illegal to add ‘blacklist’. So to that extent, the availability of rapid and simple methods for monitoring of traces of these compounds prior to intake and characterising of food-borne hazards is of paramount importance in the environmental control and food security.
In recent years, numerous analytical techniques by way of high performance liquid chromatography (HPLC; Liang, Xu, & Li, Citation2008), gas chromatography coupled with mass spectrometry (GC-MS; Toda, Sako, Yagomea, & Nakamura, Citation2004) and even some more accurate methods including solid-phase microextraction (Feng, Zhu, & Sensenstein, Citation2005) and liquid-liquid microextraction (Yan, Cheng, & Liu, Citation2011) were generally reported. Although sensitive and reliable, they extremely require expensive and bulky equipment, large amounts of organic solvents for sample extraction and clean-up procedures, long run times. As an alternative, immunoassays, the measurement of antibody or antigen concentrations based on biospecific recognition interactions, have proven to be a major analytical method for identifying and quantitating the presence of the target analyte in various environmental matrices, such as food and medicine (Darwish, Al-Obaid, & Al-Malaq, Citation2010; El-Gendy, Aly, Mosallam, & Salama, Citation2011; Wang et al., Citation2009; Zhao, He, Pu, & Deng, Citation2008). The enzyme-linked immunosorbent assay (ELISA) is the technique most commonly used by molecular biologist for biomarker detection and quantification (Self & Cook, Citation1996). In terms of sensitivity, specificity, good selectivity and cost-effectiveness, several immunoassays for phthalate esters have been described by other authors (Kuang, Xu, Cui, Ma, & Xu, Citation2010; Zhang, Cong, Sheng, & Liu, Citation2010; Zhang, Liu, Cong, Liu, & Hu, Citation2011; Zhang, Wang, & Zhuang, Citation2006; Zhang et al., Citation2012). Of the three most commonly used ELISA enzymes (horseradish peroxidase [HRP], alkaline phosphatase and β-galactosidase), HRP is the most desired antibody or antigen label due to it being the smallest and most stable.
Therefore, based on the antigen competed with the free analyte to the primary labelled antibody based on the high specificity of antigen-antibody response, direct competitive ELISA (dc-ELISA) demonstrated that this proposed method development is suitable, sensitive and specific for trace analysis of DCHP in drinking water and milk samples.
Materials and methods
Chemicals
DCHP and o-phenylenediamine dihydrochloride (OPD) were supplied by Shanghai Chemical Reagent Co. (Shanghai, China). Bovine serum albumin (BSA) and chicken egg ovalbumin (OVA) were products of Sigma Chemicals (St Louis, Mo, USA). HRP was obtained from Sangon Biotechnology Technology Company Ltd (Shanghai, China). Twenty-five per cent of glutaraldehyde was purchased from Hefei BoMei Biotechnology Co. (Hefei, China). Freud's complete adjuvant (lanoline: mineral oil 1:2, with heat-killed mycobacterium tuberculosis) and Freud's incomplete adjuvant (lanoline: mineral oil 1:2) were prepared in our laboratory. All chemical reagents were of analytical grade and were used as received, unless specified otherwise. All aqueous solutions were prepared in doubly distillated water.
Apparatus
Absorbance measurement was performed on a Synergy HT Multi-Detection microtiter plate reader (Bio-Tek instruments, Inc., USA) at 490 nm. Polystyrene 96-well microplates were bought from Sangon Biotechnology Technology Company Ltd (Shanghai, China). Ultraviolet (UV) spectra were carried out on a UV-3010 spectrophotometer (Hitachi, Tokyo, Japan) with a plotter unit and 10-mm quartz cell. The pH values of all buffer solutions were measured with pHs-3C pH meter (Shanghai Precision & Scientific Instrument Co., Shanghai, China). Ultrapure Water polishing system (PSDK-10-E) was obtained from Son Han Century Technology Company Ltd (Beijing, China).
Buffers and solutions
DCHP standard solution was prepared in ethanol, before diluting with mixture in the proportion of PBS and ethanol.
Coating buffer (CB): 50 mM pH 9.6 carbonate buffer, contained 15 mM Na2CO3, 35 mM NaHCO3.
