ABSTRACT
Phenylbutazone, a non-steroidal anti-inflammatory drug commonly used to treat horses for musculoskeletal disorders, can cause adverse effects in humans and is therefore not permitted for use in food-producing animals in the EU. In recent years some equine and bovine samples have been found to be non-compliant. While physicochemical methods of analysis exist there is a need for less-expensive, rapid screening methods such as immunoassays. This study describes the production of antisera to detect phenylbutazone and its main metabolite, oxyphenylbutazone. Four haptens were chosen for immunogen preparation and two of these, suxibuzone and γ-hydroxyphenylbutazone, produced antisera with 50% of maximal inhibitory concentration (IC50s) of <5 ng mL−1 for phenylbutazone. Antisera with IC50s of 5.5 ng mL−1 for phenylbutazone and 5.6 ng mL−1 for oxyphenylbutazone were produced by their respective haptens. The figures suggest that the antisera could deliver detection capabilities below the concentration that is recommended by the Community Reference Laboratory (5 ng mL−1).
1. Introduction
Phenylbutazone (PBZ; ) is a non-steroidal anti-inflammatory drug (NSAID) that was introduced to human medicine in 1949 for the treatment of rheumatoid arthritis. Subsequent years of use led to the discovery that it could induce disorders of the blood including aplastic anaemia, leukopoenia, granulocytosis and thrombocytopenia, in some cases leading to death (Lees & Toutain, Citation2013a). Consequently, its use in human medicine became limited with the licence for use in man revoked in the UK in 1984 (Tobin et al., Citation1986).
From the 1950s it has been used extensively to provide horses with relief from pain particularly that associated with joint and muscle conditions (Lees & Toutain, Citation2013a). The Committee for Medicinal Products for Veterinary Use (CVMP) assessed PBZ in 1997 (European Medicines Agency, Citation1997) and found that the main health risks to the consumer were blood dyscrasias and the genotoxic/carcinogenic potential for which no thresholds could be identified and so no maximum residue limits could be established. Consequently, PBZ is not permitted for use in any animal destined for human consumption. The risks were reaffirmed in a joint statement by the European Food Safety Authority and the European Medicines Agency in Citation2013. In addition to the EU, PBZ is banned from use in food production animals in most other countries such as the USA, Canada and Japan. Suxibuzone (SBZ) is a derivative () of PBZ produced in the 1970s as a NSAID to cause less gastro-intestinal disturbances than PBZ in man (Mizushima, Shiokawa, Honma, & Kageyama, Citation1983) and horses (Monreal, Sabate, Segura, Mayos, & Homedes, Citation2004) but with the same efficacy. It is initially metabolised to PBZ in humans (Yasuda et al., Citation1982) and horses (Jaraiz, Rodriguez, San Andres, Gonzalez, & San Andres, Citation1999) and therefore is also unauthorised for use in food-producing animals. EU legislation requires that the treatment of any horses with the drugs must be documented in their passport so that they do not enter the food chain. However, food-producing horses are routinely monitored for the presence of PBZ and results published by the European Food Safety Authority (Citation2014) show an overall positive rate of 5.4% for the period from 2008 to 2012. The scandal that broke in 2013 regarding the fraudulent use of horsemeat as a substitute for beef (O'Mahony, Citation2013) led to the analysis of a number of food products for the banned drug. PBZ was subsequently found in beef-based products which had previously tested positive for equine DNA.
The use of the drug in food-producing animals is not limited to the equine species; bovine samples tested by EU member states from 2008 to 2012 produced a positive rate of 0.3% for the drug (European Food Safety Authority, Citation2014). Although a lower positive rate than for horses, this figure potentially equates to fivefold more bovine animals than equines containing detectable residues of the drug. The CVMP (1997) noted that PBZ is metabolised to oxyphenylbutazone (OPBZ) and γ-hydroxyphenylbutazone (HPBZ), probably representing the major metabolic pathways in most species. With this in mind detection of both PBZ and its metabolites may be beneficial for monitoring the illegal use of PBZ to provide versatility if various species and sample matrices are to be analysed.
