ABSTRACT
The objective of the present study was to examine the effect of yoghurt peptides on expression of various pro- and anti-inflammatory genes by ovine monocytes and neutrophils. A total of eight commercial traditional-type Greek yoghurts made with ovine or bovine milk were used. Water-soluble extracts (WSEs) were obtained from all samples. Peptides present in WSE induced cyclooxygenase-2 gene expression and down-regulated inducible NO synthase, Interleukin-1 Receptor Antagonist and Ιnterleukin-12β gene expression in both cell types. Treatment of neutrophils with WSE resulted in a decrease in Interleukin-1β gene expression relative to control. There was a different response from monocytes and neutrophils regarding Transforming Growth Factor-β1 gene expression with an increase by monocytes and a decrease by neutrophils relative to control. Expression of Ιnterferon-γ and Ιnterleukin-10 remained unaffected. In conclusion, peptides derived from Greek yoghurt exhibited a mixed effect (pro- and anti-inflammatory) on two immunocompetent cell populations, although the anti-inflammatory effect was more pronounced.
1. Introduction
Proteolytic breakdown of milk proteins generates a number of peptides endowed with various biological properties (antimicrobial, antihypertensive, antithrombotic, and immunomodulatory activities) (Baldi et al., Citation2005; Korhonen & Pihlanto, Citation2007). The ability of milk-born peptides to modulate the function of monocytes and neutrophils, two key cellular components of the non-specific (innate) immunity, has been previously demonstrated (Baldi et al., Citation2005; Politis & Chronopoulou, Citation2008). Work from our laboratory (Politis & Chronopoulou, Citation2008) has demonstrated that two specific peptides, namely, the tripeptide Leu-Leu-Tyr and the hexapeptide Pro-Gly-Pro-Ileu-Pro-Asp which correspond to residues 191–193 and residues 63–68 of bovine β-casein, respectively, act in an anti-inflammatory manner by down-regulating the cascade of reactions leading to plasminogen activation by porcine blood mononuclear cells. The protein kinase A system and/or the exchange protein directly activated by cyclic adenosine monophosphate (Epac-1) are implicated in the mechanism through which both peptides act in an anti-inflammatory manner.
Whether yoghurt contains peptides capable of modulating immune response is not known with certainty. In yoghurt the main source of peptides is the deliberate proteolysis caused by lactic acid bacteria cultures. The proteolytic machinery of lactic acid bacteria includes a cell wall bound proteinase and several intracellular peptidases (Law & Haandrikman, Citation1997). The transport system of these bacteria enables them to internalize oligopeptides up to 18 amino acids in length. Longer oligopeptides is a major source of bioactive peptides in yoghurt when they are further degraded by gastrointestinal enzymes or by intracellular peptidases of lysed bacteria following yoghurt consumption (Law & Haandrikman, Citation1997). However, milk proteins can be subjected to inadvertent proteolysis in milk prior to yoghurt manufacturing caused by native in milk proteolytic systems (i.e. plasmin) during storage of milk before processing. Lastly, inadvertent proteolysis can be caused by the proteolytic machinery of the natural milk microflora present in milk. Pasteurization minimizes the ability of these microorganisms to grow but their proteolytic enzymes remain within milk and the resulting yoghurt (Adolfsson, Meydani, & Russell, Citation2004).