Assay buffer (PBS): 10 mM pH 7.4 phosphate-buffered saline, consisted of 137 mM NaCl, 2.7 mM KCl, 8.3 mM Na2HPO4, 1.9 mM NaH2PO4.
Washing solution (PBST): PBS containing 0.05% (v/v) Tween-20. Blocking buffer: 1% OVA added in PBS.
Substrate solution: 4 mg of OPD dissolved in 10 mL pH 5.0 phosphate-citrate buffer (sodium phosphate dibasic-citric acid monohydrate buffer solution), and freshly 15 µl 30% H2O2 was added immediately prior to use.
Stop solution: 2 mol L−1 sulphuric acid.
Synthesis of antigen
As is generally known, low molecular weight compounds such as the target DCHP (MW 330.4) are not immunogenic and do not elicit an immune response. Accordingly, it requires conjugation to carrier proteins to be immunogenic before the molecular structure containing an active functional group, such as hydroxyl, carboxyl or amino group. Dicyclohexyl 4-nitro phthalate (4-DCHNP) and dicyclohexyl 4-amino phthalate (4-DCHAP) as hapten derivative were synthesised in our laboratory. Immunogen as DCHAP-BSA conjugate was previously used (Zhang & Sheng, Citation2010). Similar reaction conditions as for the coated antigen DCHAP-OVA was covalently attached to OVA by the diazotisation method. Briefly, hapten DCHAP (0.0553 g) was dissolved in 0.1 mL concentrated hydrochloric acid and 3 mL H2O was added later. Then 4 mL 0.1 mol L−1 NaNO2 was added drop by drop. The mixture solution was stirred in the ice water bath for 30 minutes and a litter urea was added to remove the excess sodium nitrite. OVA solution (160 mg dissolved in 40 mL sodium borate, pH 9.2) was added dropwise and continuously stirred on ice bath for 3–4 hours. The colour of the reaction mixture changed from colourless to intense copper brown when the pH of the mixture was adjusted to 9.0. Finally, the obtained conjugate was dialysed against doubly distilled water (pH 7.0) that was changed with fresh water twice a day for five days at 4°C, and stored in the refrigerator last until use.
Generation of anti-DCHP antibody
Polyclonal antibodies were produced by DCHAP-BSA conjugation as immunogen according to the immunisation protocol described previously (Zhang & Sheng, Citation2010; Zhao et al., Citation2008). After immunisation, antisera titers were determined by non-competitive indirect ELISA using checkerboard titration (Cao, Lu, Long, Hong, & Sheng, Citation2005). When the final anti-sera exhibited strong positive response (1:64000), sufficient amount of sera were prepared for isolating by centrifugation, and then purified using saturated ammonium sulphate. Finally, the purified antibody was stored at 20°C and then used at appropriate dilution for the competitive ELISA.
Syntheses of HRP-antibody conjugate
HRP has been used for the detection purpose in this study because of its small size and high stability to the chemical modifications. The HRP label activity is applied as an enhancer toward the OPD chromogen that can be directly related to the analyte concentration and improved the sensitivity and detection limit of the dc-ELISA. In this paper, the anti-DCHP antibody labelled with HRP was achieved consulting the literature with a little modification (Zhang et al., Citation2010). Activation was performed by dissolving 10 mg of HRP in 0.4 ml of 0.05 M CB buffer and 0.1 ml of 25% glutaraldehyde. After incubation for 2 hours at 37°C, 2 ml of analytical reagent ice-cold ethanol (100%) was added and centrifuged. The precipitate was washed twice with ethyl alcohol (80%, v/v) and dissolved in 0.05 M sodium carbonate buffer (pH 9.6). Finally, the antibody solution (0.5 mL) was added into the mixture. After stored at 4°C overnight, the precursor solution was neutral by addition of a little dipotassium hydrogen phosphate. The content was purified, and then dialysed against PB (pH 7.2) overnight at 4°C. The obtained solution was kept at 4°C in refrigerator for further use.
