ABSTRACT
A sensitive and selective indirect competitive enzyme-linked immunosorbent assay (ic-ELISA) and an immunochromatographic test strip were developed for the detection of tetrabromobisphenol A (TBBPA), an important brominated flame retardant. A monoclonal antibody (mAb) against TBBPA was produced and the half inhibition concentration (IC50) was 0.44 ng/ml, with the linear range 0.15–1.32 ng/ml. TBBPA recovery by ic-ELISA was 70.7–108.8% from rice pudding samples and 110.2–119.8% from lake water samples. A sensitive and rapid immunochromatographic test strip was developed using this specific mAb, with a good cut-off value of 25 and 50 ng/ml in lake water and rice pudding samples, respectively. The results of this study revealed that the ic-ELISA and immunochromatographic test strip represent useful methods of TBBPA detection in lake water and rice pudding samples.
Introduction
Tetrabromobisphenol A (TBBPA, 4,4′-(propane-2, 2-diyl) bis (2,6-dibromophenol)) is the most widely used brominated flame retardant. As an additive flame retardant, TBBPA is mainly used to produce high-impact polystyrene and acrylonitrile–butadiene–styrene resins (Bu, Zhuang, Zhou, & Yang, Citation2014; Covaci et al., Citation2009). And as a reactive flame retardant, it is commonly used in printed circuit board epoxys and polycarbonate resins where it contributes to the fire safety of electrical and electronic equipment. Therefore, TBBPA is present in several consumer goods, such as electronics, furniture, cars, computers, and construction materials (Ezechias, Covino, & Cajthaml, Citation2014; Stubbings & Harrad, Citation2014). However, TBBPA residues are harmful to the environment. It has been reported that TBBPA is present in soil, river, air, sea, wildlife, and even humans (Germer et al., Citation2006; Kefeni, Okonkwo, Olukunle, & Botha, Citation2011). Studies have shown that TBBPA contributes to endocrine disorders, immune dysfunction, and neurotoxicity (Tada et al., Citation2006). The Stockholm Convention on Persistent Organic Pollutants has established guidelines on most brominated flame retardants. In April 2015, TBBPA was included in the Community Rolling Action Plan, while controls for its remaining are under discussion. Several studies have analyzed TBBPA levels in the environment including waste electrical, lake water, soil, sediments, and some aquatic organisms (Bu et al., Citation2014; Feng et al., Citation2012; He, Luo, & Yu, Citation2010; Kajiwara et al., Citation2014; Kuiper et al., Citation2007; Puype, Samsonek, Knoop, Egelkraut-Holtus, & Ortlieb, Citation2015; Svihlikova et al., Citation2015; Xu et al., Citation2012). However, few studies have analyzed TBBPA levels in foods, such as breast milk, liquid foods, and their packaging materials (Driffield et al., Citation2008; Lyche, Rosseland, Berge, & Polder, Citation2015; Nakao et al., Citation2015; Pratt et al., Citation2013).
In recent years, TBBPA has been detected using more and more different methods, especially instrumental analytical methods, including liquid chromatography, liquid chromatography–mass spectrometry (Ezechias et al., Citation2014; Ten Dam, Pardo, Traag, van der Lee, & Peters, Citation2012), liquid chromatography-tandem mass spectrometry (LC-MS/MS) (Driffield et al., Citation2008; Geens, Roosens, Neels, & Covaci, Citation2009), gas chromatography–mass spectrometry (Samsonek & Puype, Citation2013; Sanchez-Brunete, Miguel, & Tadeo, Citation2009), ultra-performance liquid chromatography–mass spectrometry (Shi et al., Citation2013), polymerase chain reaction (Ma, Crump, Farmahin, & Kennedy, Citation2015), and enzyme-linked immunosorbent assay (ELISA) (Bu et al., Citation2014; Xu et al., Citation2012, Citation2013). With the exception of ELISA, these analytical methods are accurate; however, they require expensive equipment, skilled personnel, and time-consuming sample preparation. Additionally, these methods are not suitable for TBBPA detection on site.
In this study, we produced a specific and highly sensitive monoclonal antibody (mAb) against TBBPA. Based on the mAb, a sensitive indirect competitive enzyme-linked immunosorbent assay (ic-ELISA) was developed to detect TBBPA residues in lake water and rice pudding samples. In addition, an immunochromatographic test strip was developed for the rapid on site detection of TBBPA.