The inflammatory response aims at defeating threatening infections or other stressors, and it is characterized by the activation of a broad panel of cytokines, chemokines, interleukins, inducible cyclooxygenase (COX-2; PTGS2), and inducible nitric oxide synthase (iNOS; NOS2) which mediate many features of the inflammatory processes, involving tissue degradation, proliferation signals, recruitment of macrophages and other immune cells, and angiogenesis. iNOS is the main source of NO production and it has been associated with injury and inflammation. iNOS is expressed in many cell types, including macrophages, neutrophils, dendritic cells, endothelial cells, and epithelial cells (Bogdan, Citation2001). As iNOS produces a large amount of NO, it has been assumed that such NO is harmful and plays an effector function. This is true of neutrophils and macrophages. However, NO also plays an immunoregulatory role in the induction and resolution of inflammation (Kobayashi, Citation2010). COX-2, normally induced by inflammatory stimuli, hormones, and growth factors, is the more important source of prostaglandins (PGs), which play a key role in the generation of the inflammatory response formation (Dubois et al., Citation1998). However, it also contributes to the generation of autoregulatory and homeostatic prostanoids. The profile of prostanoid production, which is determined by the differential expression of COX-2 within cells present at sites of inflammation (Tilley, Coffman, & Koller, Citation2001), plays a key role in the generation of the inflammatory response. Interferon-γ (IFN-γ; IFNG) production is controlled by cytokines secreted by antigen-presenting cells, most notably Interleukin-12 (IL-12β; IL12B) and Interleukin-18 (Munder, Mallo, Eichmann, & Modolell, Citation1998, Citation2001). Negative regulators of IFN-γ production include Interleukin-10 (IL-10; IL10) and Transforming Growth Factor-β1 (TGF-β1; TGFB1) (Fukao et al., Citation2001; Schindler, Lutz, Rollinghoff, & Bogdan, Citation2001). Interleukin-1β (IL-1β; IL1B) is a pro-inflammatory cytokine that exerts pleiotrophic effects on a variety of cells and play key roles in acute and chronic inflammation (Dinarello, Citation1998). IL-12's major functions include induction of IFN-γ production, enhancement of cytotoxity of natural killer cells, and differentiation of naive T cells into Th1 effectors (Denkers, Del Rio, & Bennouna, Citation2003; Gately et al., Citation1998; Ma & Trinchieri, Citation2001). TGF-β is a pleiotropic cytokine with potent regulatory and inflammatory activity (Li & Flavell, Citation2008a, Citation2008b). The multi-faceted effects of TGF-β on numerous immune functions are cellular and environmental context dependent (Li, Wan, Sanjabi, Robertson, & Flavell, Citation2006). IL-10 limits the immune response during infection and thus prevents immune system-mediated damage to the host (Li & Flavell Citation2008a). However, high production of IL-10 is associated with sustained chronic infections and its blockade promotes pathogen clearance (Couper, Blount, & Riley, Citation2008). It is produced by almost every type of cell of the immune system, including monocytes and neutrophils (Moore, de Waal Malefyt, Coffman, & O'Garra, Citation2001; O'Garra, Barrat, Castro, Vicari, & Hawrylowicz, Citation2008; Sabat et al., Citation2010). Interleukin-1 Receptor Antagonist (IL-1ra; IL1RN) blocks the action of IL-1β functional ligands by competitive inhibition at the IL-1 receptor level. IL-1ra binds with equal or greater affinity than does IL-1β to the type-1 membrane-bound IL-1 receptor. IL-1ra does not bind with high affinity to the type-II IL-1 receptor (Dinarello, Citation1997; Sims et al., Citation1993). The anti-inflammatory cytokines IL-4, IL-6, IL-10, and IL-13 inhibit the synthesis of IL-1β, yet they stimulate the synthesis of IL-1ra (Dinarello, Citation1997).
To the best of our knowledge, there are no studies reporting the effect of yoghurt peptides on mRNA expression of cytokines and other inflammation-related genes in monocytes and neutrophils. Thus, the objective of the present study was to investigate the ability of Water-soluble extracts (WSEs) obtained from yoghurts made from bovine and ovine milk to modulate the expression of various pro-inflammatory and anti-inflammatory genes by activated ovine monocytes and neutrophils. Neutrophils and monocytes are important cellular effectors of the innate immune defense, and it is clear that circulating monocytes also contribute significantly to the defense against a range of microbial pathogens (Geissmann et al., Citation2008; Serbina, Jia, Hohl, & Pamer, Citation2008).
2. Materials and methods
2.1. Materials
Unless otherwise stated all reagents were purchased from Sigma Chemical (St. Louis, MO).
2.2. Yoghurt samples
A total of eight traditional-type commercial Greek yoghurt brands were selected to participate in the present experiment. Four yoghurt brands were made using ovine and another four brands were made using bovine milk. All brands selected had comparable composition of main nutrients (fat 4.5%, protein 5.6%, and total solids 15.8%). The protein composition of the four yoghurt brands made from bovine milk was elevated to the level of 5.6% by protein fortification. Yoghurt samples were stored for up to 25 days at 4°C.
2.3. Water-soluble extracts
WSEs were obtained from all eight yoghurts at days 3 and 25 of storage using the method proposed by Kuchroo and Fox (Citation1982). In more detail, 20 g of water was added to 20 g of yoghurt and the sample was homogenized in Stomacher lab blender for 5 min. The sample was then incubated at 40°C for 1 h and centrifuged at 3000×g for 30 min. Supernatant was filtered through glass wool and aliquots were kept at −80°C. The determination of WSE protein concentration was performed using the Kjeldahl method (N × 6.38) (AOAC, Citation1990).