Direct competitive ELISA procedure
A competitive direct ELISA format was adopted for analysing DCHP base on coating antigen and HRP-antibody conjugate. Antigen (DCHP-OVA diluted in CB: 7 µg mL−1, 100 µL per well) was coated onto the microtiter plates and incubated for 2 hours at 37°C. At each incubation, three washing steps were carried out with PBST per well to remove any excess antigen. Binding sites not occupied by the coating antigen were then blocked with blocking reagent (1% OVA in PBS, 150 µL per well) for 0.5 hour at 37°C. After washing in the same way, 50 µL per well of diluted DCHP standard or sample solutions was added to the wells, followed by the addition of 50 µL of HRP-antibody (1:125 dilution of the labelled antibody solution). The competitive immunological reaction was performed for 3 hours at 37°C, and the wells were again rinsed. About 100 µL of OPD peroxide-based substrate solution was added to each well, when allowed to react with HRP, and to proceed for 30 minutes at 37°C. Finally, the enzymatic reaction was stopped by addition of 50 µL 2 M sulphuric acid and the colour changed to orange. The absorbance was then read and recorded by a spectrophotometric ELISA reader at 490 nm. A standard curve was obtained by plotting the absorbance values against the logarithm of DCHP concentration, from that the concentrations of analyte in samples could be calculated run on the same plate.
Cross-reactivity
A group of structurally related phthalate esters were selected for testing cross-reactivity (CR) based on the values (A/A 0=50%) from the calibration curves. Preparation of standard solutions for other tested chemicals was similar to that for DCHP. Each cross-reactivity value was calculated using the following equation: CR=(IC50 of DCHP/ IC50 of other structurally relates substances)×100%. The IC50 values can be regarded as a measure of the affinity of a specific antibody for a given analyte (Wang et al., Citation2009).
Collection and pretreatment of samples
To evaluate the validity of the presented method, the optimised ELISA was applied to DCHP detection in several liquid food samples, such as fruit drink, milk, yoghourt, fruit and vegetable juice, which were purchased from the local retail supermarkets. Before spiked and recovery studies, food samples were fortified with the various appropriate concentrations of DCHP. For dc-ELISA determination, the sample pretreatment was carried out according to descried method with subtle changes (Wang, Chen, Gao, & Song, Citation2009; Zhang et al., Citation2012). Two milliliter aliquot of samples was extracted with 400 µL of ethanol (100%), after which the mixture was centrifuged twice at 8000 rpm for 15 minutes. The supernatant was then diluted 20-fold with the 0.01 M PBS (pH 7.4), directly used for the spiked experiment in triplicate, and the recovery was calculated based on the standard curve.
Results and discussion
Characterisation of coated antigen
Synthesis of coated antigen was obtained by diazotisation of an aromatic amine hapten and OVA. We have utilised the strong UV–Visible (UV–Vis) absorbance to calculate the approximate hapten/protein ratio on account of epitope densities for protein conjugates prepared by azo coupling (Szurdoki, Szekaces, Le, & Hammock, Citation2002). Thus, our estimation of antigen is based on molar extinction coefficient (ε) values of model azo compounds. UV–Vis spectra data () supported the structures of the final conjugate. In this study, the hapten density of hapten DCHAP-OVA was determined as 12.
Optimisation of assay conditions
In this study, to monitor the low amounts of DCHP and improve the performances of the selected immunoassay format, the effects of coating antigen and HRP-labelled anti-DCHP antibody concentration, incubation time, pH, concentration of PBS and blocking regent should be carefully optimised. The optimum conditions providing the best sensitivity were then chosen.
Optimum concentrations of antigen and HRP-antibody
We determined the optimal antigen concentration and antibody dilution in each method by the direct competitive protocol described above. The following checkerboard titration was carried out. Coated antigen (diluted 1–9 µg mL−1) was dispensed in the rows, and serial dilutions of peroxidase-labelled antibody (1:200, 1:250, 1:300, 1:350 and 1:400 in PBS buffer) were allowed to bind to each immobilised conjugated concentration. The corresponding concentration was considered to be favourable in the following test, when the measured absorbance values were plotted as a function of antigen concentrations at the different concentrations of HRP-antibody (). Thus, based on these data, the optimal coating antibody concentration was 7 µg mL−1, and the appropriate dilution of HRP-antibody conjugate was 1:250.
