ABSTRACT
The biological features of porcine alveolar macrophages (PAMs) and interstitial macrophages (IMs) were investigated, including morphology, nitric oxide (NO) secretion, cell viability and porcine reproductive and respiratory syndrome virus (PRRSV) mRNA expression post-inoculation with TJ-F10 or TJM-F92. Viability and NO secretion of PAMs and IMs were examined by 3-(4,5-dimethylthiazol-2-yl)-2,5-diphenyltetrazolium bromide assay and Griess's assay, respectively. mRNA expression of PRRSV, inducible nitric oxide synthase (iNOS) and Arginase1 (Arg1) in PAMs and IMs were detected by quantitative real-time polymerase chain reaction technique. Our results show that PAMs were bigger and more granular than IMs and the Arg1/iNOS value was much higher in PAMs than in IMs. In addition, the vaccine strain TJM-F92 evoked higher NO production in PAMs and IMs compared with the wild type strain TJ-F10. In conclusion, our results indicate that the PAMs and IMs are heterogeneous in morphology, NO production and susceptibility to PRRSV.
Introduction
Porcine reproductive and respiratory syndrome (PRRS) has been one of the most economically important diseases affecting swine industry worldwide and causes great economic losses each year. The major characteristics of PRRS include reproductive failure and respiratory disease that usually leads to compromised lung defense, and results in secondary complications, such as persistent infections, antibody-dependent enhancement, interstitial pneumonia and immunosuppression. The porcine reproductive and respiratory syndrome virus (PRRSV) replicates mainly in porcine alveolar macrophages (PAMs) and dendritic cells, which could delay and down-modulate adaptive immune functions, leading to reduced vaccine efficacy (Wongyanin et al., Citation2012).
In 2006, a highly pathogenic PRRSV (HP-PRRSV) was reported as the main cause of the large-scale outbreak of PRRS with high mortality in China. The HP-PRRSV non-structural protein 2 was shown to have two discontinuous deletions, a 1 amino acid deletion at position 481 and a 29 amino acid deletion at positions 533–651 (Li et al., Citation2012). Epidemics of the atypical disease caused by this emergent HP-PRRSV have resulted in huge economic losses in the Chinese pig industry since 2006 (Tian et al., Citation2007). To develop a vaccine against HP-PRRS virus, the HP-PRRS virus strain TJ was attenuated by serial passages and plaque-cloned every 5–10 passages in Marc-145 cells. A new continuous 120 amino acid deletion after the discontinuous 30 amino acid deletions (sites 481 and 533–561) occurred in strain TJ passages, and the 120 amino acid deletion is between 628 and 747 (Leng-Xue et al., Citation2012). The genetic characteristics differ greatly from other current commercial HP-PRRSV vaccine strains in China, such as JXA1-R and HuN4-F114. In order to further evaluate the efficacy for preventing PRRS and to determine the molecular mechanisms of prevention, it is important to identify the biological features that are altered in this genetically varied vaccine strain.
Since the emergence of PRRS in the late 1980s, extensive research has been conducted on PRRS etiology, epidemiology, pathogenic mechanisms, diagnosis and prevention. The pathologic changes in pigs infected with PRRSV include dramatically reduced macrophage numbers that could lead to secondary infection, mixed infection and persistent infection (Labarque, Van Gucht, Nauwynck, Van Reeth, & Pensaert, Citation2003; Rodríguez-Gómez et al., Citation2014). PRRSV causes only minimal lung inflammation (Pol, van Dijk, Wensvoort, & Terpstra, Citation1991) and neutrophil infiltration (Labarque, Nauwynck, Van Reeth, & Pensaert, Citation2000). These findings suggest the involvement of apoptotic processes, since apoptotic bodies could be phagocytized by resident macrophages without provoking a dominant inflammatory response. Nevertheless, the phenomenon of reduced PAMs alone cannot fully explain interstitial pneumonia characterized by excessive macrophage infiltration into lesion areas. It is known that IM cells also play a critical role in shaping the outcome of lung inflammation after infection. We hypothesized that the heterogeneity of PAM and IM cells may lead to divergent effects on the occurrence, development and recovery in PRRS, and the infection of the PRRSV vaccine strain TJM-F92 may have a different mechanism from the PRRSV wild strain infection. We, therefore, investigated the biological changes in PAMs and IMs post-inoculation in vitro with the wild type strain TJ-F10 and the vaccine strain TJM-F92, in an effort to explore the potential heterogeneity of lung macrophages. We undertook this study as an important opportunity to advance the understanding of the function of macrophages in pathogenesis and recovery from PRRS and development of new vaccine for PRRS.
Materials and methods
Virus
PRRSV wild type strain TJ-F10 was kindly provided by Dr Hua Wu from Sinovet (Beijing) Biotechnology Co., Ltd., Beijing, China. A stock of the virus was the 10th passage cell culture prepared in Marc-145 cells with a titer of 108 TCID50/mL. Marc-145 cells were grown in Dulbecco's modified Eagle's medium (DMEM; Invitrogen) supplemented with 10% fetal bovine serum (FBS; Invitrogen), 100 U/mL Penicillin G, 100 mg/mL Na streptomycin sulfate, and 2 mM l-glutamine. Commercial PRRSV vaccine strain TJM-F92 was an attenuated live PRRSV purchased from Shanxi Luckier Co. Ltd., Jinzhong, Shanxi, China.
Animals
Three- to four-week-old weaned pigs (conventional Large White-Duroc crossbred) were procured from a swine herd seronegative for PRRSV, porcine respiratory coronavirus, transmissible gastroenteritis virus and porcine circovirus 2 virus.
Collection of PAM
Pigs were euthanized by exsanguination. The trachea was ligated to prevent total pulmonary collapse, and the heart and lungs were removed from the thorax. Alveolar macrophages were collected from lungs by bronchioalveolar lavage. Analysis by flow cytometry has demonstrated that bronchial alveolar lavage fluid (BALF) preparations are almost exclusively composed of PAMs (Ait-Ali et al., Citation2007). The PAMs were re-suspended in complete RPMI-1640 medium (10% FBS, 2 mM l-glutamine, 1 µg/mL fungizone, 100 U/mL penicillin and 100 µg/mL streptomycin). PAMs were incubated in Petri dishes for 2 h at 37°C in a humidified 5% CO2 atmosphere. The medium and non-adherent cells were removed by aspiration and the adherent cells were washed once with complete RPMI-1640 medium. The adherent cells were digested by trypsin, washed three times, counted, and scored for viability by trypan blue dye exclusion. The cells were plated at a density of 1 × 105 cells/well in 96-well culture plates or 1 × 106 cells/well in 6-well culture plates.
Isolation of IMs
The lung tissue was chopped into pieces of less than 1 mm3 using a scissor. To remove remaining PAMs and blood cells, the tissue was washed with phosphate-buffered saline over a 100 μm cell strainer until the filtrate appeared to be clear. The tissue was then digested using 0.025% collagenase IA (Sigma) for 60 min at 37°C in a shaking water bath. The digestion was filtrated by 200 mesh stainless steel filter, centrifuged at 1500 rpm for 10 min and re-suspended in RPMI 1640 culture medium with 10% FBS in Petri dishes (Hoppstädter et al., Citation2010). These cells were allowed to adhere to Petri dishes, collected, washed, counted, scored for viability and plated as PAMs.
Virus inoculation
PAMs and IMs were inoculated with PRRSV at a multiplicity of infection (MOI) of 0.2, 1, 5 virions/cell, respectively. After 1 h of incubation at 37°C and 5% CO2, the inoculum was removed and the cells were washed with RPMI-1640 before further culture for 24 h for viral mRNA quantification and nitric oxide (NO) and MTT assay.
RNA extraction and quantitative real-time polymerase chain (PCR) reaction
Total RNA was prepared using Trizol reagent (Takara, China) according to the manufacturer's instructions. cDNA was then synthesized using an oligo(dT)15 primer and SuperScript™ II reverse transcriptase reagents (Takara, China). Quantitative real-time PCR was performed on a CFX96 Optics Module instrument (Bio-Rad Laboratories, Hercules, CA, USA) with the iTaq™ Universal SYBR GREEN Supermix according to the manufacturer's recommendations. The following forward and reverse primer sequences were used: hypoxanthine phosphoribosyltransferase (HPRT), For 5′-GAGCTACTGTAATGACCAGTCAACG-3′ and Rev 5′-CCAGTGTCAATTATATCTTCAACAATCAA-3′; PRRSV, For 5′-CAATCTTCCGACACCATCCGAG-3′ and Rev 5′-TGTGAGGACGCAGACAAATCCAGAG-3′; Arginase1, For 5′-CCAGTCCATGGAGGTCTGTC-3′ and Rev 5′-GTGTCTTCCCCAGAGATGGA-3′; iNOS, For 5′-CAGCAAAGAAATCTCCAGACTCC-3′ and Rev 5′-CCTGGGTCCTATGGTCAAACTT-3′; The thermal cycling conditions were as follows: 3 min at 95°C followed by 35 cycles of denaturation at 95°C for 5 s, annealing at 55°C for 10 s, and extension at 72°C for 30 s. The fluorescence signal was detected at the end of each cycle. The results were analyzed with the CFX96 manager software supplied with the machine, and a melting curve and electrophoresis was used to confirm the specificities of the products. The expression levels of PRRSV were normalized to the internal control HPRT.
NO quantitation
The PAM and IM cells were plated at a density of 1 × 105 cells/well in 96-well plates and then were inoculated with PRRSV (TJ-F10 or vaccine strain) at a MOI of 1, 3 and 7 virions/cell. After 18 h of incubation at 37°C and 5% CO2, culture media were collected for the NO assays. Nitric oxide concentration was measured by detection of its stable oxidative metabolite, nitrite. In brief, 80 μL of the culture media were mixed with an equal volume of Griess reagent (0.1% naphthylethylenediamine dihydrochloride and 1% sulfanilamide in 5% phosphoric acid) and then shaken for 5 min at room temperature. The absorbance at 550 nm was measured, and the nitrite concentration was determined using a calibration curve constructed using sodium nitrite standard (Su et al., Citation2011).
Cell viability
The viability of the cells was evaluated by the reduction of yellow MTT (3-[4,5-dimethylthiazol-2-yl]-2,5-diphenyl tetrazolium bromide) to purple formazan. The PAM and IM cells (1 × 105/well) were treated with PRRSV as described above. After removal of the media, cells in each well were incubated with MTT in phenol red-free RPMI 1640 media for 3.5 h according to the manufacturer's protocol. The assay was stopped by adding MTT solubilization solution (10% Triton X-100, 0.1 N HCl in anhydrous isopropanol). The plates were stored overnight at 37°C to completely dissolve formazan crystals. Formazan was quantified using a Bio-Rad iMark™ microplate reader (Bio-Rad) by monitoring the absorbance at 570 nm (Wang et al., Citation2009).
Statistical analysis
Data are represented as mean ± SD when indicated and Student's t-test was used for all statistical analyses with the Prism 5.0 software (GraphPad Software, Inc., San Diego, CA, USA). Differences were considered significant when the p value was less than .05.
Results
Morphology of PAMs and IMs
The morphologies of PAMs and IMs were observed by a light microscopy. PAM cells were bigger and more granular than IM cells ().
Polarization-related gene expression
Arginase1 (Arg1) and inducible nitric oxide synthase (iNOS) are phenotype markers of differently polarized macrophage pro-inflammatory cells (M1) and anti-inflammatory cells (M2), respectively. The gene expression of both Arg1 and iNOS was measured. The Arg1/iNOS value was calculated by dividing the relative mRNA expression level of Arg1 by that of iNOS ().
NO secretion
Dynamic changes of NO levels secreted by PAMs inoculated with different doses of wild type or vaccine type PRRSV (MOI = 1, 3 and 7) were investigated. The results showed that the NO levels were similar for the PAMs inoculated with different MOI of field strain TJ-F10. However, the NO levels in the vaccine strain TJM-F92-treated PAMs were elevated in a dose-dependent manner ((a)).
Figure 3. Dynamic changes of the NO levels in PAMs (a) and IMs (b) inoculated with different doses of the wild type (TJ-F10) or the vaccine type PRRSV (TJM-F92) (MOI = 1, 3 and 7). **p < .01.
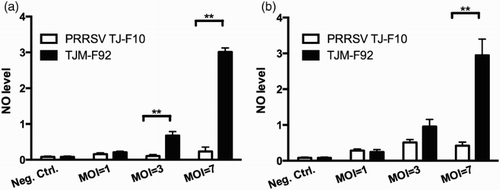
Similar experiments were conducted with IMs. The NO levels were similar for the IMs treated with different MOI of field strain TJ-F10; As with PAMs, the NO levels in IMs treated with the vaccine strain TJM-F92 were elevated in a dose-dependent manner ((b)).
Cell viability
Dynamic changes of PAM and IM viability inoculated with different doses of wild type or vaccine type PRRSV (MOI = 1, 3 and 7) were investigated by MTT assay. The results showed that the viability of both PAMs and IMs treated with the vaccine strain (TJM-F92) was higher than that of the cells treated with the field strain (TJ-F10) ((a) and 4(b)).
Virus quantitation
Relative expression of PRRSV in PAMs and IMs was detected for both the wild type and the vaccine strain by real-time PCR technique. The PAMs and IMs were inoculated by the virus strains at MOI of 0.2, 1 and 5, respectively. The expression levels of PRRSV were normalized to the internal control HPRT. The results showed that the PRRSV wild strain TJ-F10 mRNA expression in PAMs was much higher at MOI of 5, compared with its expression in IMs ((a)). Similar results were observed by the vaccine strain (TJM-F92) ((b)).
Discussion
PRRSV, as a positive-sense RNA virus, initiates infection in PAMs. PRRSV is known to elicit weak immune responses, and establish a persistent infection. It causes a disease characterized by reproductive failure, including early stillbirth and late-term abortion, as well as respiratory distress in piglets and influenza-like disease in growing and finishing swine. The molecular mechanisms of PRRSV infection are still poorly understood (Xiao et al., Citation2010).
Extensive studies on the macrophage cells in human and mice indicate that the macrophages are heterogeneous in these species (Kyrova, Stepanova, Rychlik, Faldyna, & Volf, Citation2012). Macrophages can be divided into classically activated inflammatory macrophages (M1) and alternatively activated anti-inflammatory macrophages (M2). It has been generally accepted that M1 macrophages are polarized into an inflammatory environment to produce pro-inflammatory cytokines, while M2 macrophages are involved in anti-inflammation and aid tissue repair in wound healing (Kyrova et al., Citation2012). Nevertheless, the heterogeneity of macrophages in pigs is less understood. There are mainly two types of macrophages in pigs: PAMs and IMs. Our study showed that, morphologically, PAMs were bigger and more granular than IMs. We measured the NO levels in PAMs and IMs to determine their polarization. NO is an important early signal in many pathophysiological processes (Miller et al., Citation2010). The NO is known to inhibit viral protein and/or RNA synthesis by modification of target molecules essential for viral replication, such as ribonucleotide reductase and viral protease (Miller, Lager, & Kehrli, Citation2009). Arg1 acts on l-Arg, the same substrate of iNOS, to produce l-ornithine (l-Orn) and urea, precursors of polyamines and collagen. The competition for the same substrate acts as a way to control the concentration of NO (Nelson, Lei, & Prabhu, Citation2011). The relative level of Arg1toiNOS, which is frequently used as index of the M2 versus M1 functional macrophage phenotype (Chiang et al., Citation2008). In general, M1s or classically activated macrophages are pro-inflammatory and microbicidal, whereas M2s or alternatively activated macrophages are immunomodulatory, reparative, and poorly microbicidal. The balance of these two phenotypes plays a critical role in the phagocytosis of pathogens, the clearance of apoptotic cells, and the remodeling of injured tissues. The actual macrophage phenotype is viewed as a continuum of functional states between these two opposing ends (Garg, Pullen, Oskeritzian, Ryan, & Bowlin, Citation2013). Based on our data in , the Arg1/iNOS value in PAMs and IMs differs significantly, indicating the heterogeneity of different macrophages in the porcine lung.
In , we show that the NO levels in PAMs and IMS had no significant difference when treated with different MOI of PRRSV field strain TJ-F10, indicating that although PRRSV induces severe apoptotic death of PAMs in lungs, it does not influence the NO level in the respiratory tract. NO might not play a critical role in the pathogenesis of PRRSV (Jung, Gurnani, Renukaradhya, & Saif, Citation2010). Surprisingly, when the PAMs and IMs treated with the vaccine strain TJM-F92, the NO levels were increased in a virus dose-dependent manner. Furthermore, the vaccine strain increased the viability of PAMs and IMs compared with the wild type strain TJ-F10 (), indicating the wild type and the vaccine type virus act through different mechanisms.
We next determined whether macrophage infection by the PRRSV was similar in the vaccine and the wild type strain. The data in showed the mRNA level of the PRRSV is much higher in the PAMs (MOI = 5) than in the IMs (p < .01). Compared with the mRNA level of PRRSV field strain TJ-F10 in PAMs, the level of the vaccine strain TJM-F92 was 10-fold lower (MOI = 5), which maybe related with the higher NO production evoked by the vaccine strain as shown in .
Vaccines for PRRS in China are predominately live attenuated products originated from the field strain, which produce an acute infection with viremia followed by a state of persistence as the field strain. Immunologically vaccine viruses are the same as virulent viruses, expressing the same antigens in the same way. Vaccinated animals harbor virus persistent in the lymphoid tissues and transmit the virus to other pigs, as evidenced by re-isolation of the vaccine strains from commercial herds. New types of vaccine need to be developed for prevention of PRRS to immunize the pig in a different way from that by the field strain. In this work, we found the vaccine strain TJM-F92 is different from its original strain TJ-F10 in NO secretion, macrophage viability and virus mRNA expression in PAMs and IMs.
In summary, our study showed the PAMs and IMs are heterogeneous in morphology, NO production and susceptibility to PRRSV. PAMs were larger and more granular than IMs. Interestingly, the PRRSV vaccine strain TJM-F92 produced viability and evoked higher NO production in PAMs compared with the wild type strain TJ-F10. Whether these effects are essential for preventing PRRS remains to be determined.
Disclosure statement
No potential conflict of interest was reported by the authors.
Notes on contributor
Yaping Hu is a graduate student in the College of Life Science and Technology, Dalian University, China. She is working on her Master thesis.
Meichen Wang is a graduate student in the Department of Veterinary Physiology and Pharmacology, Texas A&M University.
Xuemei Zhang is a graduate student in the College of Life Science and Technology, Dalian University. She is working on her Master thesis.
Peng Wang is a graduate student in the College of Life Science and Technology, Dalian University. He is working on his Master thesis.
Huan Liu is an undergraduate student in the College of Life Science and Technology, Dalian University. He is currently conducting his undergraduate research project.
Dr Qinfu Wang is a professor of immunology in the Institute of Immunology, Dalian University. Dr Wang’s current research interests focus on the mechanism and prevention and control of key animal infectious diseases, including porcine reproductive and respiratory syndrome (PRRS), classical swine fever (CSF), foot and mouth disease (FMD), etc.
ORCID
Qinfu Wang http://orcid.org/0000-0001-8155-8345
Additional information
Funding
References
- Ait-Ali, T., Wilson, A. D., Westcott, D. G., Clapperton, M., Waterfall, M., Mellencamp, M. A., … Archibald, A. L. (2007). Innate immune responses to replication of porcine reproductive and respiratory syndrome virus in isolated Swine alveolar macrophages. Viral Immunology, 20(1), 105–118. doi:10.1089/vim.2006.0078
- Chiang, C.-S., Chen, F.-H., Hong, J.-H., Jiang, P.-S., Huang, H.-L., Wang, C.-C., … McBride, W. H. (2008). Functional phenotype of macrophages depends on assay procedures. International Immunology, 20(2), 215–222. doi:10.1093/intimm/dxm137
- Garg, K., Pullen, N. A., Oskeritzian, C. A., Ryan, J. J., & Bowlin, G. L. (2013). Macrophage functional polarization (M1/M2) in response to varying fiber and pore dimensions of electrospun scaffolds. Biomaterials, 34(18), 4439–4451. doi:10.1016/j.biomaterials.2013.02.065
- Hoppstädter, J., Diesel, B., Zarbock, R., Breinig, T., Monz, D., Koch, M., … Kiemer, A. K. (2010). Differential cell reaction upon Toll-like receptor 4 and 9 activation in human alveolar and lung interstitial macrophages. Respiratory Research, 11, 124. doi:10.1186/1465-9921-11-124
- Jung, K., Gurnani, A., Renukaradhya, G. J., & Saif, L. J. (2010). Nitric oxide is elicited and inhibits viral replication in pigs infected with porcine respiratory coronavirus but not porcine reproductive and respiratory syndrome virus. Veterinary Immunology and Immunopathology, 136(3–4), 335–339. doi:10.1016/j.vetimm.2010.03.022
- Kyrova, K., Stepanova, H., Rychlik, I., Faldyna, M., & Volf, J. (2012). SPI-1 encoded genes of Salmonella Typhimurium influence differential polarization of porcine alveolar macrophages in vitro. BMC Veterinary Research, 8, 115. doi:10.1186/1746-6148-8-115
- Labarque, G. G., Nauwynck, H. J., Van Reeth, K., & Pensaert, M. B. (2000). Effect of cellular changes and onset of humoral immunity on the replication of porcine reproductive and respiratory syndrome virus in the lungs of pigs. The Journal of General Virology, 81(Pt 5), 1327–1334. doi:10.1099/0022-1317-81-5-1327
- Labarque, G., Van Gucht, S., Nauwynck, H., Van Reeth, K., & Pensaert, M. (2003). Apoptosis in the lungs of pigs infected with porcine reproductive and respiratory syndrome virus and associations with the production of apoptogenic cytokines. Veterinary Research, 34(3), 249–260. doi:10.1051/vetres:2003001
- Leng-Xue, N., Li, Z.-G., Li, X.-Y., Wang, F.-X., Zhang, X.-H., & Wu-Hua, N. (2012). [Genetic variation and pathogenicity analysis of highly pathogenic porcine reproductive and respiratory syndrome virus strain TJ in the course of attenuation]. Bing Du Xue Bao = Chinese Journal of Virology/[bian Ji, Bing Du Xue Bao Bian Ji Wei Yuan Hui], 28(2), 136–142.
- Li, Z., Leng, X., Qi, Q., Wang, F., Wen, Y., Tan, B., … Wu, H. (2012). Complete genome sequence of a highly pathogenic porcine reproductive and respiratory syndrome virus NM1 strain from northern China. Journal of Virology, 86(24), 13863–13864. doi:10.1128/JVI.02642-12
- Miller, L. C., Lager, K. M., & Kehrli, M. E. (2009). Role of Toll-like receptors in activation of porcine alveolar macrophages by porcine reproductive and respiratory syndrome virus. Clinical and Vaccine Immunology: CVI, 16(3), 360–365. doi:10.1128/CVI.00269-08
- Miller, L. C., Neill, J. D., Harhay, G. P., Lager, K. M., Laegreid, W. W., & Kehrli, M. E. (2010). In-depth global analysis of transcript abundance levels in porcine alveolar macrophages following infection with porcine reproductive and respiratory syndrome virus. Advances in Virology, 2010, 864181. doi:10.1155/2010/864181
- Nelson, S. M., Lei, X., & Prabhu, K. S. (2011). Selenium levels affect the IL-4-induced expression of alternative activation markers in murine macrophages. The Journal of Nutrition, 141(9), 1754–1761. doi:10.3945/jn.111.141176
- Pol, J. M., van Dijk, J. E., Wensvoort, G., & Terpstra, C. (1991). Pathological, ultrastructural, and immunohistochemical changes caused by Lelystad virus in experimentally induced infections of mystery swine disease (synonym: Porcine epidemic abortion and respiratory syndrome (PEARS)). The Veterinary Quarterly, 13(3), 137–143. doi:10.1080/01652176.1991.9694298
- Rodríguez-Gómez, I. M., Barranco, I., Amarilla, S. P., García-Nicolás, O., Salguero, F. J., Carrasco, L., & Gómez-Laguna, J. (2014). Activation of extrinsic- and Daxx-mediated pathways in lymphoid tissue of PRRSV-infected pigs. Veterinary Microbiology, 172(1–2), 186–194. doi:10.1016/j.vetmic.2014.05.025
- