ABSTRACT
Analytical methodology to detect ricin and Shiga toxins (Stx) in food matrices is important for food safety and biosecurity. Monoclonal antibodies (mAbs) that bind each toxin were used for capture in sandwich enzyme-linked immunosorbent assay, and IgY polyclonal antibodies were prepared as detection antibodies. The ricin assay systems, using colorimetric or chemiluminescent substrates, detected native, but not heat-denatured ricin. The lower limit of detection (LOD) was 0.13 ng mL−1 in milk and 0.8 ng g−1 in ground beef. The Stx2 assay systems detected native Stx2, but not heat-denatured Stx2 or Stx1. The LOD was 0.13 ng mL−1 in milk and 0.7 ng g−1 in ground beef. Using a standard 96-well-plate format, the assays can detect less than 1 × 10−4 of an estimated lethal oral dose of either toxin in a serving of milk. The IgY detection antibodies for ricin were more heat-stable than mouse polyclonal anti-ricin at 65°C.
KEYWORDS:
1. Introduction
Ricin and Shiga toxins (Stx) are both globular ribosomal-inactivating proteins (RIPs) with catalytic A subunits and receptor-binding B subunits (Stirpe, Citation2004). Ricin is a dimeric toxin that is found in the mash resulting from industrial castor seed processing, along with the closely related, but much less toxic tetrameric Ricinus communis agglutinin-1 (RCA-1). Ricin has been a tool of bioterrorists (CDC, Citation2003), and consequently castor production is fraught with the risk of diversion to criminal purposes (Auld, Trostle, Miller, He, & Duncan, Citation2014; Severino et al., Citation2012). Stx include the classical Shigella dysenteriae toxin (O'Brien, LaVeck, Griffin, & Thompson, Citation1980) and two Shiga toxin-producing E. coli (STEC) toxins – Stx1, essentially identical to classical Stx, and the closely related Stx2, which occurs as multiple variants (Jackson, Neill, O'Brien, Holmes, & Newland, Citation1987; Paton, Bourne, Manning, & Paton, Citation1995). Despite structural differences among the holotoxins (Fraser et al., Citation2004), all Stx have a catalytic A-chain and accessory B-chains, with AB5 stoichiometry. Stx1 and Stx2 are principal pathogenicity factors in outbreaks of STEC-caused disease (Smith, Fratamico, & Gunther, Citation2014) and contribute to a complex and variable set of sequellae (Keithlin, Sargeant, Thomas, & Fazil, Citation2014), including hemolytic uremic syndrome. Although animal models provide the most direct means for assessing the toxicity and bioavailability in complex food matrices, in vitro tests for toxins, including immunochemical formats, provide essential analytical data for food safety assurance (Brandon & Carter, Citation2012). Both polyclonal (pAb) and monoclonal antibody (mAb) reagents have been developed that bind ricin (Brandon & Hernlem, Citation2009; Garber & O'Brien, Citation2008; Poli, Rivera, Hewetson, & Merrill, Citation1994; reviewed by He, Citation2014) and Stx 2 (He et al., Citation2013; He, Patfield, Hnasko, Rasooly, & Mandrell, Citation2013; Strockbine, Marques, Holmes, & O'Brien, Citation1985) with high affinity. Enzyme-linked immunosorbent assay (ELISA) and other formats afford high assay sensitivities and are adaptable for use in even more highly amplified assay systems (He, McMahon, McKeon, & Brandon, Citation2010; He et al., Citation2011). In addition, activity assays, such as the inhibition of translation of luciferase mRNA by these toxic RIPs, are useful for quantification of active toxin (He, Quiñones, Carter, & Mandrell, Citation2009; Rasooly & He, Citation2012). The use of antibodies in sample preparation or to suppress toxin activity can make these mRNA translation assays specific for an individual toxin (Hale, Citation2001; He et al., Citation2009).
Although mammalian IgG is used in the overwhelming majority of published ELISAs, IgY is an evolutionary predecessor of IgG (Warr, Magor, & Higgins, Citation1995), and it is an interesting variation on the “immunoglobulin theme” that has been used for both analytical and potential therapeutic purposes (Neri et al., Citation2011; Pauly et al., Citation2009). Chickens incorporate IgY into their eggs at about 50 mg/egg (Pauly, Chacana, Calzado, Brembs, & Schade, Citation2011). IgY possesses an extra domain compared to classical rabbit or mouse IgG with four heavy chain domains. The “stiffer” hinge region may render it more stable (to acid or heat, for example), particularly in the presence of sugars and other polyols (Losch, Schranner, Wanke, & Jurgens, Citation1986; Shimizu, Nakai, & Fitzsimmons, Citation1988; Shimizu, Nagashima, Hashimoto, & Suzuki, Citation1994). Additionally, the avian immune repertoire has provided the basis of recombinant antibody fragments for food immunoanalysis (Fitzgerald, Leonard, Darcy, Danaher, & O'Kennedy, Citation2011). IgY has been reported against a variety of toxins, such a B. cereus enterotoxin (Machaiah & Krishnan, Citation2014) and staphylococcal enterotoxins (Jin et al., Citation2013), and was demonstrated to neutralize Stx2 (Parma et al., Citation2011; Feng, Lui, & Shi, Citation2013; Arimitsu, Sasaki, Kohda, Shimizu, & Tsuji, Citation2014). In this report, we present the development of IgY ELISAs for the protein toxins, ricin and Stx2, and compare the thermal stabilities of mouse and chicken antibodies.