Experimental methods
Chemicals and materials
All reagents used in this study were analytical grade (AR) unless indicated otherwise. High-purity TBBPA (≥99%) was purchased from Aladdin Chemistry Co. Ltd. (Beijing, China). Bovine serum albumin (BSA; MW = 67,000) and ovalbumin (OVA; MW = 45,000) were used as carrier proteins, and 4,4-bis (3,5-dibromo-4-hydroxyphenyl) valeric acid, 1-(3-dimethylaminopropyl)-3-ethyl carbodiimide (EDC) and N-hydroxysuccinimide (NHS) were used in antigen synthesis. Complete Freund's adjuvant (FCA) and incomplete adjuvant (FIA) were used to immunize mice. All of the above chemicals were obtained from Sigma Chemical Co. Ltd. (St. Louis, MO, USA). Horseradish peroxidase (HRP)-labeled goat anti-mouse immunoglobulin was acquired from Kangcheng Bioengineering Co. (Shanghai, China). Reagents for cell fusion such as fetal calf serum, RPMI-1640 cell culture medium, hypoxanthine–aminopterin–thymidine medium, and hypoxanthine–thymidine medium were obtained from Gibco BRL (Paisley, Scotland). Nitrocellulose membranes were purchased from Pierce; adhesive tape and semi-rigid polyethylene sheets were obtained from a local market. Other materials for the immunochromatographic test strip were obtained from JieYi Biotech. Co. Ltd. (Shanghai, China).
Buffers and solutions
The buffers and solutions used in this study were the following, phosphate-buffered saline (PBS, 0.01 M, pH 7.4); 0.05% Tween-20 (m/v) in PBS, used as washing buffer coating solution (2.93 g NaHCO3 and 1.59 g Na2CO3 in 1 l Milli Q-water (CB, pH 9.6); 0.2% gelatin (m/v) in CB, which was applied as the blocking buffer; solution A (9.33 g citric acid, 36.8 g Na2HPO4, and 180 µl of 30% H2O2 in 1 l Milli Q-water), and solution B (0.3 g tetramethylbenzidine (TMB) in 500 ml glycol). The ELISA substrate solution for the HRP was composed of solution A and solution B, mixing A and B at a ratio of 5:1 (v/v) unless use. The stop solution was 2 mol/l sulfuric acid.
Immunogen and coating antigen synthesis
There are no functional groups in TBBPA that can be covalently attached to a protein. Therefore, 4-bis (3, 5-dibromo-4-hydroxyphenyl) valeric acid, which has a carboxyl group and is similar in structure to TBBPA, was chosen as the hapten. The hapten was covalently attached to BSA for immunogen synthesis using a carbodiimide method (Li et al., Citation2013). The hapten (20 mg) was dissolved in methanol (2 ml); EDC (3 mg) and NHS (3 mg) were added and allowed to react under constant stirring for 4 h at room temperature (solution a). BSA (24 mg) was dissolved in 0.01 M CB (pH 9.6) as solution b. Solution a was subsequently added dropwise to solution b under constant stirring and allowed to react at room temperature for 8 h. The reaction mixture was transferred to a pretreated dialysis bag, dialyzed against PBS for 3 days, and stored at −20°C. BSA conjugates were prepared to immunize BALB/c mice. The hapten was covalently coupled to OVA for coating antigen synthesis in a similar manner.
Production of mAb
Firstly, the immunogen hapten–BSA (100 µg) was emulsified with isovolumetric FCA and then subcutaneously injected into the backs of four female BALB/c mice (6–8 weeks of age) (Suryoprabowo, Liu, Peng, Kuang, & Xu, Citation2014; Zhang et al., Citation2013). Every three weeks, 50 µg of the complete antigen was emulsified with isovolumetric FIA for enhanced immunizations. After 10 weeks, blood samples were collected from the tail of each mouse and measured by ic-ELISA. Following five immunizations, the mouse with the lowest half-maximum inhibition concentration (IC50) was selected for cell fusion. After 3 days of the last intraperitoneal injection, the mouse was sacrificed to obtain the spleen and blood from ocular was collected simultaneously.