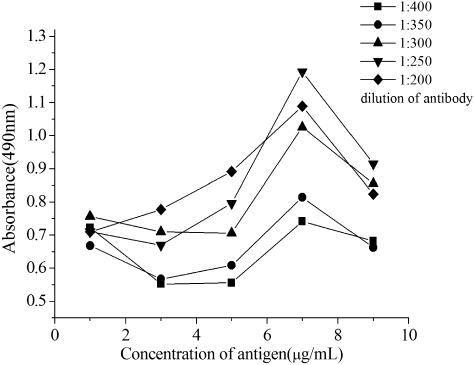
Effect of the blocking solution
The blocking solution was selected to decrease the background value. Briefly, competitive assay was performed using serial dilutions of OVA (from 3% to 0%, w/v) in . The addition of 1% OVA allowed for the successful suppression of the nonspecific binding between non-target analyte and antibody.
Incubation time
As the reaction of the antibody and the analyte is under a dynamic balance, the incubation time of competition reaction is an important influencing factor of the assay, as shown in . With the increase of incubation time, the formed immunocomplex increased, and reached a plateau at about 3 hours. Longer incubation time did not improve the response distinctly. Thus, taking time into account, 3 hour was chosen as the best time of antibody-antigen interaction for further studies.
Influence of pH of the buffer solution
Extreme pH values can be detrimental to a protein by causing irreversible denaturation. A narrower range of pH values may affect antibody binding to the corresponding antigen. This behaviour is similar to the immunoassays of phthalate esters previously reported (Zhang et al., Citation2006; Zhang et al., Citation2010). As illustrated in , the assay performed better under neutral and basic conditions than under slightly acidic ones. So a pH value around 7.4 was selected.
Influence of ionic strength
The binding of antigen and antibody is characterised by the weak intermolecular bonds such as van der Waals forces and hydrophobic interactions, which are affected by the changes in ionic strength. presents the effect of the phosphate ion concentration on ELISA characteristics at the competition step, which was evaluated from 5 to 50 mM. Increasing the concentration of PBS caused an initial improvement followed by a decline in assay sensitivity. In this study, the optimum concentration of the buffer, which provided the highest A max value, was 10 mM at pH 7.4, containing 0.05% Tween-20. And this result was actually agreed with our previous works (Zhang & Sheng, Citation2010).
Effect of organic solvent on the assay
Usually, immunoassays are developed for application with the aqueous phase. A problem can arise when the target analyte is hydrophobic, as for DCHP. In earlier experiments, alcohols were found to be assay-compatible solvents; the sensitivity of the ELISA was less affected when these solvents were present (Scharnweber, Fisher, Suchànek, Knopp, & Niessner, Citation2001). Ethanol is water miscible and commonly used for the extraction of analytes from various matrixes. In present dc-ELISA, it was found that the aqueous assay buffer was sufficient to 10% of ethanol without significant loss of assay sensitivity, because the large amount of organic solvent on present protocol cause the reduction of immunoreaction or enzyme reaction, which was explained by somewhat denaturation of antibody (Goda et al., Citation2005).
Calibration curve
In the development of ELISA, DCHP is quantified by its ability to inhibit the binding of HRP-labelled anti-DCHP antibody to the coating antigen in a competitive fashion. Accordingly, the typical standard curve of DCHP is shown in , under the optimal conditions chosen above. The linear range was 0.1–100 ng mL−1 with a correlation coefficient (r 2) 0.9989. The assay showed a good sensitivity with a limit of detection (LOD) of 0.03 ng mL−1, compared with 0.86 ng mL−1 for HPLC (Cai, Jiang, Liu, & Zhou, Citation2003). For the proposed assay, linear range is the low to high concentration range of standard curve. The LOD is calculated by the concentration corresponding to three times the standard deviation of the A 0, negative control, from its mean absorbance (Sherry & Clement, Citation1992). The dependence of these indices was demonstrated as a standard curve plotted using Origin 6.0 software. Thus, based on these data, this could indicate that the dc-ELISA method for determination of DCHP was sensitive, without complex sample clean-up steps.