2.4. Determination of immunomodulatory activity
The immunomodulatory activity of WSE was tested by measuring their ability to modulate the expression of several pro- and anti-inflammatory genes by ovine monocytes and neutrophils. Monocytes and neutrophils were isolated using methods previously described by us (Politis, Theodorou, Lampidonis, Chronopoulou, & Baldi, Citation2012). The purity of monocytes was 92% (88–97%). Cell viability was assessed by the trypan blue dye exclusion methodology (typically >95% of total cells obtained were viable). All reagents and water used were endotoxin-free to avoid monocyte activation. Following isolation, cells were cultured in cell culture medium (RPMI 1640 containing 2 mmol/L L-glutamine, 1.5 g/L HCO3, and 10 mmol/L HEPES supplemented with 5.5 mmol/L glucose, 1 mmol/L sodium pyruvate, 50 mg/mL penicillin and streptomycin and 10% fetal bovine serum) in the presence or absence of crude WSE (0.3 mg/mL). All treatments were performed in duplicate while six samples without addition of WSE were used as a control group. After 3 h of incubation, cell culture medium was removed, cells were washed three times with PBS and then phorbol myristate acetate (PMA, 81 nmol/L) was added to activate the cells. After incubation for 30 min, cells were washed again three times with PBS and then processed for RNA isolation.
Total RNA was extracted from approximately 5 × 106 cells using TriReagent (Sigma-Aldrich Co., USA) according to the manufacturer's instructions. Extracted RNA was treated with DNAseI using a commercially available kit (New England Biolabs). The quality and quantity of the RNA extracted were confirmed by spectrophotometry as well as gel electrophoresis. Relative levels of mRNA for target genes (IL1B, IL12B, IL10, IL1RN, IFNG, TGFB1, NOS2, and PTGS2) were quantified with real-time, quantitative RT-PCR using SYBR Green chemistry. The amount of sample RNA was normalized by using glyceraldehyde 3-phosphate dehydrogenase (GAPDH) and β-actin (ACTB) as housekeeping genes. A pair of primers for each of the genes used in this study was constructed using PERLprimer software (Marshall, Citation2004) and all primers were designed to be specific for Capra hircus and Ovis aries except for ACTB where it was not possible (). Total RNA (100–500 ng) was reverse transcribed with the PrimeScript First Strand cDNA Synthesis Kit (Takara), according to the manufacturer's instructions using a mix of random hexamers and oligo-dT primers. Real-time PCR was performed in the 7500 Real-Time system (Applied Biosystems) using the SYBR® Select Master Mix (Life Technologies) according to the manufacturer's protocol. Each reaction (10 μl) contained 5 ng RNA equivalents as well as 300 nmol/L of forward and reverse primers for each gene. The reactions were incubated at 95°C for 2 min followed by 40 cycles of 15 s at 95°C and 60 s at 60°C. This was followed by a melt curve analysis to determine the reaction specificity. Each sample was measured in duplicate. A modified version of the Pfaffl (Citation2001) normalization method was used for normalization against the two reference genes (Hellemans, Mortier, De Paepe, Speleman, & Vandesompele, Citation2007).
Table 1. Sequences and relative positions of forward (F) and reverse (R) primers for Interleukin-1B (IL1B), Interleukin-12B (IL12B), Interleukin-10 (IL10), Interleukin-1 Receptor Antagonist (IL1RN), Interferon gamma (IFNG), Transforming Growth Factor-β1 (TGFB1), inducible nitric oxide synthase (NOS2) that is the name of the gene although the name of the protein product is iNOS, cyclooxygenase 2 (PTGS2), glyceraldehyde 3-phosphate dehydrogenase (GAPDH), and β-actin (ACTB) used in real-time PCR.
2.5. Statistical analysis
Data were analyzed using the SPSS statistical package (version 17.0). For all relative expression analyses, the control group was set to 1 and the expression of the treatments with bovine and ovine WSE is presented as x-fold expression to the control group. The normality of the distribution for each variable was tested using the Kolmogorov–Smirnov test, while the homogeneity of variance using the Levene's test. Departures from homogeneity of variance were found for the variables that correspond to the expression of IL12B, NOS2, and IL1RN in neutrophils. For these variables, the Welch's test with the Dunnett's T3 as post hoc test was used for comparison of means. All other comparisons between groups were conducted by one-way ANOVA using Tukey's HSD post hoc test and data are given as means ± SE. Significance for all cases was set at 0.05. All data presented are derived from three independent experiments.