Cells with good sensitivity and low IC50 values were selected for subcloning experiments 7 days after cell fusion. The optimum cell line was selected for amplification culture and intraperitoneally injected into two mice for ascite production. Seven days later, ascites were collected and purified by caprylic acid and ammonium sulfate precipitation (Yan, Liu, Xu, Kuang, & Xu, Citation2015; Yin, Liu, Song, Kuang, & Xu, Citation2014). Antibody concentrations were measured by UV–visible spectroscopy at 280 nm and stored at –20°C.
Determination of mAb sensitivity and specificity using ic-ELISA
TBBPA mAb sensitivity was assessed by ic-ELISA (Kuang et al., Citation2013; Liu, Yan, Zhang, Kuang, & Xu, Citation2014) using checkerboard assays to evaluate the optimum type and concentration of coating antigen and antibody (Chen et al., Citation2013).
For ic-ELISA optimization, different variables were evaluated including pH value (5.0, 6.0, 7.4, 8.6, and 9.6), ionic strength (0, 0.1, 0.2, and 0.5% Tween), and organic solvent concentration (0, 10, 20, 30, and 40% methanol). The obtained data were calculated according to the four-parameter logistic equation using the OriginProv8.5 software, and then the standard curve was generated.
Cross-reactivity
TBBPA mAb specificity was assessed by cross-reactivity (CR) (%) experiments using a series of structural analogues. CR was calculated with the following equation:
Pretreatment of samples
Lake water and rice pudding samples were TBBPA-negative based on LC-MS/MS results. The TBBPA standard was dissolved in methanol and diluted to different concentrations (0, 2, 5, 10, 25, and 50 ng/ml). Different amounts of TBBPA standard (0, 2, 5, and 10 ng) were added to 1 g of homogeneous rice pudding, followed by 3 ml of ethyl acetate. The sample was subjected to ultrasonic extraction for 20 min and centrifuged for 2400g for 15 min to collect the organic phase. The resulting supernatant was evaporated to dryness and dissolved in 10 ml BB. Different amounts of TBBPA standard (0, 0.2, 0.5, and 1 ng) were added to 1 ml lake water. After shaking, the prepared samples were used. Every analysis was repeated at least three times.
Preparation of gold nanoparticle-labeled mAb
According to our previous works (Guo et al., Citation2014; Liu, Luo, et al., Citation2014), gold nanoparticles were prepared using the sodium citrate reduction method. The prepared TBBPA mAb (0.5 ml) was added dropwise to the colloidal gold solution (20 ml) whose pH was adjusted to pH 7.0 with 0.1 M potassium carbonate (K2CO3). Following a 50-min equilibration, the mixture was stirred for 2 h, mixed with 0.5% (w/v) casein, and centrifuged at 8000 rpm for 30 min to remove excess gold nanoparticles, blocking reagent, and mAb. The mixture was centrifuged three times to collect the lower layer (colloidal gold nanoparticle-labeled mAb). Gold nanoparticle-labeled mAb was suspended in 1 ml borate buffer (0.01M, pH 8.6) with 0.02% sodium azide (NaN3), and the optical density (OD) was adjusted to 3.0 at 520 nm. The conjugates were stored at 4°C.
Assembly of the immunochromatographic test strip
The immunochromatographic test strip for TBBPA detection was based on the microparticle immunoassay. The components of the immunochromatographic test strip included a nitrocellulose pad, an absorption pad, a sample pad, and a conjugated pad (Shukla, Leem, & Kim, Citation2011).
According to the ic-ELISA, the coating antigen (T3-OVA) was sprayed onto the nitrocellulose membrane to produce the test line, and the goat anti-mouse IgG was drawn onto the NC membrane to produce the control line. The conjugate pad was prepared by spraying the captured reagent (T3-OVA and goat anti-mouse IgG) onto a glass fiber membrane. The NC membrane was dried at 37°C initially for 30 min and subsequently for 2 h. All the pads were assembled to the plastic backing plate, which was cut into 5-mm long and 5-mm wide strips using a strip cutter (Liu, Xing, Yan, Kuang, & Xu, Citation2014; Wang et al., Citation2015; Xu et al., Citation2015).
Immunochromatographic strip assay
A TBBPA stock solution was prepared by dissolving 2 mg TBBPA in 2 ml of methanol. A series of TBBPA standards was prepared (0, 10, 15, 20, 25, and 50 ng/ml) from the stock solution. TBBPA standards (100 µl) were mixed with gold nanoparticle-labeled mAb and added to the sample pad. After 5 min, the result was examined visually.
Results and discussion
Preparation of anti-TBBPA antigens
Firstly, the immunogen hapten T3-BSA (100 µg) was used to produce TBBPA mAb. The peak of T3-BSA was significantly different (at 310 and 280 nm) from that of T3, BSA using the UV–Vis absorption spectrum, so as the T3-OVA ().
TBBPA mAb sensitivity and specificity
To determine the mAb sensitivity, the protocol for ic-ELISA was used. To obtain the optimum concentration of coating antibody and antigen, checkerboard assays were performed. According to the mouse blood test, T3-OVA had lower IC50 values than other coating antigens. Therefore, T3-OVA were selected for the following assays. Based on the optimized conditions mentioned before, the best concentration of the coating antigen and the mAb was 0.3and 0.1 μg/ml, respectively. As shown in (a), the suitable maximum and the lowest IC50 were gained at the pH 8.6. Methanol concentration had little effect on the maximum OD value (b); however, it had a significant effect on IC50. The lowest IC50 value was obtained at 0 methanol. Finally, Tween-20 concentration had little effect on the maximum OD value, but had significant effects on IC50. High IC50 values were obtained with 0.2% and 0.5% Tween-20; therefore, 0.1% Tween-20 was selected for the following assays. Based on the optimized conditions, a standard curve was generated; IC50 was 0.44 ng/ml, IC20 was 0.149 ng/ml, and IC80 was 1.316 ng/ml.
Figure 2. Optimization of assay buffer for ic- ELISA system and standard content in assay buffer on ic-ELISA performance; (a) effects of pH about assay buffer on ic-ELISA performance; (b) effects of methanol content in assay buffer on ic-ELISA performance; (c) effects of Tween-20 content in assay buffer on ic-ELISA performance; (d) standard inhibition curve for ic-ELISA analysis of TBBPA. Each point of inhibition curve represents six replicates in analysis.
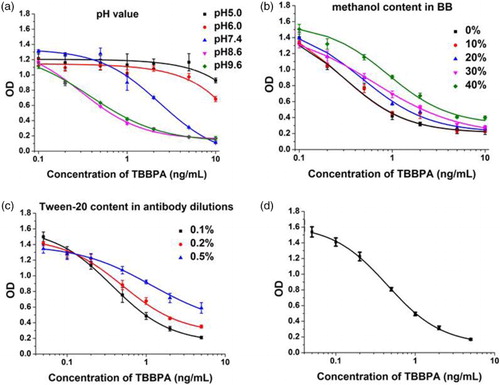
To confirm mAb sensitivity and specificity, an immunoglobulin isotype test was performed to assess the isotype. The results revealed that mAb had a IgG1 isotype. Secondly, CR experiments were performed with four structural TBBPA analogues: bisphenol A (BPA), bisphenol C (BPC), bisphenol F (BPF), and bisphenol S (BPS). As shown in , there was no CR even at 1 µg/ml. Therefore, TBBPA mAb was highly specific.
Table 1. Cross-reactivity results under the optimized ic-ELISA conditions.
Recovery assays
Lake water and rice pudding were chosen for recovery test. The rice pudding ingredients were so complex that it had a big difference on OD value. Thus, to eliminate matrix interference, the sample was diluted 10 times after dried. Following the serial dilution, the sample was spiked with 0, 2, 5, and 10 ng/ml TBBPA. The lake water samples were directly spiked with TBBPA at 0, 0.2, 0.5, and 1 ng/ml. shows that the average recoveries of TBBPA from lake water and rice pudding were 70.7–108.8% and 110.2–119.8%, respectively. The results indicated the ic-ELISA was sensitive and available for the detection of TBBPA in lake water and rice pudding samples.
Table 2. Recovery results for lake water and rice pudding.
Preparation of gold nanoparticle-labeled mAb
The results of the immunochromatographic test strip are rapidly determined (∼5–10 min) based on the red color of the gold nanoparticles. The size of the nanoparticles may affect the sensitivity of the strip. Additionally, pH is an important factor in this assay, because it affects the isoelectric point of the antibody. Based on our previous findings (Guo et al., Citation2014; Liu, Xing, et al., Citation2014; Wang et al., Citation2015; Xu et al., Citation2015), 20 nm gold nanoparticles was used for labeling mAb, and the conjugation reaction between mAb and colloidal gold nanoparticles was performed at pH 8.2.
Optimization of the immunochromatographic test strip
To assess the optimum coating antigen and mAb, five types of coating antigen and three types of mAb were evaluated (1F2, 2B6, and 3E4). The optimum concentrations were 0.5 mg/ml coating antigen, 1 ml of gold nanoparticle, 4 µl K2CO3, and 8 µg/ml mAb. Coating antigen T3-OVA provided the best results, while 3E4 cell strain had the lowest IC50 value (25 and 50 ng/ml; ).
Figure 3. Images of TBBPA analysis using the colloidal gold immunochromatographic strip assay in different samples (a) PBS; (b) rice pudding and (c) lake water. The concentrations of TBBPA were 0, 5, 10, 25, 50, 100 ng/ml (1 = 0, 2 = 5 ng/ml, 3 = 10 ng/ml, 4 = 25 ng/ml, 5 = 50 ng/ml, 6 = 100 ng/ml).
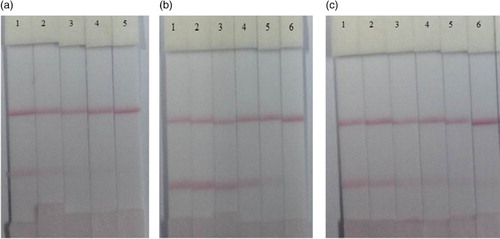
Immunochromatographic test strip for TBBPA detection in lake water and rice pudding
The immunochromatographic test strip was used to detect TBBPA in lake water and rice pudding samples. Different concentrations of TBBPA (0, 5, 10, 25, 50, and 100 ng/ml) were added to lake water and rice pudding samples. As shown in , the cut-off value and threshold concentration (i.e. where the test line of the strip could not be seen visually any more) were 50 ng/ml (b) and 25 ng/ml (c), respectively. The test strip results were evaluated in 10 min. In comparison to ic-ELISA, the immunochromatographic test strip is more convenient and quicker for the on-site detection of TBBPA.
Conclusion
A specific TBBPA mAb with an IC50 value 0.44 ng/ml was prepared using T3 as the hapten. An ic-ELISA was developed for TBBPA detection in lake water and rice pudding samples. This analytical method had high sensitivity and specificity, low detection limits, and good recovery. An immunochromatographic test trip was developed using TBBPA mAb labeled with gold nanoparticles. The TBBPA cut-off value of the test strip was 25 ng/ml in lake water and 50 ng/ml in rice pudding. In conclusion, the ic-ELISA and immunochromatographic test strip are rapid, simple, and sensitive for TBBPA detection in lake water and rice pudding samples.
Disclosure statement
No potential conflict of interest was reported by the authors.
Notes on contributors
Li Yu obtained her bachelor’s degree from Henan Agricultural University, Zhengzhou, China in 2013 and her master’s degree in food science from Jiangnan University (Wuxi, China). Her research interest includes immunoassay development for food contact materials.
Liqiang Liu earned his Ph.D. in Food science in 2014 from Jiangnan University, Wuxi, China and then became a faculty in college of Food science and technology of Jiangnan University. His research interests include immunochromatographic strip design and application.
Shanshan Song received her Master’s degree in Food science in 2012 from Jiangnan University, Wuxi, China and then became a research assistant in college of Food science and technology of Jiangnan University. Her research interests are monoclonal antibody development.
Hua Kuang obtained her Ph.D. from China Agricultural University in 2009 and then began to work as a faculty in college of Food science and technology of Jiangnan University. She is currently a full professor in food safety. Her research interests are biosensor development.
Chuanlai Xu is a full professor of Food science and technology of Jiangnan University. He earned his Ph.D. in food science in 2002. His research interests are fast detection technology and food safety evaluation.