Immunoassay specificity
The specificity of the ELISA to distinguish between DCHP and similar compounds is an important aspect that needs to be assessed by the CR of the anti-DCHP antibody with six structurally related phthalate esters; the results are listed in . As shown, the CRs of else related phthalate esters were all less than 10%, which is absolutely acceptable in the analysis. Interestingly, the anti-DCHP antibody exhibited high cross-reactivity with DCHNP due to the same aromatic structure, but DCHNP did not exist in the environment, only used as hapten for experiment. The interference of DCHNP in this assay would be eliminated. These results suggest that the antibody only reacts with dicyclonhexyl phthalate, and the developed method can easily differentiate DCHP from PAEs or other materials in the environmental samples.
Table 1. Cross-reactivity of DCHP structurally-related compounds.
Analysis of food samples
The reliability of the proposed assay was applied to the determination of DCHP in liquid food samples. Four food samples diluted with pure water were spiked at several concentrations of DCHP covering the optimised working range (0.5, 5, 10 ng mL−1). These diluting samples that diminish the matrices interference were directly analysed by use of the best ELISA (Zhao et al., Citation2008). Results of the analytical data, expressed as the percentage of recovery, are summarised in . The recoveries of DCHP obtain a good agree of the levels of immunoassay, varied from 96.6% to 112.4%. It is worth mentioning that the different matrixes in any of the sample tested did not affect DCHP concentration. The study of the analytical performance in spiked samples proved that the described immunoassay can be suitable for real sample analysis.
Table 2. Recovery of DCHP from spiking food samples measured by the optimised ELISA.
Conclusions
This purpose of the present work was to establish a simple and specific assay to monitor DCHP doping in food samples such as fruit and vegetable juice, fruit drink, milk and yoghourt. Under the optimised conditions, DCHP was detected by dc-ELISA, which has the concentration range of 0.1–100 ng mL−1 with a detection limit of 0.03 ng mL−1. In comparison with 0.05 µg mL−1 for the indirect competitive fluorescence immunoassay, the assay was therefore more sensitive although the linear range was not as wide, being 0.1–200 µg mL−1 (Zhang & Sheng, Citation2010). Meantime, the direct-coating ELISA format offers advantages over the indirect ELISA such as decreased time of analysis and conservation of primary antibody. In a word, this approach is a feasible screening method and successfully applied to practical monitoring in various fields. As we expected, the new rapid and effective analytical tool will be gradually marketed in future.
Acknowledgements
This work is supported by the National Natural Science Foundation of China (Nos. 21075002; 21175004), the Key Program of the Natural Science Foundation of the Anhui Higher Education Institutions of China (Grant No. KJ2011A141). We express our deep thanks for financial support. In addition, we thank Yali Sheng for the preparation of anti-DCHP antibody.
References
- Cai, Y. Q., Jiang, G. B., Liu, J. F., & Zhou, Q. X. (2003). Multi-walled carbon nanotubes packed cartridge for the solid-phase extraction of several phthalate eaters from water samples and their determination by high performance liquid chromatography. Analytica Chimica Acta, 494, 149–156. doi:10.1016/j.aca.2003.08.006
- Cao, Y. S., Lu, Y. T., Long, S. Y., Hong, J. B., & Sheng, G. Q. (2005). Development of an ELISA for the detection of bromoxynil in water. Environment International, 31, 33–42. doi:10.1016/j.envint.2004.06.002
- Castle, L., Mayo, A., & Gilbert, J. (1989). Migration of plasticizers from printing inks into foods. Food Additives and Contaminants, 6, 437–443. doi:10.1080/02652038909373802
- Darwish, I. A., Al-Obaid, A. M., & Al-Malaq, H. A. (2010). A highly sensitive and specific polyclonal antibody-based enzyme immunoassay for therapeutic monitoring and pharmacokinetic studies of atorvastatin. Microchimica Acta, 170, 67–74. doi:10.1007/s00604-010-0390-5