A number of reports have been published describing mainly chromatographic methods for the detection of PBZ often in combination with OPBZ and/or other NSAIDs. A high performance liquid chromatography (HPLC) method was developed by Taylor and Westwood (Citation1995) to measure PBZ and OPBZ levels in the plasma of race horses; the method had a limit of quantitation (LOQ) of less than 1 μg mL−1. Another HPLC screening method with confirmation by gas chromatography-mass spectrometry (Neto, Andraus, & Salvadori, Citation1996) had a similar LOQ (1 μg ml−1) for plasma and urine and HPLC was also used to detect PBZ and OPBZ in serum (Haque & Stewart, Citation1997) with a LOQ of 0.5 μg mL−1. Liquid chromatography with UV detection was employed by De Veau (Citation1999) to quantify PBZ in bovine plasma at concentrations within the range of 20 ng to 2 μg mL−1 and PBZ was included in a qualitative multi-residue method to detect 6 NSAIDs in bovine muscle by liquid chromatography-tandem mass spectrometry (Van Hoof, De Wasch, Poelmans, Noppe, & De Brabander, Citation2004); the method could detect the analyte at 100 μg kg−1. More recent methods have continued the trend towards multi assays and have seen improvements in detection capability. LC-MS methods have been used to detect: PBZ and OPBZ in urine with a decision limit (CCα) of 2 ng mL−1 (Igualada & Moragues, Citation2005); OPBZ, PBZ and SBZ (plus five other NSAIDs) in bovine milk with CCαs of 2.12, 0.55 and 2.86 ng mL−1, respectively (Dowling, Gallo, Malone, & Regan, Citation2009); PBZ and OPBZ in muscle samples as two of nine NSAIDs with LOQs of 2.82 and 2.13 μg kg−1, respectively (Jedziniak, Szprengier-Juszkiewicz, Olejnik, & Zmudzki, Citation2010) and PBZ in bovine milk with a practical quantification limit (PQL) of 2.5 ng mL−1 in a method that also measured novobiocin residues (Thompson, Noot, & Kendall, Citation2010). As a less-expensive and more rapid alternative to chromatograpic methods, Meucci et al. (Citation2013) developed an electrochemical sensing technique for PBZ and flunixin in equine plasma samples with a LOQ of 10 ng mL−1 for PBZ. Immunochemical techniques such as enzyme linked immunosorbent assay (ELISA), which exploit the binding properties of specific antibodies, are another less costly alternative which can increase sample throughput substantially as screening methods prior to physicochemical confirmation. A report by Kwiatkowski et al. (Citation1989) describes the development of both a fluoroimmunoassay and ELISA to detect PBZ in the blood and urine of race horses. However, the publication and others referenced within it provide little experimental detail describing the hapten used or the conjugation procedure employed to prepare an immunogen. The ELISA proved to be the more sensitive of the two immunoassays with the half-maximal inhibition occurring at approximately 10 ng mL−1 for PBZ and 300 ng mL−1 for OPBZ. This sensitivity was more than adequate for the intended purpose of analysing race horse samples for violative levels of the drug (5 μg mL−1 in plasma). However, the antibody would not be able to detect the low levels required for monitoring drug residue levels in a food safety setting. The current study set out to produce antibodies capable of detecting both PBZ and OPBZ at concentrations below 5 ng mL−1, the detection concentration of the two target analytes as recommended by the Community Reference Laboratory (CRL Guidance Paper, Citation2007). PBZ, SBZ, OPBZ and HPBZ were employed as haptens and various chemical coupling techniques were used to prepare immunogens and corresponding enzyme labels for use in ELISAs. The antisera produced were assessed for sensitivity and specificity to determine their potential as suitable reagents in an immunochemical-based analytical method.
2. Materials and methods
2.1. Reagents and chemicals
Phenylbutazone (P8386), suxibuzone (S5521), N-(3-dimethylaminopropyl)-N-ethylcarbodiimide hydrochloride (EDC; E1769), N-hydroxysuccinimide (NHS; 130672), human serum albumin (HSA; A1887), 2-(N-morpholino) ethanesulphonic acid (MES; M3671), 4-aminobenzoic acid (ABA; A9878), sodium nitrite (237213), 1,1′ carbonyldiimidazole (CDI; 115533), N,N′-disuccininmidyl carbonate (DSC; 43720) and 4-(dimethylamino)pyridine (DMAP; 107700) were obtained from Sigma-Aldrich (Poole, Dorset, UK). Oxyphenylbutazone was obtained from LGC standards (Teddington, Middlesex, UK) and γ-hydroxyphenylbutazone (FH24086) was obtained from Carbosynth Ltd (Compton, Berkshire, UK). N-(p-maleimidophenyl)isocyanate (PMPI; 28100), N-succinimidyl-S-acetylthiopropionate (SATP; 26100), hydroxylamine hydrochloride (26103) and diaminoethanetetra acetic acid disodium salt (EDTA; D/0650/53) were purchased from Fisher Scientific UK (Loughborough, Leicestershire, UK); horseradish peroxidase (HRP; 10-814407-001) was purchased from Roche-Diagnostics Ltd. (Burgess Hill, West Sussex, UK); PD-10 desalting columns containing Sephadex G25 (17-0851-01) were obtained from GE Healthcare (Little Chalfont, UK) and Montanide ISA 50 V adjuvant was obtained from Seppic (Paris, France).