2. Materials and methods
2.1. Buffers
The principal buffers used in this study were phosphate-buffered saline (PBS, 5 mM Na2HPO4, 0.15 M NaCl, pH 7.0), PBS-0.05% (v/v) Tween®-20 (PBST). PBST plus 10 mg/mL bovine serum albumin, and 0.1 mg/mL thimerosal was used as ELISA diluent. For ELISA of ricin, the diluent for analyte, detection antibody, and secondary reagents also contained 100 mM galactose to minimize lectin interactions.
2.2. Toxins and toxoid
Ricin and RCA-1 were obtained from Vector Laboratories (Burlingame, CA, USA). Crude ricin was prepared from castor seeds as described previously (Brandon, Citation2011), and contained 2.4 mg/mL ricin. Stx2 was obtained from Toxin Technology (Sarasota, FL, USA) and was specified as 50% pure. Stx2 toxoid was prepared by a modification of the procedure described by Nakao et al. (Citation1999). Stx2 at a final concentration of 0.4 mg/mL was incubated for seven days at 37°C in 0.2% formaldehyde, 10 mM lysine, 50 mM Na phosphate, pH 7.6. The formalinized toxoid was dialyzed and stored frozen. Toxins were heat-denatured by incubation at 90°C for 30 min. Antibody cross-reactivity with Stx1 was tested using an analog developed as a genetic toxoid, Stx1E167Q (Skinner, Patfield, Stanker, Fratamico, & He, Citation2014).
2.3. Milk and ground beef analyses
Fresh nonfat and whole (3.3% fat) milk and ground beef (labeled as 90% lean) samples were obtained from local retailers. Stored under refrigeration, milk was used within one week of purchase, and ground beef, within two days. Samples were spiked with ricin or Stx2 preparations using working dilutions (0.1–10 µg/mL) in PBS. Milk samples were then diluted with an equal volume of ELISA diluent for analysis. Ground beef was homogenized and analyzed at a 1:5 dilution, as previously described (Brandon, Citation2011). Briefly, 4 g portions on ice were spiked (8 µL), thoroughly mixed, and then homogenized in 8 mL PBS (with 100 mM galactose for ricin analysis) using a saw-tooth generator probe (two 30 s intervals at 30,000 rpm).
2.4. Antibodies and assays
2.4.1. Monoclonal antibodies and their conjugates
MAb 1443 anti-ricin mouse mAb, was used as capture Ab for ricin (Brandon & Hernlem, Citation2009). Anti-Stx2 mouse mAb 11E10 (Toxin Technology) was used as a capture antibody for coating plates in Stx2 detection assays.
2.4.2. Husbandry of chickens
Husbandry of chickens and antibody production procedures were conducted under a protocol approved by the Western Regional Research Center's Animal Care and Use Committee, in accordance with the requirements of the US Animal Welfare Act and guidelines of the US Public Health Service. Female white Leghorn chickens were initially reared in a brooder on Start and Grow feed containing the coccidiostat, amprolium (Purina Mills, Gray Summit, MO, USA) and then transferred to standard laboratory poultry caging that facilitated egg collection. At 18 weeks, the feed was changed to Layena high-calcium diet (Purina).
2.4.3. IgY production
Stx2 toxoid or ricin A-chain at 0.2 mg/mL was emulsified with an equal volume of metabolizable monophosphoryl A – trehalose dicorynomycolate adjuvant (Sigma Chemical, St. Louis, MO, USA). Hens were inoculated intramuscularly with 0.2 mL emulsion (about 20 µg immunogen) divided between 2 sites at week 13, and 2 (Stx2) or 3 (ricin) booster inoculations were administered at three to six-week intervals thereafter. Test bleeds were collected from the brachial vein to confirm the immune response, and eggs were collected daily and stored at 4°C. Highest IgY titers were obtained from eggs 10–40 days after booster inoculation.