Additional information
Funding
References
- Bu, D., Zhuang, H., Zhou, X., & Yang, G. (2014). Biotin-streptavidin enzyme-linked immunosorbent assay for detecting Tetrabromobisphenol A in electronic waste. Talanta, 120, 40–46. doi: 10.1016/j.talanta.2013.11.080
- Chen, X., Xu, L., Ma, W., Liu, L., Kuang, H., Wang, L., & Xu, C. (2013). General immunoassay for pyrethroids based on a monoclonal antibody. Food and Agricultural Immunology, 25(3), 341–349. doi: 10.1080/09540105.2013.794328
- Covaci, A., Voorspoels, S., Abdallah, M. A., Geens, T., Harrad, S., & Law, R. J. (2009). Analytical and environmental aspects of the flame retardant tetrabromobisphenol-A and its derivatives. Journal of Chromatography A, 1216(3), 346–363. doi: 10.1016/j.chroma.2008.08.035
- Driffield, M., Harmer, N., Bradley, E., Fernandes, A. R., Rose, M., Mortimer, D., & Dicks, P. (2008). Determination of brominated flame retardants in food by LC-MS/MS: Diastereoisomer-specific hexabromocyclododecane and tetrabromobisphenol A. Food Addit Contam Part A Chem Anal Control Expo Risk Assess, 25(7), 895–903. doi: 10.1080/02652030701882999
- Ezechias, M., Covino, S., & Cajthaml, T. (2014). Ecotoxicity and biodegradability of new brominated flame retardants: A review. Ecotoxicology and Environmental Safety, 110, 153–167. doi: 10.1016/j.ecoenv.2014.08.030
- Feng, A. H., Chen, S. J., Chen, M. Y., He, M. J., Luo, X. J., & Mai, B. X. (2012). Hexabromocyclododecane (HBCD) and tetrabromobisphenol A (TBBPA) in riverine and estuarine sediments of the Pearl River Delta in southern China, with emphasis on spatial variability in diastereoisomer- and enantiomer-specific distribution of HBCD. Marine Pollution Bulletin, 64(5), 919–925. doi: 10.1016/j.marpolbul.2012.03.008
- Geens, T., Roosens, L., Neels, H., & Covaci, A. (2009). Assessment of human exposure to Bisphenol-A, Triclosan and Tetrabromobisphenol-A through indoor dust intake in Belgium. Chemosphere, 76(6), 755–760. doi: 10.1016/j.chemosphere.2009.05.024
- Germer, S., Piersma, A. H., van der Ven, L., Kamyschnikow, A., Fery, Y., Schmitz, H. J., & Schrenk, D. (2006). Subacute effects of the brominated flame retardants hexabromocyclododecane and tetrabromobisphenol A on hepatic cytochrome P450 levels in rats. Toxicology, 218(2–3), 229–236. doi: 10.1016/j.tox.2005.10.019
- Guo, J., Liu, L., Xue, F., Xing, C., Song, S., Kuang, H., & Xu, C. (2014). Development of a monoclonal antibody-based immunochromatographic strip for cephalexin. Food and Agricultural Immunology, 26(2), 282–292. doi: 10.1080/09540105.2014.907242
- He, M. J., Luo, X. J., & Yu, L. H. (2010). Tetrabromobisphenol-A and Hexabromocyclododecane in birds from an E-Waste Region in South China: Influence of diet on diastereoisomer- and enantiomer-specific distribution and trophodynamics. Environmental Science and Technology, 44, 5748–5754. doi: 10.1021/es101503r
- Kajiwara, N., Hirata, O., Takigami, H., Noma, Y., Tachifuji, A., & Matsufuji, Y. (2014). Leaching of brominated flame retardants from mixed wastes in lysimeters under conditions simulating landfills in developing countries. Chemosphere, 116, 46–53. doi: 10.1016/j.chemosphere.2014.01.025
- Kefeni, K. K., Okonkwo, J. O., Olukunle, O. I., & Botha, B. M. (2011). Brominated flame retardants: Sources, distribution, exposure pathways, and toxicity. Environmental Reviews, 19, 238–253. doi: 10.1139/a11-010
- Kuang, H., Liu, L., Xu, L., Ma, W., Guo, L., Wang, L., & Xu, C. (2013). Development of an enzyme-linked immunosorbent assay for dibutyl phthalate in liquor. Sensors (Basel), 13(7), 8331–8339. doi: 10.3390/s130708331
- Kuiper, R. V., Canton, R. F., Leonards, P. E., Jenssen, B. M., Dubbeldam, M., Wester, P. W., … Vethaak, A. D. (2007). Long-term exposure of European flounder (Platichthys flesus) to the flame-retardants tetrabromobisphenol A (TBBPA) and hexabromocyclododecane (HBCD). Ecotoxicology and Environmental Safety, 67(3), 349–360. doi: 10.1016/j.ecoenv.2006.12.001
- Li, Z., Liu, L., Song, S., Guo, S., Kuang, H., & Xu, C. (2013). Development of an enzyme-linked immunosorbent assay for octylphenol. Food and Agricultural Immunology, 25(3), 397–410. doi: 10.1080/09540105.2013.821597
- Liu, L., Luo, L., Suryoprabowo, S., Peng, J., Kuang, H., & Xu, C. (2014). Development of an immunochromatographic strip test for rapid detection of ciprofloxacin in milk samples. Sensors (Basel), 14(9), 16785–16798. doi: 10.3390/s140917621
- Liu, L., Xing, C., Yan, H., Kuang, H., & Xu, C. (2014). Development of an ELISA and immunochromatographic strip for highly sensitive detection of microcystin-LR. Sensors (Basel), 14(8), 14672–14685. doi: 10.3390/s140814672
- Liu, L., Yan, H., Zhang, X., Kuang, H., & Xu, C. (2014). Development of an anti-chlorothalonil monoclonal antibody based on a novel designed hapten. Food and Agricultural Immunology, 26(3), 410–419. doi: 10.1080/09540105.2014.938319
- Lyche, J. L., Rosseland, C., Berge, G., & Polder, A. (2015). Human health risk associated with brominated flame-retardants (BFRs). Environment International, 74, 170–180. doi: 10.1016/j.envint.2014.09.006
- Ma, M., Crump, D., Farmahin, R., & Kennedy, S. W. (2015). Comparing the effects of tetrabromobisphenol-A, bisphenol A, and their potential replacement alternatives, TBBPA-bis(2,3-dibromopropyl ether) and bisphenol S, on cell viability and messenger ribonucleic acid expression in chicken embryonic hepatocytes. Environmental Toxicology and Chemistry, 34(2), 391–401. doi: 10.1002/etc.2814
- Nakao, T., Akiyama, E., Kakutani, H., Mizuno, A., Aozasa, O., Akai, Y., & Ohta, S. (2015). Levels of tetrabromobisphenol A, tribromobisphenol A, dibromobisphenol A, monobromobisphenol A, and bisphenol A in Japanese breast milk. Chemical Research in Toxicology, 28(4), 722–728. doi: 10.1021/tx500495j
- Pratt, I., Anderson, W., Crowley, D., Daly, S., Evans, R., Fernandes, A., … Tlustos, C. (2013). Brominated and fluorinated organic pollutants in the breast milk of first-time Irish mothers: Is there a relationship to levels in food? Food Addit Contam Part A Chem Anal Control Expo Risk Assess, 30(10), 1788–1798. doi: 10.1080/19440049.2013.822569
- Puype, F., Samsonek, J., Knoop, J., Egelkraut-Holtus, M., & Ortlieb, M. (2015). Evidence of waste electrical and electronic equipment (WEEE) relevant substances in polymeric food-contact articles sold on the European market. Food Addit Contam Part A Chem Anal Control Expo Risk Assess, 32(3), 410–426.
- Samsonek, J., & Puype, F. (2013). Occurrence of brominated flame retardants in black thermo cups and selected kitchen utensils purchased on the European market. Food Addit Contam Part A Chem Anal Control Expo Risk Assess, 30(11), 1976–1986. doi: 10.1080/19440049.2013.829246
- Sanchez-Brunete, C., Miguel, E., & Tadeo, J. L. (2009). Determination of tetrabromobisphenol-A, tetrachlorobisphenol-A and bisphenol-A in soil by ultrasonic assisted extraction and gas chromatography-mass spectrometry. Journal of Chromatography A, 1216(29), 5497–5503. doi: 10.1016/j.chroma.2009.05.065
- Shi, Z., Jiao, Y., Hu, Y., Sun, Z., Zhou, X., Feng, J., … Wu, Y. (2013). Levels of tetrabromobisphenol A, hexabromocyclododecanes and polybrominated diphenyl ethers in human milk from the general population in Beijing, China. Science of the Total Environment, 452–453, 10–18. doi: 10.1016/j.scitotenv.2013.02.038
- Shukla, S., Leem, H., & Kim, M. (2011). Development of a liposome-based immunochromatographic strip assay for the detection of Salmonella. Analytical and Bioanalytical Chemistry, 401(8), 2581–2590. doi: 10.1007/s00216-011-5327-2
- Stubbings, W. A., & Harrad, S. (2014). Extent and mechanisms of brominated flame retardant emissions from waste soft furnishings and fabrics: A critical review. Environment International, 71, 164–175. doi: 10.1016/j.envint.2014.06.007
- Suryoprabowo, S., Liu, L., Peng, J., Kuang, H., & Xu, C. (2014). Development of a broad specific monoclonal antibody for fluoroquinolone analysis. Food Analytical Methods, 7(10), 2163–2168. doi: 10.1007/s12161-014-9863-1
- Svihlikova, V., Lankova, D., Poustka, J., Tomaniova, M., Hajslova, J., & Pulkrabova, J. (2015). Perfluoroalkyl substances (PFASs) and other halogenated compounds in fish from the upper Labe River basin. Chemosphere, 129, 170–178. doi: 10.1016/j.chemosphere.2014.09.096
- Tada, Y., Fujitani, T., Yano, N., Takahashi, H., Yuzawa, K., Ando, H., … Kamimura, H. (2006). Effects of tetrabromobisphenol A, brominated flame retardant, in ICR mice after prenatal and postnatal exposure. Food and Chemical Toxicology, 44(8), 1408–1413. doi: 10.1016/j.fct.2006.03.006
- Ten Dam, G., Pardo, O., Traag, W., van der Lee, M., & Peters, R. (2012). Simultaneous extraction and determination of HBCD isomers and TBBPA by ASE and LC-MSMS in fish. Journal of Chromatography. B, Analytical Technologies in the Biomedical and Life Sciences, 898, 101–110. doi: 10.1016/j.jchromb.2012.04.025
- Wang, W., Feng, M., Kong, D., Liu, L., Song, S., & Xu, C. (2015). Development of an immunochromatographic strip for the rapid detection of Pseudomonas syringae pv. maculicolain broccoli and radish seeds. Food and Agricultural Immunology, 26(5), 738–745. doi: 10.1080/09540105.2015.1023266
- Xu, C., Ou, J., Cui, Y., Wang, L., Lv, C., Liu, K., … Liu, S. (2013). Development of a monoclonal antibody-based enzyme-linked immunosorbent assay for tetrabromobisphenol A. Monoclon Antib Immunodiagn Immunother, 32(2), 113–118. doi: 10.1089/mab.2012.0099
- Xu, N., Xu, L., Ma, W., Liu, L., Kuang, H., & Xu, C. (2015). An ultrasensitive immunochromatographic assay for non-pretreatment monitoring of chloramphenicol in raw milk. Food and Agricultural Immunology, 26(5), 635–644. doi: 10.1080/09540105.2014.998640
- Xu, T., Wang, J., Liu, S. Z., Lu, C., Shelver, W. L., Li, Q. X., & Li, J. (2012). A highly sensitive and selective immunoassay for the detection of tetrabromobisphenol A in soil and sediment. Analytica Chimica Acta, 751, 119–127. doi: 10.1016/j.aca.2012.06.030
- Yan, H., Liu, L., Xu, N., Kuang, H., & Xu, C. (2015). Development of an immunoassay for carbendazim based on a class-selective monoclonal antibody. Food and Agricultural Immunology, 26(5), 659–670. doi: 10.1080/09540105.2015.1007446
- Yin, Y., Liu, L., Song, S., Kuang, H., & Xu, C. (2014). Development of a highly sensitive icELISA to detect semicarbazide based on a monoclonal antibody. Food and Agricultural Immunology, 26(3), 356–365. doi: 10.1080/09540105.2014.914891
- Zhang, X., Feng, M., Liu, L., Xing, C., Kuang, H., Peng, C., … Xu, C. (2013). Detection of aflatoxins in tea samples based on a class-specific monoclonal antibody. International Journal of Food Science & Technology, 48(6), 1269–1274. doi: 10.1111/ijfs.12086