2.2. Preparation of hapten–protein conjugates
2.2.1. Preparation of PBZ–HSA immunogen
Sodium nitrite (24 mg) dissolved in 100 µL of water was added to ABA (40 mg), dissolved in 1 mL water with 100 µL concentrated hydrochloric acid (HCl), and allowed to react in darkness for 4 h at room temperature with gentle stirring. PBZ (90 mg) was dissolved in 250 µL of dimethylformamide (DMF) and added to the mixture; further aliquots of DMF were added to keep all the constituents in solution. The mixture was allowed to react overnight in darkness at room temperature and then freeze dried to remove the solvent. The residue was reconstituted in 1 mL of pyridine and 200 µL of this was added to EDC (10 mg) and NHS (5 mg), dissolved in 100 µL of MES buffer (0.05 M, 0.5 M sodium chloride, pH 5) and allowed to react at room temperature for 10 mins. The activated PBZ–ABA (200 µL) was then added to HSA (20 mg) dissolved in 1 mL phosphate buffered saline (PBS) (pH 7.2) and allowed to react for 3 h at room temperature with gentle mixing followed by dialysis against normal saline (0.15 M sodium chloride) to purify.
2.2.2. Preparation of SBZ–HSA immunogen
SBZ (24 mg) and CDI (52 mg) were dissolved in 12 mL of dry acetone and allowed to react in darkness for 4 h at room temperature with gentle stirring. The acetone was removed under a stream of nitrogen gas at 40°C. The residue was reconstituted in 600 µL of PBS (pH 7.2) and 200 µL of pyridine for complete solubility. The activated SBZ was added to HSA (20 mg) which was dissolved in 2 mL PBS (pH 7.2); a few drops of pyridine was added to keep all constituents in solution and the mixture was incubated for 72 h at 4°C with gentle stirring. The conjugate was then dialysed against normal saline to purify.
2.2.3. Preparation of OPBZ–HSA immunogen
SATP (4 mg) was dissolved in dimethyl sulphoxide (DMSO) and added to HSA (20 mg), dissolved in 2 ml PBS (pH 7.2), and allowed to react for 30 min at room temperature with gentle stirring. The modified protein was purified by gel filtration in a sephadex 25 desalting column against PBS (pH 7.2). Deacetylation solution (200 µL), comprising of 0.5 M hydroxylamine hydrochloride and 0.25 mM EDTA in PBS (pH 7.2), was added to the activated HSA mixture and allowed to react for 3 h at room temperature with gentle stirring before purification by gel filtration against PBS (pH 7.2). OPBZ (4 mg) and PMPI (7 mg) were dissolved in DMSO and allowed to react for 3 h at room temperature before adding to the modified HSA. The mixture was incubated overnight at 4°C with gentle mixing and then purified by dialysis against normal saline.
2.2.4. Preparation of HPBZ–HSA immunogen
HPBZ (2.5 mg), DSC (12 mg) and DMAP (5.5 mg) were each dissolved in 1 ml of dry acetone and combined. The mixture was allowed to react for 4 h at room temperature with gentle mixing before removing the solvent at 40°C under a stream of nitrogen gas. The residue was reconstituted in 200 µL of PBS (pH 7.2) and 200 µL pyridine and then added to HSA (20 mg), dissolved in PBS (pH 7.2). The mixture was incubated overnight at 4°C with gentle mixing before purification by gel filtration against normal saline.
2.2.5. Preparation of hapten–enzyme labels
Corresponding enzyme conjugates were prepared for each of the haptens by coupling with HRP using the same technique as described for the respective immunogens. The enzyme conjugates were employed in direct competitive ELISAs to assess the antisera for specific antibodies and to determine their relative sensitivity.
2.3. Immunisation and blood sampling protocol for antiserum production
The prepared immunogen (0.5 mg protein in 0.5 mL of saline) was mixed with 0.5 mL of Montanide ISA 50v adjuvant to create an emulsion. Two New Zealand White rabbits were immunised every 4 weeks with the immunogenic emulsion at four injection sites. Blood samples were collected 10 days after each immunization and assessed for specific antibody titre. When a satisfactory titre was obtained, the antibodies were harvested from the rabbits. All procedures were performed in accordance with the Animals (Scientific Procedures) Act 1986, UK.