3. Results
The ability of WSE obtained from yoghurts made from bovine or ovine milk to modulate the expression of NOS2 and PTGS2 by ovine monocytes and neutrophils was examined and the results are presented in . Data indicate that treatment with WSE derived from ovine yoghurt downregulates the expression of NOS2 by both neutrophils and monocytes, relative to the control group, while the same effect applies to bovine WSE treatment only in neutrophils. In contrast, PTGS2 is induced by both bovine and ovine WSE in monocytes, relative to the control group, while in neutrophils only bovine WSE induces expression.
Figure 1. Effect of treatment of ovine monocytes (a) and neutrophils (b) with WSE obtained from ovine and bovine yoghurt on the expression of inducible nitric oxide synthase (NOS2) and cyclooxygenase-2 (PTGS2). Values are means ± SE. Differences between columns labeled with different letters are statistically significant at P < .05. All comparisons between groups were conducted by one-way ANOVA using Tukey's HSD post hoc test, except for NOS2 in neutrophils (b) where Welch's test with Dunnett's T3 post hoc was used due to a departure from homogeneity.
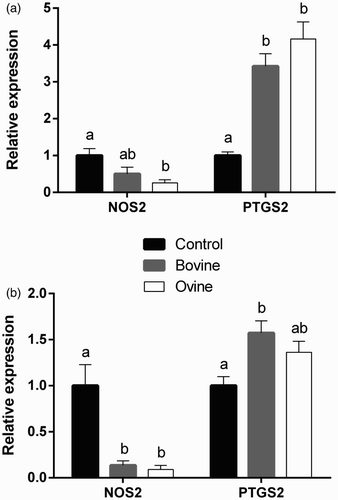
Changes in the expression of IL1B, IL12B, and IFNG by ovine monocytes and neutrophils following treatment with WSE from bovine and ovine yoghurt are presented in . A significant decrease in mRNA expression of IL1B was recorded in neutrophils following treatment with ovine and bovine WSE, relative to the control group. No statistically significant differences between the three groups were observed in IL1B expression by monocytes. IL12B mRNA expression was reduced in monocytes and neutrophils following treatment with WSE from ovine yoghurt, relative to the control group, whereas treatment with WSE from bovine yoghurt reduced IL12B expression in monocytes only. There were no statistically significant changes in IFNG expression by either type of cells.
Figure 2. Effect of treatment of ovine monocytes (a) and neutrophils (b) with WSE obtained from ovine and bovine yoghurt on the expression of Interleukin-1β (IL1B), Interleukin-12β (IL12B), and Interferon-γ (IFNG). Values are means ± SE. Differences between columns labeled with different letters are statistically significant at P < .05. All comparisons between groups were conducted by one-way ANOVA using Tukey's HSD post hoc test, except for IL12B in neutrophils (b) where Welch's test with Dunnett's T3 post hoc was used due to a departure from homogeneity.

The effect of WSE treatment on the expression of genes that are considered to have mainly anti-inflammatory role is presented in . IL10 expression remains unaffected in both cell types following treatment with yoghurt WSE. IL1RN expression, on the other hand, is down-regulated in both cell types following treatment with WSE from ovine yoghurt, relative to the control group, while a decrease in expression is also evident by treatment with WSE from bovine yoghurt in neutrophils only. Finally, the effect of the treatment with yoghurt WSE on the expression of TGFB1 in the two cell populations is the exact opposite. While the neutrophils exhibit reduced expression of TGFB1 following treatment with yoghurt WSE, relative to the control group, there is an upregulation of gene expression in monocytes.
Figure 3. Effect of treatment of ovine monocytes (a) and neutrophils (b) with WSE obtained from ovine and bovine yoghurt on the expression of Interleukin-10 (IL10), Interleukin-1 Receptor Antagonist (IL1RN), and Transforming Growth Factor-β1 (TGFB1). Values are means ± SE. Differences between columns labeled with different letters are statistically significant at P < .05. All comparisons between groups were conducted by one-way ANOVA using Tukey's HSD post hoc test, except for IL1RN in neutrophils (b) where Welch's test with Dunnett's T3 post hoc was used due to a departure from homogeneity.