- Destaillats, H., Maddalena, R. L., Singer, B. C., Hodgson, A. T., & McKone, T. E. (2008). Indoor pollutants emitted by office equipment: A review of reported data and information needs. Atmospheric Environment, 42, 1371–1388. doi:10.1016/j.atmosenv.2007.10.080
- El-Gendy, K. S., Aly, N. M., Mosallam, E. M., & Salama, A. K. (2011). Preparation of antibodies and development of an enzyme immunoassay for determination of atrazine in environmental samples. Journal of Environmental Science and Health, Part B, 46, 321–327. doi:10.1080/03601234.2011.559885
- European Union. (1999). Press release: Ban of phthalates in childcare articles and toys. Retrieved from http://europa.eu/rapid/pressReleasesAction.do?reference=IP/99/829&format=HTML&aged=1&language=EN
- Feng, Y. L., Zhu, J. P., & Sensenstein, R. (2005). Development of a headspace solid-phase microextraction method combined with gas chromatography mass spectrometry for the determination phthalate esters in cow milk. Analytica Chimica Acta, 538, 41–48. doi:10.1016/j.aca.2005.02.020
- Fourth National Report on Human Exposure to Environmental Chemicals. (2009). Retrieved from: http://www.cdc.gov/exposurereport/
- Goda, Y., Hirobe, M., Kobayashi, A., Fujimoto, S., Ike, M., & Fujita, M. (2005). Production of a monoclonal antibody and development of enzyme-linked immunosorbent assay for alkyl ethoxylates. Analytica Chimica Acta, 528, 47–54. doi:10.1016/j.aca.2004.07.036
- Hoshino, N., Iwai, M., & Okazaki, Y. (2005). A two-generation reproductive toxicity study of dicyclohexyl phthalate in rats. The Journal of Toxicological Sciences, 30, S79–S96. doi:10.2131/jts.30.S79
- Kamrin, M. A. (2009). Phthalate risks, phthalate regulation, and public health: A review. Journal of Toxicology and Environmental Health, Part B, 12, 157–174. doi:10.1080/10937400902729226
- Kuang, H., Xu, L. G., Cui, G., Ma, W., & Xu, C. L. (2010). Development of determination of di-n-octyl phthalate (DOP) residue by an indirect enzyme-linked immunosorbent assay. Food and Agricultural Immunology, 21, 265–277. doi:10.1080/09540101003758962
- Liang, P., Xu, J., & Li, Q. (2008). Application of dispersive liquid–liquid microextraction and high-performance liquid chromatography for the determination of three phthalate esters in water samples. Analytica Chimica Acta, 609, 53–58. doi:10.1016/j.aca.2007.12.025
- McKee, R. H., Butala, J. H., David, R. M., & Gans, G. (2004). NTP center for the evaluation of risks to human reproduction reports on phthalates: addressing the data gaps. Reproductive Toxicology, 18, 1–22. doi:10.1016/j.reprotox.2003.09.002
- Otake, T., Yoshinag, J., & Yanagisawa, Y. (2004). Exposure to phthalate esters from indoor environment. Journal of Exposure Analysis and Environmental Epidemiology, 14, 524–528. doi:10.1038/sj.jea.7500352
- Scharnweber, T., Fisher, M., Suchànek, M., Knopp, D., & Niessner, R. (2001). Monoclonal antibody to polycyclic aromatic hydrocarbons based on a new benzo[a]pyrene immunogen. Fresenius. Journal of Analytical Chemistry, 371, 578–585. doi:10.1007/s002160101012
- Self, C. H., & Cook, D. B. (1996). Advances in immunoassay technology. Current Opinion in Biotechnology, 7, 60–65. doi:10.1016/S0958-1669(96)80096-6
- Shen, H. Y. (2005). Simultaneous screening and determination eight phthalates in plastic products for food used by sonication-assisted extraction/GC-MS methods. Talanta, 66, 734–739. doi:10.1016/j.talanta.2004.12.021
- Sherry, J. P., & Clement, R. E. (1992). Environmental chemistry: The immunoassay option. Critical Reviews in Analytical Chemistry, 23, 217–300. doi:10.1080/10408349208050856
- Staples, C. A., Parkerton, T. F., & Peterson, D. R. (2000). A risk assessment of selected phthalate esters in North American and Western European surface waters. Chemosphere, 40, 885–891. doi:10.1016/S0045-6535(99)00315-X
- Stringer, R., Labunska, I., Santillo, D., Johnston, P., Siddorn, J., & Stephenson, A. (2000). Concentrations of phthalate esters and identification of other additives in PVC children toys. Environmental Science and Pollution Research, 7, 27–36. doi:10.1065/espr199910.007 Retrieved from http://link.springer.com/article/10.1065%2Fespr199910.007?LI=true.