2.4. Assessment of specific antibody response
During the immunisation schedule, antisera samples were assessed for the presence of antibodies specific to the hapten that was used to prepare the relevant immunogen. This was achieved by performing ELISA checkerboards in a competitive assay format with each of the four enzyme conjugates to compare the effectiveness of homologous and heterologous assays. Microtitre plates (Falcon 353070) were coated (100 μL/well) with serial dilutions of rabbit serum in 1 mM sodium acetate solution, pH 6.2, and incubated overnight at room temperature. Analyte standard (50 μL) diluted to 200 ng mL−1 in 1 mM sodium acetate solution, pH 6.2 and horseradish peroxidase conjugate (50 μL) serially diluted in blocking buffer (2 mg mL−1, w/v bovine serum albumin in 1 mM sodium acetate solution, pH 6.2) were added to positive control wells. Negative control wells containing all components except the analyte were also included. The plate was then incubated overnight at 4 °C. After 12 washes with wash solution (0.9% sodium chloride, 0.1% Tween 20), antibody bound peroxidase was measured using 3,3′,5,5′-tetramethylbenzidine (ELISA) (TMB/E; Chemicon International ES001), a colorimetric substrate. Absorbance was read at 450 nm on a Tecan Sunrise plate reader after 12 min colour development.
2.5. Determination of assay sensitivity and specificity in buffer by ELISA
Antisera that possessed hapten-specific antibodies were assessed further for sensitivity and specificity towards PBZ, OPBZ, SBZ and HPBZ. The assessment was achieved using the enzyme conjugate that performed best with each respective antisera while determining the antibody response. The ELISA described above was performed using the optimum antiserum and enzyme label dilutions as predetermined by the checkerboard titration results. Sets of standards were prepared in 1 mM sodium acetate solution, pH 6.2 (0, 1, 2.5, 5, 10, 25, 50 and 100 ng mL−1) for PBZ, OPBZ, SBZ and HPBZ. Each standard was added (50 μL/well) in triplicate to the microtitre plates previously coated with antisera. The appropriate enzyme label was also added (50 μL/well) to all wells. Plates were incubated overnight at 4°C before washing and developing using enzyme substrate. The average optical density (OD) of the zero standard wells (0 STD), containing all components except the competitor, was taken to represent 100% binding and the remaining standards were normalised relative to the optical density of the zero standard. The 50% of maximal inhibitory concentration (IC50; half-maximal inhibitory concentration) was calculated as the concentration of unlabelled analyte that caused a 50% reduction in binding of the enzyme labelled form of the analyte and was used as a comparative indicator of sensitivity. IC50 values were determined for each of the standard sets as an indicator of sensitivity. Cross-reactivity values were determined as a ratio of the IC50 of the relevant hapten with the IC50 of the other competitors and given as a percentage.
3. Results and discussion
There is a need to produce antibodies, capable of binding PBZ, to employ in immunochemical methods of detection for the veterinary drug. Depending on which sample matrix is chosen as the target for analysis, there may also be a need to produce antibodies that can bind the main metabolites of PBZ; detection of SBZ is not necessary as it is a prodrug of PBZ. PBZ, its two metabolites (OPBZ and HPBZ) and SBZ are all commercially available and so these compounds, shown in , were obtained to be used as haptens for antibody production. The carboxylic acid group of SBZ and the hydroxyl groups of OPBZ and HPBZ were targeted as functional groups that could be reacted with primary amines on a carrier protein via a range of commonly used conjugation techniques. While PBZ lacks such a functional group, a technique described by Kawashima, Dixon, and Spector (Citation1975) was employed to conjugate p-aminobenzoic acid to the drug prior to coupling to the carrier protein via the introduced carboxylic acid group. The reaction schemes used to couple PBZ, SBZ, OPBZ and HPBZ to the HSA carrier protein are shown in –, respectively. The position of conjugation is similar for PBZ and OPBZ at one of the benzene rings while for SBZ and HPBZ conjugation occurs at the opposite end of the molecule. It could be anticipated that these two hapten pairs may produce antibodies with similar cross-reactivity profiles. However, while HPBZ provides a functional hydroxyl group on the butyl chain for reaction, the hemisuccinate chain on SBZ for conjugation leaves the entire molecular structure of PBZ free as an epitope for antibody production.
Figure 2. Diazotised amino benzoic acid is reacted with PBZ to provide a carboxylic acid which can be activated to react with primary amines on the carrier protein.