2.4.4. IgY purification
IgY was partially purified by differential precipitation with polyethyleneglycol solutions as described by Polson, von Wechmar, and Fazakerley (Citation1980). The final precipitate was resuspended in and dialyzed against PBS. The preparation was clarified by centrifugation, diluted to 1 mg/mL (using A280, 1 mg/mL = 1.35), and stored in aliquots at −20°C. IgY (anti-ricin) was used at 10 µg/mL; IgY (anti-Stx2) was used as 2.5 µg/mL, based on preliminary titrations by ELISA to determine the working range of reagents.
2.4.5. ELISA plates
Colorimetric ELISAs were performed on clear polystyrene Immulon 4HBX plates or strips (Dynex, Chantilly, VA, USA). Chemiluminescent ELISAs utilized black polystyrene Fluotrac 600 assay plates or strips (Greiner Bio-One, Longwood, FL, USA) to minimize the cross-talk between wells observed with white plates. Plates or strip assemblies were coated by adsorption of ricin capture mAb 1443 at 5 µg/mL and Stx2 capture mAb 11E10 at 4 µg/mL. For thermal stability studies, Immulon plates were coated with ricin at 5 µg/mL. Plates were washed, blocked, dried, and stored desiccated as described previously (Brandon & Hernlem, Citation2009).
2.4.6. Toxin immunosorbent assay procedures and data analysis
Sandwich ELISAs were conducted with 60 min incubations at ambient temperature for samples, and 30 min incubations for antibodies and secondary reagents. Well contents were removed and five washes with water were done between steps. The contents of the wells from toxin incubation and first rinse were collected and inactivated using sodium hypochlorite. IgY was detected with horseradish (HRP)-conjugated donkey anti-IgY heavy and light chain antibody at 20 ng mL−1 (Gallus Immunotech, Fergus, ON, Canada) or goat anti-IgY Fc at 100 ng mL−1 (Thermo-Pierce, Rockford, IL, USA), with essentially identical results. Tetramethylbenzidine (TMB) substrate (100 µL/well) was used for colorimetric assays (Neogen, Lexington, KY, USA) with 100 µL/well 0.3N HCl stop reagent and PS-Atto substrate (Lumigen, Southfield, MI, USA) was used for chemiluminescence (200 µL/well). Plate reading and data analysis were performed as described previously (Brandon, Korn, & Yang, Citation2012), using the model M2 plate reader with SoftMax Pro v5.3 (Molecular Devices, Sunnyvale, CA, USA) for colorimetric assays and a Wallac Victor 2 luminometer with Manager v3, rev.5 (PerkinElmer, Shelton, CT, USA). Toxin concentrations for standards and spikes were 0, 0.1, 0.2, 0.5, 1, 2, 5, 10, and 20 ng/mL (for buffer, milk) or ng/g (for ground beef), with a narrower spiking range utilized for repeated experiments. Experiments were conducted with triplicate or greater replication, using two or three well replicates for each data point. Typical plots are shown in the figures.
2.5. Thermal stability study
The thermal stability of mouse mAbs and pAbs and chicken IgY antibodies to ricin was tested by direct-binding colorimetric ELISA on ricin-coated assay wells. In addition to the IgY preparations described in this article, we used mouse anti-ricin mAbs 1443, 1642, and 1797 and mouse pAb – two pooled mouse antisera collected during immunization for mAb preparation and stored at −20° (Brandon & Hernlem, Citation2009). Preliminary experiments were conducted to identify relevant time–temperature conditions and antibody concentration to achieve a final A450–A650 of 2 ± 0.5 under standard ELISA conditions. Antibody solutions diluted in PBS (mouse pAb, 1:100; IgY or mAb at 10 µg mL−1) were heated in 12 × 75 mm glass tubes in a thermostat-controlled heating block for 0, 5, 20, or 80 min at 65°, then rapidly cooled on ice. Antibodies were further diluted in ELISA diluent by the following factors for analysis: IgY #78, 25; IgY #79 and mouse pAb A, 200; mAbs 1642 and 1797 and mouse pAb B, 100; mAb 1443, 80. Secondary ELISA reagents in this study were HRP-goat anti-IgY Fc and HRP-rabbit anti-mouse IgG (Invitrogen, Medford, OR, USA). Resulting absorbance readings were expressed relative to controls (0 min heating) that were normalized to 100%.
2.6. Quantitative and statistical analysis
ELISA standard curves were fit to a 4-parameter logistic equation using SlideWrite version 7.01 (Advanced Graphics, Rancho Santa Fe, CA, USA) and were used for quantitation of analytes and for determining cross-reactivities. Cross-reactivity expressed as a percent was calculated from the reciprocal of the concentration of analyte producing 1 absorbance unit (colorimetric detection) or 1 × 106 luminescence units (chemiluminescent detection) under standard ELISA conditions, relative to the value for toxin standard. Paired t-tests and graphical determination of limit of detection (LOD) based on one-sided 95% confidence intervals were performed using SlideWrite.