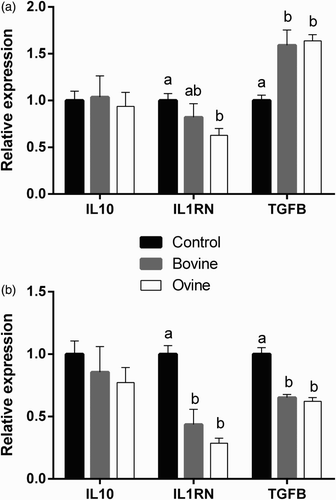
4. Discussion
The present study reports for the first time immunomodulating effects of peptides derived from Greek traditional yoghurt in two immunocompetent cell populations. Results indicate that peptides from yoghurts made from bovine or ovine milk act in many cases in a similar manner modulating the expression of pro- and anti-inflammatory genes in ovine monocytes and neutrophils. However, there are some small differences regarding the extent of this modulation and more importantly a differential modulation in TGFB1 expression.
The reduction of NOS2 expression that is observed in neutrophils (to a greater extent) from both ovine and bovine WSE and from ovine WSE in monocytes is clearly an anti-inflammatory effect, as iNOS has been reported to be upregulated in macrophages in vivo, especially in disease states and chronic inflammatory processes (Kobzik, Citation2009), whereas it has been suggested that iNOS-derived NO enhances neutrophil infiltration (Sparkman & Boggaram, Citation2004).
The induction of PTGS2 expression in both cell types when treated with WSE derived either from ovine or bovine yoghurt is a more puzzling finding. Although the pro-inflammatory properties of individual PGs during the acute inflammatory response are well established, their role in the resolution of inflammation is more controversial. Monocytes-/macrophage-derived PGs have been implicated in down-regulating the expression of inflammatory cytokines (Aoki & Narumiya, Citation2012; James, Penglis, Caughey, Demasi, & Cleland, Citation2001) and in the self-limitation of the inflammatory response (Takayama et al., Citation2002). It seems, therefore, that COX-2 contributes to both phases of inflammation, in the onset of inflammation with the production of pro-inflammatory PGs and in the resolution by the production of anti-inflammatory PGs. Based on the other findings of the present study, it is logical to assume that the induction observed in WSE-treated immunocompetent cells is an anti-inflammatory effect.
This assumption is further supported by the results obtained on the gene expression of pro-inflammatory cytokines such as IL-1β and IL-12. The expression of IL-1β a pro-inflammatory cytokine that exerts pleiotrophic effects on a variety of cells and plays key roles in acute and chronic inflammation (Dinarello, Citation1998; Keyel, Citation2014) is significantly lower in neutrophils following treatment with both types of WSE (ovine and bovine), while no significant changes are observed in monocytes. IL-12 major functions include induction of IFN-γ production, enhancement of cytotoxicity of NK and cytotoxic T cells, and differentiation of naive T cells into Th1 effectors, suggesting a key role for IL-12 in the development of cell-mediated immunity (Denkers et al., Citation2003; Gately et al., Citation1998; Ma & Trinchieri, Citation2001). The observed down-regulation of IL12B in monocytes by both ovine and bovine WSE and in neutrophils by ovine WSE serves as further indication that bioactive peptides derived from ovine and bovine milk have anti-inflammatory properties. The third pro-inflammatory cytokine used in the present study, IFNG, did not differ significantly between experimental groups in monocytes or neutrophils.
Expression of the anti-inflammatory cytokines used in the present study shows at first glance a mixed effect for the two immunocompetent cell types. The reduction of IL1RN expression observed in neutrophils by both ovine and bovine WSE and in monocytes by ovine WSE ranges from 38% to 72%. However, this reduction seems insignificant when taking into account that IL-1ra is produced by monocytes and neutrophils and is released into the systemic circulation in >100-fold excess than either IL-1α or IL-1β after lipopolysaccharide stimulation (Dinarello, Citation1998; Malyak, Smith, Abel, & Arend, Citation1994). Furthermore, the fact that IL10 expression is not affected by treatment with WSE in monocytes and neutrophils merely implies a possible IL-10 independent mechanism of down-regulation of inflammation. Finally, the most confusing observation lies in the differential modulation of TGFB1 which is thought to have mainly an anti-inflammatory role. That is indeed true for monocytes (Braesch-Andersen, Paulie, Smedman, Mia, & Kumagai-Braesch, Citation2013) and our results agree with that as there is up-regulation of TGFB1 following treatment with both ovine and bovine WSE. Furthermore, Eligini, Barbieri, Arenaz, Tremoli, and Colli (Citation2007) report a TGF-β1-mediated induction of COX-2 in monocytes which agrees with our data that show an upregulation of both PTGS2 and TGFB1 in monocytes. In the case of neutrophils, however, Csernok et al. (Citation1996) reported that TGF-β might serve as a pro-inflammatory factor in neutrophil populations. Although there are no more studies that concur with this observation, our own findings regarding the down-regulation of TGFB1in neutrophils following treatment with bovine and ovine WSE taken together with the findings that point to an anti-inflammatory effect of yoghurt peptides in neutrophils support their claims.