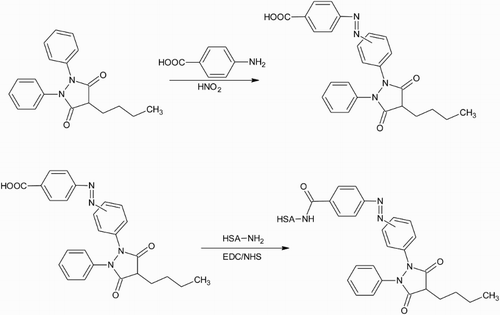
Figure 3. The carboxylic acid on suxibuzone is activated with one end of the bifunctional cross-linker CDI. The other end is reacted with primary amines on the carrier protein to generate a stable amide bond.
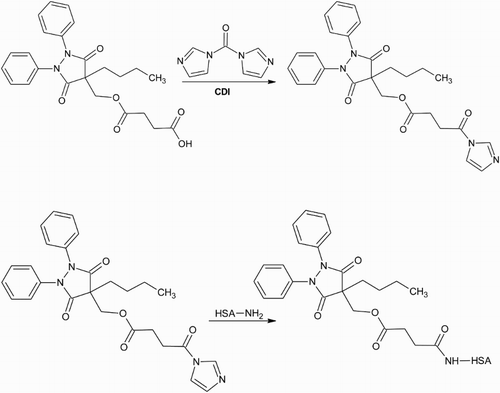
Figure 4. OPBZ is activated by reacting the hydroxyl group with the isocyanate group of the cross-linker PMPI. The carrier protein is modified with SATP to introduce sulphhydryl groups that are then reacted with the maleimide group on the other end of PMPI to create a stable thioether coupling.
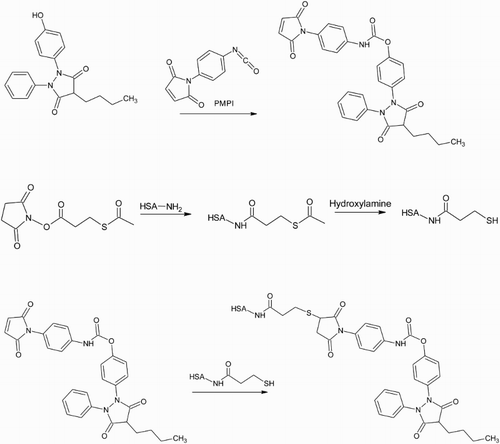
Figure 5. The hydroxyl group on HPBZ is activated using one end of the bifunctional cross-linker DSC. The other end reacts with primary amines on the carrier protein to produce a carbamate linkage between hapten and protein.
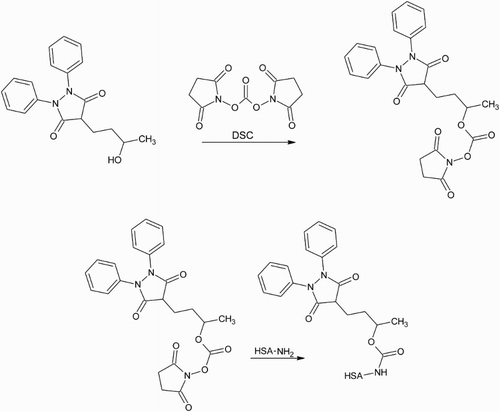
The haptens were evaluated for their efficacy at producing the desired antibodies by assessing how the resultant antisera performed with respect to sensitivity and specificity towards the target compounds. When antisera from each rabbit was being assessed for the presence of specific antibodies each of the four respective hapten–enzyme labels was used in conjunction with each antisera. For all antisera the combination with the SBZ–HRP proved to provide the optimum response and so only this enzyme label was subsequently employed for the determination of sensitivity and specificity. As has been shown before (Fodey et al., Citation2013) it is common for heterologous assays to be more sensitive than the corresponding homologous ones. In this study, this is the case for three groups of antisera with only antisera produced by the SBZ hapten performing better in a homologous format. These results indicate that the reaction to prepare the SBZ–HRP is more efficient than the reactions employed for the other three haptens. The reactivity of hapten is more important for preparation of enzyme labels than it is for immunogen preparation as there are fewer primary amines available for reaction on HRP than on HSA (or other carrier proteins).