3. Results and discussion
3.1. Specificity of ELISAs with IgY detection of toxin
After conducting preliminary experiments (checkerboard titrations) to determine the dilution of reagents for optimal detection of analyte, and we proceeded to determine assay specificity and sensitivity. shows the standard curves for colorimetric and chemiluminescent ELISA of ricin and Stx2 in buffer, using IgY and HRP-donkey anti-IgY for detection. All four assays yielded typical sigmoidal standard curves. summarizes the specificity of the ELISAs for the two toxins. The ricin assay primarily detected native toxin, as indicated by the 1% cross-reactivity with heat-denatured ricin. This specificity permits the estimation of residual ricin activity in thermally processed, contaminated products. The assay was highly selective for ricin, compared to RCA-1, which had 6% cross-reactivity. Though significant, the cross-reactivity level is acceptable for screening analyses because samples containing crude ricin would be expected to contain RCA-1 as well. A positive assay of a food sample would indicate the presumptive occurrence of intentional contamination and the need for follow-up assays and actions to safeguard the food supply.
Figure 1. Standard curves for ELISA of ricin with (a) colorimetric, and (b) chemiluminescent detection; and ELISA of Stx2 with (c) colorimetric and (d) chemiluminescent detection. Typical results are illustrated for analysis of toxins in buffer matrix.
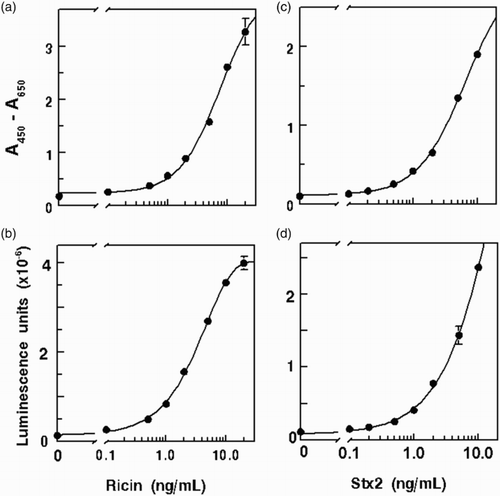
Table 1. Specificity of IgY ELISAs for ricin and Stx.
Data for IgY ELISA detection of Stx2 () show that these assays were specific for native toxin, with <1% cross-reactivity by heat-denatured Stx2. There was no detectable recognition of Stx1E167Q, an analog that differs by 1 amino acid from authentic Stx1 and that induced Stx1-specific antibody production in mice (Skinner et al., Citation2014). Thus, the IgY ELISA had the requisite specificity for detection of Stx2, the toxin most frequently associated with contamination by STEC.
3.2. Performance of ELISAs in food matrices
3.2.1. Detection of ricin in milk
Typical data for determining LOD in milk are shown in . Most determinations yielded LODs close to the lowest spiking level, 0.1 ng/mL, which defines the lowest LOD reported in this study. The details are summarized in . Differences between detection modes as well as differences among different sample types were not significant (p > .05). Details on recovery of ricin from milk are summarized in . The differences in recoveries between colorimetric and chemiluminescence and between recoveries for different milk fat levels were not significant (p > .05), Overall, we found that ricin spiked at levels between 0.5 and 10 ng/mL was recovered at a level of 60 ± 9.6%.
Figure 2. Lower LOD for toxins in nonfat milk by ELISA. The 90% confidence intervals are drawn, and the broken line indicates the value for the blank + 3 s.d. (a) ricin, colorimetric, LOD = 0.1 ng/mL; (b) ricin, chemiluminescent, LOD = 0.1 ng mL−3; (c) Stx2, colorimetric, LOD = 0.1 ng/mL; (d) Stx2, chemiluminescent, LOD = 0.14 ng/mL.
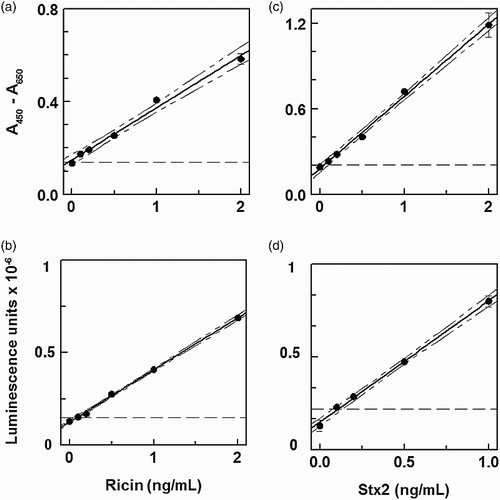
Table 2. LOD for ricin and Stx2 in buffer and food samples by IgY ELISA.
Table 3. Recovery of ricin and Stx2 from spiked food samples by IgY ELISA.