It should be noted that some differences exist in the effect of the WSE depending on the yoghurt's milk origin. The most important of those in our opinion are the differences that are observed in the expression of NOS2 in monocytes and of IL12B in neutrophils where only the ovine WSE exhibits an anti-inflammatory effect. One could argue that this could mean a more pronounced anti-inflammatory effect from ovine-derived peptides; however, we think that these differences are not so striking, and therefore further experiments should be conducted in order to verify this. The limitation of the present study lies in the fact that the yoghurt samples were commercial, and therefore there was no control on the manufacturing process and the starter cultures used.
5. Conclusions
In conclusion, the present study shows that bioactive peptides derived from both ovine and bovine traditional Greek yoghurt possess mainly anti-inflammatory properties. More studies are required in order to identify the peptides that confer this property and to elucidate the mechanism that controls this process.
Disclosure statement
No potential conflict of interest was reported by the authors.
Notes on contributors
Ioannis Politis is a Ph.D. in Animal Science. Presently, he holds a Professor position in Nutritional and Lactation Physiology in the department of Animal Science and Aquaculture of the Agricultural University of Athens in Greece. His research interests include bioactive food ingredients and immunology.
Georgios Theodorou is a biochemist with a Ph.D. in Animal Science, he currently works as a post-doctoral researcher in the department of Animal Science and Aquaculture of the Agricultural University of Athens in Greece. His research interests include farm animal immunology, transcriptomics and epigenetics.
Additional information
Funding
References
- Adolfsson, O., Meydani, S. N., & Russell, R. M. (2004). Yogurt and gut function. American Journal of Clinical Nutrition, 80(2), 245–256.
- AOAC. (1990). Official methods of analysis. Arlington: AOAC International.
- Aoki, T., & Narumiya, S. (2012). Prostaglandins and chronic inflammation. Trends in Pharmacological Sciences, 33(6), 304–311. doi: 10.1016/j.tips.2012.02.004
- Baldi, A., Politis, I., Pecorini, C., Fusi, E., Chronopoulou, R., & Dell'Orto, V. (2005). Biological effects of milk proteins and their peptides with emphasis on those related to the gastrointestinal ecosystem. Journal of Dairy Research, 72, 66–72. doi: 10.1017/S002202990500110X
- Bogdan, C. (2001). Nitric oxide and the immune response. Nature Immunology, 2(10), 907–916. doi: 10.1038/ni1001-907
- Braesch-Andersen, S., Paulie, S., Smedman, C., Mia, S., & Kumagai-Braesch, M. (2013). ApoE production in human monocytes and its regulation by inflammatory cytokines. PLoS One, 8(11), e79908. doi:10.1371/journal.pone.0079908
- Couper, K. N., Blount, D. G., & Riley, E. M. (2008). IL-10: The master regulator of immunity to infection. The Journal of Immunology, 180(9), 5771–5777. doi: 10.4049/jimmunol.180.9.5771
- Csernok, E., Szymkowiak, C. H., Mistry, N., Daha, M. R., Gross, W. L., & Kekow, J. (1996). Transforming growth factor-beta (TGF-beta) expression and interaction with proteinase 3 (PR3) in anti-neutrophil cytoplasmic antibody (ANCA)-associated vasculitis. Clinical and Experimental Immunology, 105(1), 104–111. doi: 10.1046/j.1365-2249.1996.d01-715.x
- Denkers, E. Y., Del Rio, L., & Bennouna, S. (2003). Neutrophil production of IL-12 and other cytokines during microbial infection. Chemical Immunology and Allergy, 83, 95–114. doi: 10.1159/000071557
- Dinarello, C. A. (1997). Induction of interleukin-1 and interleukin-1 receptor antagonist. Seminars in Oncology, 24(3 Suppl 9), S9-81–S89-93.
- Dinarello, C. A. (1998). Interleukin-1, interleukin-1 receptors and interleukin-1 receptor antagonist. International Reviews of Immunology, 16(5–6), 457–499. doi: 10.3109/08830189809043005
- Dubois, R. N., Abramson, S. B., Crofford, L., Gupta, R. A., Simon, L. S., Van De Putte, L. B., & Lipsky, P. E. (1998). Cyclooxygenase in biology and disease. Faseb Journal, 12(12), 1063–1073.