displays the IC50 and % cross-reactivity (CR) for each antiserum in combination with the SBZ–HRP enzyme label. One rabbit, immunised with OPBZ–HSA did not produce an antiserum that could specifically bind any of the four haptens at concentrations lower than 100 ng mL−1. However, the remaining seven rabbits did produce antisera that could specifically bind PBZ at concentrations of 18.3 ng mL−1 or less; the two SBZ and one of the HPBZ rabbits produced the three most sensitive antisera to PBZ with IC50s ranging from 0.9 to 4.6 ng mL−1. Although SBZ is a prodrug of PBZ, and so its detection is not required, four antisera with IC50s of less than 6 ng mL−1 were produced by one rabbit from each pair immunised with each of the haptens. The IC50s for OPBZ were >50 ng mL−1 for six of the antisera but one of the rabbits, immunised with the OPBZ hapten, produced an antiserum that could be capable of detecting the metabolite at the required concentration, displaying an IC50 of 5.6 ng mL−1, another rabbit immunised with the HPBZ produced antisera with an IC50 of 9.3 ng mL−1 for OPBZ. In a similar way IC50s for the metabolite HPBZ were greater than 100 ng mL−1 for all haptens except HPBZ itself; the two rabbits immunised with this hapten delivered IC50s of 3.9 and 12.7 ng mL−1.
Table 1. The sensitivity (IC50) and specificity (CR) of each antiserum in combination with SBZ–HRP in an ELISA.
Analytical methods employed to detect veterinary drug residues for substances that are licensed and have been assigned a maximum residue level (MRL) are required to have limits of detection at or below the MRL. For substances that are not licensed for use in food-producing animals, the methods of analysis are required to have limits of detection that are as low as possible. In some instances a minimum required performance limit (MRPL) may be assigned for techniques or as in the case for PBZ the Community Reference Laboratory has recommended a concentration for detection of 5 ppb (ng mL−1) in muscle, milk, kidney, liver and plasma for PBZ and OPBZ (CRL Guidance Paper, Citation2007); there is no such figure recommended for HPBZ.
Pharmacokinetic studies have shown that the elimination half-life of PBZ is approximately 10 times greater in cattle than in horses, which is explained by a relatively reduced metabolism of the drug (Lees, Ayliffe, Maitho, & Taylor, Citation1988). Consequently PBZ predominates in bovine plasma, with less than 1% of the total drug present as OPBZ or HPBZ (De Veau, Pedersoli, Cullison, & Baker, Citation1998). In contrast, in equine plasma OPZ concentrations are approximately 20% of PBZ concentrations while HPBZ levels are very low (Lees & Toutain, Citation2013b). Lees et al. (Citation1988) also found only trace amounts of the metabolites in bovine plasma but much higher concentrations were found in the urine (up to 301 times the plasma concentration). While it has been reported that PBZ concentrations are significantly less in tissue than plasma and over time decline in parallel with plasma (Arifah & Lees, Citation2002), information regarding tissue concentrations of OPBZ is scant. The pharmacokinetic studies above determined the depletion of PBZ from cattle by measuring levels in plasma using liquid chromatographic methods capable of detecting concentrations down to 1 μg mL−1 (De Veau et al., Citation1998), 50 ng mL−1 (Arifah & Lees, Citation2002) or 10 ng mL−1 (Lees et al., Citation1988). While adequate for pharmacokinetic studies these levels of detection are not sufficient for a food safety regulatory laboratory which is required to detect the unauthorised substance at levels below 5 ng mL−1 (CRL Guidance Paper, Citation2007). A concentration of 400 ng mL−1 for OPBZ in bovine plasma may be considered low with respect to biological activity (Arifah & Lees, Citation2002) but it is a substantial amount as a residue indicating the use of an unauthorised substance. Further pharmacokinetic studies need to be performed to establish the levels of the metabolites that can actually be detected in bovine and equine tissue. It would be beneficial to know, for example, if tissue could provide a more sensitive target matrix than blood for analysing samples from animals that may have been withdrawn from PBZ for some time before slaughter and therefore the majority of the drug could have been metabolised.
Sensitivity is an important issue when developing any method for the detection of a drug which is not licensed for use in food-producing animals. For an immunochemical technique sensitivity is primarily provided by the antibody employed in the assay. Although sample matrix effects can have a detrimental effect on sensitivity a limit of detection in matrix will often be lower than an IC50 obtained in buffer. With this in mind it would be expected that the antisera produced by the SBZ and HPBZ haptens with IC50s of 3.6 and 0.9 ng mL−1 for PBZ could provide adequate sensitivity for a detection method for PBZ in compliance with the CRL Guidance Paper (Citation2007) and in the same way antisera from one of the OPBZ rabbits would be sufficiently sensitive to employ in a method for detection of OPBZ. An ELISA developed by Kwiatkowski et al. (Citation1989) provided an IC50 of approximately 10 ng mL−1 for PBZ and 300 ng mL−1 for OPBZ. While adequately sensitive for the intended purpose of analysing race horse plasma samples for violative levels of the drug it would be insufficient for monitoring drug residue levels in a food safety setting. As far as we are aware this is the first report describing the production of antisera for PBZ and OPBZ that are capable of detecting the drugs in food samples of animal origin.