3.2.2. Detection of Stx2
Typical data for detection of Stx2 in milk are shown in . Similar to the results obtained for ricin, the LODs were very close to the lowest spiking level, 0.1 ng/mL. The details for LODs in buffer, nonfat and whole milk are summarized in . There was no significant difference between LODs for nonfat vs. whole milk or between detection methods (colorimetric vs. chemiluminescent). Overall, the IgY ELISAs yielded an LOD of 0.13 ± 0.03. The recovery of Stx2 spikes between 0.5 and 10 ng/mL for all methods was 81 ± 13%. As shown in detail in , differences in recoveries between detection methods and between milk fat contents were not significant.
3.2.3. Analysis of ground beef for toxins.
For the colorimetric assays, LODs were generally in the 2–3 ng g−1 range (data not shown), but the LODs were lower with chemiluminescent detection. We therefore proceeded with ground beef analyses using only the chemiluminescent format. shows that the LOD in ground beef by chemiluminescent ELISA was 0.8 ng g−1 for ricin and 0.7 ng g−1 for Stx2. These LODs were 5–10-fold higher than those obtained in milk, possibly owing to binding or sequestration of protein toxins by particulates in the samples. However, the LOD for ricin was in the range we had previously obtained using a mouse mAb sandwich immunosorbent assay (0.5 ng g−1 by electrochemiluminescent analysis; ng g−1 by ELISA, Brandon, Citation2011). summarizes recoveries of toxins from spiked ground beef. Ricin recovery was about 85% with a 10% standard deviation, higher than obtained in the earlier study using mouse mAbs. The recovery of Stx2 (90%) was not significantly different, but the results had a greater standard deviation (about 30%).
3.3. Stability of antibodies
We next investigated whether the anti-toxin IgY preparations represented ligands with enhanced thermal stability compared to mouse IgG antibodies. To address this question, we used a direct-binding ELISA and conducted preliminary experiments to determine a suitable temperature for monitoring thermal inactivation. Heating at 65° was the condition selected for detailed study, and the results are presented in and . shows that both pAb preparations lost binding activity gradually at 65°. After 80 min at 65°, IgY lost about 25% and mouse serum IgG lost about 50% activity. Three mouse mAbs were also tested and the results of one typical experiment are illustrated in . Two (mAbs 1443 and 1797) were very stable at 65°, but the third, mAb 1642, was labile. MAb 1642 had proven very effective for assays of ricin in foods and castor seeds (He et al., Citation2010; McKeon, Auld, Brandon, Leviatov, & He, Citation2014). These assays were conducted at neutral pH at ambient temperature (about 20°). However, we also used the mAbs in an optical sensor system and found that mAb 1642 was too acid-labile to permit regeneration using 10 s pulses with 10 mM glycine-HCl, pH 1.7 (Brandon, Adams, Yang, & Korn, Citation2014). We hypothesize that the same structural features than confer acid lability also render mAb 1642 heat-labile. The three mAbs used in this study were selected by conventional hybridoma methodology on the basis of their specificity in direct-binding and sandwich ELISA under ambient conditions and neutral pH. They are thus a subset of individual “accessions” in the immune system's diverse library of antibodies assembled in response to immunization (Tonegawa, Citation1983). In contrast, the serum antibodies represent a complex mixture of molecules that vary in structure. Polyclonal antibodies and many mouse mAbs are remarkably stable even under harsh conditions, for example, in the presence of denaturants or pH extremes used in regenerating immunosensors and immunoaffinity supports (Harlow & Lane, Citation1999). Each mAb, however, exhibits individual specificity and potentially atypical stability, as is apparently the case for mAb 1642.
4. Conclusions
This study demonstrated that IgY antibodies obtained from chicken eggs could be used effectively to detect two toxins, ricin and Stx 2, in milk and ground beef samples. In our experience IgY has served poorly for capture in sandwich ELISA, but functioned well for detection. The ELISAs had sensitivity in the 0.1 ng g−1 range, comparable to other reported sandwich ELISAs without amplification (Brandon, Korn, & Yang, Citation2014). However, amplification by use of polymerase chain reaction can routinely increase ELISA sensitivity about 100-fold (He et al., Citation2010). Although the study of thermal stability indicated that IgY was more stable than mouse polyclonal Ab at 65°, mouse mAbs used in many of our studies shared the high thermal stability of IgY (e.g. mAbs 1443 and 1797; Brandon, Adams, et al., Citation2014). IgY could be especially useful when a large quantity of PBS pAb is required, but it remains to be seen whether other features of IgY, such as its altered hinge region, afford advantages in sensor methodology. Stability and flexibility of the antibody molecule may play a more pivotal role in sensing techniques such as quartz crystal microbalance assays (Chu, Lin, Chen, Chen, & Wen, Citation2012), fluorescence resonance transfer methodology (Vinayaka, Muthukumar, & Thakur, Citation2013), and homogenous assays such as fluorescence polarization methods (Mi, Wang, Eremin, Shen, & Zhang, Citation2013).
Acknowledgements
The authors appreciated the assistance of Wanless Hatcher and Zeke Martinez with animal husbandry, and thank Lily L. Yang for assistance with antibody development. Xiaohua He is gratefully acknowledged for provision of the Stx1E167Q analog.
Disclosure statement
No potential conflict of interest was reported by the authors.
Notes on contributors
David L. Brandon, Ph.D., is a Research Chemist with interest in immunochemical analysis of foodborne contaminants and antinutrients.
Anna M. Korn, M.A., is a Biological Science Laboratory Technician (retired), with certification in clinical laboratory science.
Additional information
Funding
Notes
† Mention of trade names or commercial products in this article is solely for the purpose of providing specific information and does not imply recommendation or endorsement by USDA. USDA is an equal opportunity provider and employer.
References
- Arimitsu, H., Sasaki, K., Kohda, T., Shimizu, T., & Tsuji, T. (2014) Evaluation of Shiga toxin 2e-specific chicken egg yolk immunoglobulin: Production and neutralization activity. Microbiology and Immunology, 58, 643–648. doi: 10.1111/1348-0421.12197
- Auld, D. L., Trostle, C. L., Miller, T. D., He, X. & Duncan, R. W. (2014). Risk of ricin from commercial castor production in North America. In J. Cherwonogrodzky (Ed.), Ricin toxin (pp. 86–97). Beijing: Bentham Science.
- Brandon, D. L. (2011) Detection of ricin contamination in ground beef by electrochemiluminescence immunosorbent assay. Toxins, 3, 398–408. doi: 10.3390/toxins3040398
- Brandon, D. L., Adams, L. M., Yang, L. L., & Korn, A. M. (2014) Antibody interactions with Ricinus communis agglutinins studied by biolayer interferometry. Analytical Letters, 47, 1747–1758. doi: 10.1080/00032719.2014.886693
- Brandon, D. L., & Carter, J. M. (2012) Immunoassay. In D.-W. Sun (Ed.), Handbook of food safety engineering (pp. 279–312). London: Blackwell.
- Brandon, D. L., & Hernlem, B. J. (2009) Development of monoclonal antibodies specific for Ricinus agglutinins. Food and Agricultural Immunology, 20, 11–22. doi: 10.1080/09540100802626487
- Brandon, D. L., Korn, A. M., & Yang, L. L. (2012) Detection of ricin contamination in liquid egg by electrochemiluminescence immunosorbent assay. Journal of Food Science, 77, T83–T88. doi: 10.1111/j.1750-3841.2012.02627.x
- Brandon, D. L., Korn, A. M., & Yang, L. L. (2014) Immunosorbent analysis of ricin contamination in milk using colorimetric, chemiluminescent and electrochemiluminescent detection. Food and Agricultural Immunology, 25, 160–172. doi: 10.1080/09540105.2012.753515
- CDC. (2003) Investigation of a ricin-containing envelope at a postal facility – South Carolina, 2003. MMWR. Morbidity and Mortality Weekly Report, 52, 1129–1131.
- Chu, P. T., Lin, C. S., Chen, W. J., Chen, C. F., & Wen, H. W. (2012) Detection of gliadin in foods using a quartz crystal microbalance biosensor that incorporates gold nanoparticles. Journal of Agricultural and Food Chemistry, 60, 6483–6492. doi: 10.1021/jf2047866
- Feng, Y., Liu, W., & Shi, D. (2013) Effectiveness of egg yolk antibody against Shiga toxin ii variant toxicity in vitro and in vivo. Current Microbiology, 67, 448–453. doi: 10.1007/s00284-013-0384-8
- Fitzgerald, J., Leonard, P., Darcy, E., Danaher, M., & O'Kennedy, R. (2011) Light-chain shuffling from an antigen-biased phage pool allows 185-fold improvement of an anti-halofuginone single-chain variable fragment. Analytical Biochemistry, 410, 27–33. doi: 10.1016/j.ab.2010.11.009
- Fraser, M. E., Fujinaga, M., Cherney, M. M., Melton-Celsa, A. R., Twiddy, E. M., O'Brien, A. D., & James, M. N. (2004) Structure of Shiga toxin type 2 (Stx2) from Escherichia coli O157:H7. Journal of Biological Chemistry, 279, 27511–27517. doi: 10.1074/jbc.M401939200
- Garber, E. A. E., & O'Brien, T. W. (2008) Detection of ricin in food using electrochemiluminescence-based technology. Journal of AOAC International, 91, 376–382.
- Hale, M. L. (2001) Microtiter-based assay for evaluating the biological activity of ribosome-inactivating proteins. Pharmacology Toxicology, 88, 255–260. doi: 10.1111/j.1600-0773.2001.880506.x
- Harlow E, Lane D. (1999) Using antibodies: A laboratory manual. Cold Spring Harbor, NY: Cold Spring Harbor Lab. Press.
- He, X. (2014) Current technologies for detection of ricin in different matrices. In J. Cherwonogrodzky (Ed.), Ricin toxin (pp. 38–56). Beijing: Bentham Science.
- He, X., McMahon, S., McKeon, T. A., & Brandon, D. L. (2010) Development of a novel immuno-PCR assay for detection of ricin in ground beef, liquid chicken egg and milk. Journal of Food Protection, 73, 695–700.
- He, X., McMahon, S. A., Skinner, C. B., Merrill, P. A., Scotcher, M. C., & Stanker, L. H. (2013) Development and characterization of monoclonal antibodies against Shiga toxin 2 and their application for toxin detection in milk. Journal of Immunological Methods, 389, 18–28. doi: 10.1016/j.jim.2012.12.005
- He, X., Patfield, S., Hnasko, R., Rasooly, R., & Mandrell, R. E. (2013) A polyclonal antibody based immunoassay detects seven subtypes of Shiga toxin 2 produced by Escherichia coli in human and environmental samples. PLoS One, 8, e76368. doi:10.1371/journal.pone.0076368.
- He, X., Qi, W., Quinones, B., McMahon, S., Cooley, M., & Mandrell, R. E. (2011) Sensitive detection of Shiga toxin 2 and some of its variants in environmental samples by a novel immuno-PCR assay. Applied and Environmental Microbiology, 77, 3558–3564. doi: 10.1128/AEM.02205-10
- He, X., Quiñones, B., Carter, J. M., & Mandrell, R. E. (2009) Validation of a cell-free translation assay for detecting Shiga toxin 2 in bacterial culture. Journal of Agricultural & Food Chemistry, 57, 5084–5088. doi: 10.1021/jf9002285
- Jackson, M. P., Neill, R. J., O'Brien, A. D., Holmes, R. K., & Newland, J. W. (1987) Nucleotide sequence analysis and comparison of the structural genes for Shiga-like toxin I and Shiga-like toxin II encoded by bacteriophages from Escherichia coli 933. FEMS Microbiology Letters, 44, 109–114. doi: 10.1111/j.1574-6968.1987.tb02252.x
- Jin, W., Yamada, K., Ikami, M., Kaji, N., Tokeshi, M., Atsumi, … Ohta, M. (2013) Application of IgY to sandwich enzyme-linked immunosorbent assays, lateral flow devices, and immunopillar chips for detecting staphylococcal enterotoxins in milk and dairy products. Journal of Microbiological Methods, 92, 323–331. doi: 10.1016/j.mimet.2013.01.001
- Keithlin, J., Sargeant, J., Thomas, M. K., & Fazil, A. (2014) Chronic sequelae of E. coli O157: Systematic review and meta-analysis of the proportion of E. coli O157 cases that develop chronic sequelae. Foodborne Pathogens and Disease, 11, 79–95. doi: 10.1089/fpd.2013.1572
- Losch, U., Schranner, I., Wanke, R., & Jurgens, L. (1986). Kapnographie – eine Methode zur Beurteilung der Ventilationslage während der Allgemeinanästhesie beim Pferd. Journal of Veterinary Medicine Series A, 33, 609–616. doi: 10.1111/j.1439-0450.1986.tb00076.x
- Machaiah, M. I., & Krishnan, M. H. (2014) Immunodetection of Bacillus cereus haemolytic enterotoxin (HBL) in food samples. Analytical Methods, 6, 1841–1847. doi: 10.1039/c3ay41737a
- McKeon, T. A., Auld, D., Brandon, D. L., Leviatov, S., & He, X. (2014) Toxin content of commercial castor cultivars. Journal of the American Oil Chemists' Society, 91, 1515–1519. doi: 10.1007/s11746-014-2505-3
- Mi, T., Wang, Z., Eremin, S. A., Shen, J., & Zhang, S. (2013) Simultaneous determination of multiple (fluoro)quinolone antibiotics in food samples by a one-step fluorescence polarization immunoassay. Journal of Agricultural and Food Chemistry, 61, 9347–9355. doi: 10.1021/jf403972r
- Nakao, H., Kataoka, C., Kiyokawa, N., Fujimoto, J., Yamasaki, S., & Takeda, T. (1999) Monoclonal antibody to Shiga toxin 2 which blocks receptor binding and neutralizes cytotoxicity. Infection and Immunity, 67, 5717–5722.