- Eligini, S., Barbieri, S. S., Arenaz, I., Tremoli, E., & Colli, S. (2007). Paracrine up-regulation of monocyte cyclooxygenase-2 by platelets: Role of transforming growth factor-beta1. Cardiovascular Research, 74(2), 270–278. doi: 10.1016/j.cardiores.2006.12.013
- Fukao, T., Frucht, D. M., Yap, G., Gadina, M., O'Shea, J. J., & Koyasu, S. (2001). Inducible expression of Stat4 in dendritic cells and macrophages and its critical role in innate and adaptive immune responses. The Journal of Immunology, 166(7), 4446–4455. doi: 10.4049/jimmunol.166.7.4446
- Gately, M. K., Renzetti, L. M., Magram, J., Stern, A. S., Adorini, L., Gubler, U., & Presky, D. H. (1998). The interleukin-12/interleukin-12-receptor system: Role in normal and pathologic immune responses. Annual Review of Immunology, 16, 495–521. doi: 10.1146/annurev.immunol.16.1.495
- Geissmann, F., Auffray, C., Palframan, R., Wirrig, C., Ciocca, A., Campisi, L., … Lauvau, G. (2008). Blood monocytes: Distinct subsets, how they relate to dendritic cells, and their possible roles in the regulation of T-cell responses. Immunology and Cell Biology, 86(5), 398–408. doi: 10.1038/icb.2008.19
- Hellemans, J., Mortier, G., De Paepe, A., Speleman, F., & Vandesompele, J. (2007). qBase relative quantification framework and software for management and automated analysis of real-time quantitative PCR data. Genome Biology, 8(2), R19. doi: 10.1186/gb-2007-8-2-r19
- James, M. J., Penglis, P. S., Caughey, G. E., Demasi, M., & Cleland, L. G. (2001). Eicosanoid production by human monocytes: Does COX-2 contribute to a self-limiting inflammatory response? Inflammation Research, 50(5), 249–253. doi: 10.1007/s000110050750
- Keyel, P. A. (2014). How is inflammation initiated? Individual influences of IL-1, IL-18 and HMGB1. Cytokine, 69(1), 136–145. doi: 10.1016/j.cyto.2014.03.007
- Kobayashi, Y. (2010). The regulatory role of nitric oxide in proinflammatory cytokine expression during the induction and resolution of inflammation. Journal of Leukocyte Biology, 88(6), 1157–1162. doi: 10.1189/jlb.0310149
- Kobzik, L. (2009). Translating NO biology into clinical advances: Still searching for the right dictionary? American Journal of Respiratory Cell and Molecular Biology, 41(1), 9–13. doi: 10.1165/rcmb.2009-0156TR
- Korhonen, H., & Pihlanto, A. (2007). Technological options for the production of health-promoting proteins and peptides derived from milk and colostrum. Current Pharmaceutical Design, 13(8), 829–843. doi: 10.2174/138161207780363112
- Kuchroo, C. N., & Fox, P. F. (1982). Soluble nitrogen in Cheddar cheese: Comparison of extraction procedures. Milchwissenschaft, 37(6), 331–335.