Disclosure statement
No potential conflict of interest was reported by the authors.
References
- Arifah, A. K., & Lees, P. (2002). Pharmacodynamics and pharmacokinetics of phenylbutazone in calves. Journal of Veterinary Pharmacology and Therapeutics, 25, 299–309. doi: 10.1046/j.1365-2885.2002.00421.x
- CRL Guidance paper. (2007). CRLs view on state of the art analytical methods for national residue control plans. Retrieved from: http://www.bvl.bund.de/SharedDocs/Downloads/09_Untersuchungen/EURL_Empfehlungen_Konzentrationsauswahl_Methodenvalierungen.pdf__blob=publicationFile.
- De Veau, E. J. I. (1999). Determination of non-protein bound phenylbutazone in bovine plasma using ultrafiltration and liquid chromatography with ultraviolet detection. Journal of Chromatography B: Biomedical Sciences and Applications, 721, 141–145. doi: 10.1016/S0378-4347(98)00487-3
- De Veau, E. J. I., Pedersoli, W., Cullison, R., & Baker, J. (1998). Pharmacokinetics of phenylbutazone in plasma and milk of lactating dairy cows. Journal of Veterinary Pharmacology and Therapeutics, 21, 437–443. doi: 10.1046/j.1365-2885.1998.00167.x
- Dowling, G., Gallo, P., Malone, E., & Regan, L. (2009). Rapid confirmatory analysis of non-steroidal anti-inflammatory drugs in bovine milk by rapid resolution liquid chromatography tandem mass spectrometry. Journal of Chromatography A, 1216, 8117–8131. doi: 10.1016/j.chroma.2009.05.004
- European Food Safety Authority. (2014). Report for 2012 on the results from the monitoring of veterinary medicinal product residues and other substances in live animals and animal products. EFSA supporting publication 2014: EN-540.
- European Food Safety Authority and European Medicines Agency. (2013). Joint statement of EFSA and EMA on the presence of residues of phenylbutazone in horse meat. EFSA Journal, 11, Article no. 3190, 1–45.
- European Medicines Agency. (1997). Committee for Medicinal Products for Veterinary Use: Phenylbutazone. Summary Report, EMEA/MRL/297/97-FINAL October 1997.
- Fodey, T. L., George, S. E., Traynor, I. M., Delahaut, P., Kennedy, D. G., Elliott, C. T., & Crooks, S. R. H. (2013). Approaches for the simultaneous detection of thiamphenicol, florfenicol and florfenicol amine using immunochemical techniques. Journal of Immunological Methods, 393, 30–37. doi: 10.1016/j.jim.2013.04.003
- Haque, A., & Stewart, J. T. (1997). Direct injection HPLC method for the determination of phenylbutazone and oxyphenylbutazone in serum using a semipermeable surface column. Journal of Pharmaceutical and Biomedical Analysis, 16, 287–293. doi: 10.1016/S0731-7085(97)00041-1
- Igualada, C., & Moragues, F. (2005). Determination of phenylbutazone and oxyphenbutazone in animal urine by ion trap liquid chromatography–mass spectrometry. Analytica Chimica Acta, 529, 235–238. doi: 10.1016/j.aca.2004.10.034
- Jaraiz, M. V., Rodriguez, C., San Andres, M. D., Gonzalez, F., & San Andres, M. I. (1999). Disposition and tolerance of suxibuzone in horses. Equine Veterinary Journal, 31, 411–416. doi: 10.1111/j.2042-3306.1999.tb03841.x
- Jedziniak, P., Szprengier-Juszkiewicz, T., Olejnik, M., & Zmudzki, J. (2010). Determination of non-steroidal anti-inflammatory drugs residues in animal muscles by liquid chromatography–tandem mass spectrometry. Analytica Chimica Acta, 672, 85–92. doi: 10.1016/j.aca.2010.04.031
- Kawashima, K., Dixon, R., & Spector, S. (1975). Development of radioimmunoassay for chlorpromazine. European Journal of Pharmacology, 32, 195–202. doi: 10.1016/0014-2999(75)90283-6
- Kwiatkowski, S., Goodman, J.P., Stanley, S.D., Tai, C.L., Yang, J.-M., Wood, T., … Tobin, T. (1989). Immunoassay detection of drugs in racing horses X. Detection of phenylbutazone in equine blood and urine by particle concentration fluoroimmunoassay and ELISA. Research Communications in Substances of Abuse, 10, 123–139.
- Lees, P., Ayliffe, T., Maitho, T. E., & Taylor, J. B. O. (1988). Pharmacokinetics, metabolism and excretion of phenylbutazone in cattle following intravenous, intramuscular and oral-administration. Research in Veterinary Science, 44, 57–67.
- Lees, P., & Toutain, P. L. (2013a). Pharmacokinetics, pharmacodynamics, metabolism, toxicology and residues of phenylbutazone in humans and horses. Veterinary Journal, 196, 294–303. doi: 10.1016/j.tvjl.2013.04.019
- Lees, P., & Toutain, P. L. (2013b). Phenylbutazone in horses and man: Properties relevant to safety of humans consuming horse meat containing phenylbutazone and its metabolites. Equine Veterinary Education, 25, 545–549. doi: 10.1111/eve.12112
- Meucci, V., Vanni, M., Sgorbini, M., Odore, R., Minunni, M., & Intorre, L. (2013). Determination of phenylbutazone and flunixin meglumine in equine plasma by electrochemical-based sensing coupled to selective extraction with molecularly imprinted polymers. Sensors and Actuators B: Chemical, 179, 226–231. doi: 10.1016/j.snb.2012.09.015
- Mizushima, Y., Shiokawa, Y., Honma, M., & Kageyama, T. (1983). A double-blind comparison of phenylbutazone and suxibuzone, a prodrug of phenylbutazone, in rheumatoid-arthritis. International Journal of Tissue Reactions – Experimental and Clinical Aspects, 5, 35–39.
- Monreal, L., Sabate, D., Segura, D., Mayos, I., & Homedes, J. (2004). Lower gastric ulcerogenic effect of suxibuzone compared to phenylbutazone when administered orally to horses. Research in Veterinary Science, 76, 145–149. doi: 10.1016/j.rvsc.2003.10.004
- Neto, L. M. R., Andraus, M. H., & Salvadori, M. C. (1996). Determination of phenylbutazone and oxyphenbutazone in plasma and urine samples of horses by high-performance liquid chromatography and gas chromatography–mass spectrometry. Journal of Chromatography B: Biomedical Sciences and Applications, 678, 211–218. doi: 10.1016/0378-4347(95)00508-0
- O'Mahony, P. J. (2013). Finding horse meat in beef products – A global problem. Qjm – An International Journal of Medicine, 106, 595–597. doi: 10.1093/qjmed/hct087
- Taylor, M. R., & Westwood, S. A. (1995). Quantitation of phenylbutazone and oxyphenbutazone in equine plasma by high-performance liquid-chromatography with solid-phase extraction. Journal of Chromatography A, 697, 389–396. doi: 10.1016/0021-9673(94)00834-V
- Thompson, T. S., Noot, D. K., & Kendall, J. D. (2010). Surveillance study of novobiocin and phenylbutazone residues in raw bovine milk using liquid chromatography–tandem mass spectrometry. Food Additives and Contaminants Part a – Chemistry Analysis Control Exposure & Risk Assessment, 27, 1104–1111.
- Tobin, T., Chay, S., Kamerling, S., Woods, W. E., Weckman, T. J., Blake, J. W., & Lees, P. (1986). Phenylbutazone in the horse – A review. Journal of Veterinary Pharmacology and Therapeutics, 9, 1–25. doi: 10.1111/j.1365-2885.1986.tb00008.x
- Van Hoof, N., De Wasch, K., Poelmans, S., Noppe, H., & De Brabander, H. (2004). Multi-residue liquid chromatography/tandem mass spectrometry method for the detection of non-steroidal anti-inflammatory drugs in bovine muscle: Optimisation of ion trap parameters. Rapid Communications in Mass Spectrometry, 18, 2823–2829. doi: 10.1002/rcm.1683
- Yasuda, Y., Shindo, T., Mitani, N., Ishida, N., Oono, F., & Kageyama, T. (1982). Comparison of the absorption, excretion, and metabolism of suxibuzone and phenylbutazone in humans. Journal of Pharmaceutical Sciences, 71, 565–572. doi: 10.1002/jps.2600710521