- Szurdoki, F., Szekacs, A., Le, H. M., & Hammock, B. D. (2002). Synthesis of haptens and protein conjugates foe the development of immunoassays for the insect growth regulator fenoxycarb. Journal of Agricultural and Food Chemistry, 50, 29–40. doi:10.1021/jf0107050
- Toda, H., Sako, K., Yagomea, Y., & Nakamura, T. (2004). Simultaneous determination of phosphate esters and phthalate esters in clean room air and indoor air by gas chromatography-mass spectrometry. Analytica Chimica Acta, 519, 213–218. doi:10.1016/j.aca.2004.06.018
- Wang, Z. M., Chen, D. H., Gao, X., & Song, Z. H. (2009). Subpicogram determination of melamine in milk products using a luminol-myoglobin chemiluminecence system. Journal of Agricultural and Food Chemistry, 57, 3464–3469. doi:10.1021/jf900132u
- Wang, Y. Z., Wei, D. P., Yang, H., Yang, Y., Xing, W.W., Li, Y., & Deng, A. P. (2009). Development of a highly sensitive and specific monoclonal antibody-based enzyme-linked immunosorbent assay (ELISA) for detection of Sudanlin food samples. Talanta, 77, 1783–1789. doi:10.1016/j.talanta.2008.10.016
- Yan, H. Y., Cheng, X. L., & Liu, B. M. (2011). Simultaneous determination of six phthalate esters in bottled milks using ultrasound-assisted dispersive liquid–liquid microextraction coupled with gas chromatography. Journal of Chromatography B, 879, 2507–2512. doi:10.1016/j.jchromb.2011.07.001
- Yuan, S. Y., Liu, C., Liao, C. S., & Chang, B. V. (2002). Effects of in utero through lactational exposure to dicyclohexyl phthalate and p, p′-DDE in Sprague-Dawley rats. Chemosphere, 49, 1295–1299. doi:10.1016/S0045-6535(02)00495-2
- Zhang, M. C., Cong, Y., Sheng, Y. L., & Liu, B. L. (2010). A direct competitive enzyme-linked immunosorbent assay by antibody coated for diethyl phthalate analysis. Analytical Biochemistry, 406, 24–28. doi:10.1016/j.ab.2010.06.040
- Zhang, M. C., Hu, Y. H., Liu, S. H., Cong, Y., Liu, B. L., & Wang, L. (2012). Rapid monitoring of dipropyl phthalate in food samples using a chemiluminescent enzyme immunoassay. Food Analytical Methods, 5, 1105–1113. doi:10.1007/s12161-011-9346-6
- Zhang, M. C., Liu, B. L., Cong, Y., Liu, S. H., & Hu, Y. H. (2011). Development of highly specific fluorescence immunoassay and enzyme-linked immunosorbent assay for detection of dimethyl phthalate in water samples. Food and Agricultural Immunology, 22, 297–309. doi:10.1080/09540105.2011.575124
- Zhang, M. C., & Sheng, Y. L. (2010). An indirect competitive fluorescence immunoassay for determination of dicyclohexyl phthalate in water samples. Journal of Fluorescence, 20, 1167–1173. doi:10.1007/s10895-010-0663-8
- Zhang, M. C., Wang, Q. E., & Zhuang, H. S. (2006). A novel competitive fluorescence immunoassay for the determination of dibutyl phthalate. Analytical and Bioanalytical Chemistry, 386, 1401–1406. doi:10.1007/s00216-006-0703-z
- Zhao, D., He, L., Pu, C., & Deng, A. P. (2008). A highly sensitive and specific polyclonal antibody-based enzyme-linked immunosorbent assay for detection of antibiotic olaquidox in animal feed samples. Analytical and Bioanalytical Chemistry, 391, 2653–2661. doi:10.1007/s00216-008-2179-5