- Law, J., & Haandrikman, A. (1997). Proteolytic enzymes of lactic acid bacteria. International Dairy Journal, 7(1), 1–11. doi: 10.1016/0958-6946(95)00073-9
- Li, M. O., & Flavell, R. A. (2008a). Contextual regulation of inflammation: A duet by transforming growth factor-beta and interleukin-10. Immunity, 28(4), 468–476. doi: 10.1016/j.immuni.2008.03.003
- Li, M. O., & Flavell, R. A. (2008b). TGF-beta: A master of all T cell trades. Cell, 134(3), 392–404. doi: 10.1016/j.cell.2008.07.025
- Li, M. O., Wan, Y. Y., Sanjabi, S., Robertson, A. K., & Flavell, R. A. (2006). Transforming growth factor-beta regulation of immune responses. Annual Review of Immunology, 24, 99–146. doi: 10.1146/annurev.immunol.24.021605.090737
- Ma, X., & Trinchieri, G. (2001). Regulation of interleukin-12 production in antigen-presenting cells. Advances in Immunology, 79, 55–92. doi: 10.1016/S0065-2776(01)79002-5
- Malyak, M., Smith Jr., M. F., Abel, A. A., & Arend, W. P. (1994). Peripheral blood neutrophil production of interleukin-1 receptor antagonist and interleukin-1 beta. Journal of Clinical Immunology, 14(1), 20–30. doi: 10.1007/BF01541172
- Marshall, O. J. (2004). PerlPrimer: Cross-platform, graphical primer design for standard, bisulphite and real-time PCR. Bioinformatics, 20(15), 2471–2472. doi: 10.1093/bioinformatics/bth254
- Moore, K. W., de Waal Malefyt, R., Coffman, R. L., & O'Garra, A. (2001). Interleukin-10 and the interleukin-10 receptor. Annual Review of Immunology, 19, 683–765. doi: 10.1146/annurev.immunol.19.1.683
- Munder, M., Mallo, M., Eichmann, K., & Modolell, M. (1998). Murine macrophages secrete interferon gamma upon combined stimulation with interleukin (IL)-12 and IL-18: A novel pathway of autocrine macrophage activation. Journal of Experimental Medicine, 187(12), 2103–2108. doi: 10.1084/jem.187.12.2103
- Munder, M., Mallo, M., Eichmann, K., & Modolell, M. (2001). Direct stimulation of macrophages by IL-12 and IL-18 – a bridge built on solid ground. Immunology Letters, 75(2), 159–160. doi: 10.1016/S0165-2478(00)00288-1
- O'Garra, A., Barrat, F. J., Castro, A. G., Vicari, A., & Hawrylowicz, C. (2008). Strategies for use of IL-10 or its antagonists in human disease. Immunological Reviews, 223, 114–131. doi: 10.1111/j.1600-065X.2008.00635.x
- Pfaffl, M. W. (2001). A new mathematical model for relative quantification in real-time RT-PCR. Nucleic Acids Research, 29(9), 2002–2007, e45. doi: 10.1093/nar/29.9.e45
- Politis, I., & Chronopoulou, R. (2008). Milk peptides and immune response in the neonate. Advances in Experimental Medicine and Biology, 606, 253–269. doi: 10.1007/978-0-387-74087-4_10
- Politis, I., Theodorou, G., Lampidonis, A. D., Chronopoulou, R., & Baldi, A. (2012). Soya protein hydrolysates modify the expression of various pro-inflammatory genes induced by fatty acids in ovine phagocytes. British Journal of Nutrition, 108(7), 1246–1255. doi: 10.1017/S0007114511006520
- Sabat, R., Grutz, G., Warszawska, K., Kirsch, S., Witte, E., Wolk, K., & Geginat, J. (2010). Biology of interleukin-10. Cytokine Growth Factor Reviews, 21(5), 331–344. doi: 10.1016/j.cytogfr.2010.09.002
- Schindler, H., Lutz, M. B., Rollinghoff, M., & Bogdan, C. (2001). The production of IFN-gamma by IL-12/IL-18-activated macrophages requires STAT4 signaling and is inhibited by IL-4. The Journal of Immunology, 166(5), 3075–3082. doi: 10.4049/jimmunol.166.5.3075
- Serbina, N. V., Jia, T., Hohl, T. M., & Pamer, E. G. (2008). Monocyte-mediated defense against microbial pathogens. Annual Review of Immunology, 26, 421–452. doi: 10.1146/annurev.immunol.26.021607.090326
- Sims, J. E., Gayle, M. A., Slack, J. L., Alderson, M. R., Bird, T. A., Giri, J. G., … Shanebeck, K. (1993). Interleukin 1 signaling occurs exclusively via the type I receptor. Proceedings of the National Academy of Sciences, 90(13), 6155–6159. doi: 10.1073/pnas.90.13.6155
- Sparkman, L., & Boggaram, V. (2004). Nitric oxide increases IL-8 gene transcription and mRNA stability to enhance IL-8 gene expression in lung epithelial cells. AJP: Lung Cellular and Molecular Physiology, 287(4), L764–L773.
- Takayama, K., Garcia-Cardena, G., Sukhova, G. K., Comander, J., Gimbrone Jr., M. A., & Libby, P. (2002). Prostaglandin E2 suppresses chemokine production in human macrophages through the EP4 receptor. Journal of Biological Chemistry, 277(46), 44147–44154. doi: 10.1074/jbc.M204810200
- Tilley, S. L., Coffman, T. M., & Koller, B. H. (2001). Mixed messages: Modulation of inflammation and immune responses by prostaglandins and thromboxanes. Journal of Clinical Investigation, 108(1), 15–23. doi: 10.1172/JCI200113416