- Neri, P., Tokoro, S., Kobayashi, R., Sugiyama, T., Umeda, K., Shimizu, T., … Mori, H. (2011) Specific egg yolk immunoglobulin as a new preventive approach for shiga-toxin-mediated diseases. PLoS One, 6, e26526. doi:10.1371/journal.pone.0026526.
- O'Brien, A. D., LaVeck, G. D., Griffin, D. E., & Thompson, M. R. (1980) Characterization of Shigella dysenteriae 1 (Shiga) toxin purified by anti-Shiga toxin affinity chromatography. Infection and Immunity, 30, 170–179.
- Parma, Y. R., Chacana, P. A., Rogé, A., Kahl, A., Cangelosi, A., Geoghegan, P., … Fernandez-Miyakawa, M. E. (2011) Antibodies anti-Shiga toxin 2 B subunit from chicken egg yolk: Isolation, purification and neutralization efficacy. Toxicon, 58, 380–388. doi: 10.1016/j.toxicon.2011.07.009
- Paton, A. W., Bourne, A. J., Manning, P. A., & Paton, J. C. (1995) Comparative toxicity and virulence of Escherichia coli clones expressing variant and chimeric Shiga-like toxin type II operons. Infection and Immunity, 63, 2450–2458.
- Pauly, D., Chacana, P. A., Calzado, E. G., Brembs, B., & Schade, R. (2011) IgY technology: Extraction of chicken antibodies from egg yolk by polyethylene glycol (PEG) precipitation. Journal of Visualized Experiments. doi:10.3791/3084.
- Pauly, D., Dorner, M., Zhang, X., Hlinak, A., Dorner, B., & Schade, R. (2009) Monitoring of laying capacity, immunoglobulin Y concentration, and antibody titer development in chickens immunized with ricin and botulinum toxins over a two-year period. Poultry Science, 88, 281–290. doi: 10.3382/ps.2008-00323
- Poli, M. A., Rivera, V. R., Hewetson, J. F., & Merrill, G. A. (1994) Detection of ricin by colorimetric and chemiluminescence ELISA. Toxicon, 32, 1371–1377. doi: 10.1016/0041-0101(94)90409-X
- Polson, A., von Wechmar, M. B., & Fazakerley, G. (1980) Antibodies to proteins from yolk of immunized hens. Immunology Communications, 9, 495–514. doi: 10.3109/08820138009066011
- Rasooly, R., & He, X. (2012) Sensitive bioassay for detection of biologically active ricin in food. Journal of Food Protection, 75, 951–954. doi: 10.4315/0362-028X.JFP-11-448
- Severino, L. S., Auld, D. L., Baldanzi, M., Candido, M. J. D., Chen, G., Crosby, W., … Zieler, H. (2012) A review on the challenges for increased production of castor. Journal of Visualized Experiments, 104, 853–880.
- Shimizu, M., Nagashima, H., Hashimoto, K. & Suzuki, T. (1994) Egg yolk antibody (Ig Y) stability in aqueous solution with high sugar concentrations. Journal of Food Science, 59, 763–765.
- Shimizu, M., Nakai, S., & Fitzsimmons, R. C. (1988) Anti-E. coli immunoglobulin Y isolated from egg yolk of immunized chickens as a potential food ingredient. Journal of Food Science, 53, 1360–1368. doi: 10.1111/j.1365-2621.1988.tb09277.x
- Skinner, C., Patfield, S., Stanker, L. H., Fratamico, P., & He, X. (2014) New high-affinity monoclonal antibodies against Shiga toxin 1 facilitate the detection of hybrid Stx1/Stx2 in vivo. PLoS One, 9, e9985. doi:10.1371/journal.pone.0099854.
- Smith, J. L., Fratamico, P. M., & Gunther, N. W. (2014) Shiga toxin-producing Escherichia coli. Advances in Applied Microbiology, 86, 145–197. doi: 10.1016/B978-0-12-800262-9.00003-2
- Stirpe, F. (2004) Ribosome-inactivating proteins. Toxicon, 44, 371–383. doi: 10.1016/j.toxicon.2004.05.004
- Strockbine, N. A., Marques, L. R., Holmes, R. K., & O'Brien, A. D. (1985) Characterization of monoclonal antibodies against Shiga-like toxin from Escherichia coli. Infection and Immunity, 50, 695–700.
- Tonegawa, S. (1983) Somatic generation of antibody diversity. Nature, 302, 575–581. doi: 10.1038/302575a0
- Vinayaka, A. C., Muthukumar, S. P., & Thakur, M. S. (2013) Avian antibodies for staphylococcal enterotoxin B as an efficient tool for FRET-based fluoroimmunosensor. BioNanoScience, 3, 232–240. doi: 10.1007/s12668-013-0101-x
- Warr, G. W., Magor, K. E., & Higgins, D. A. (1995) IgY: Clues to the origins of modern antibodies. Immunology Today, p. 16, 392–398.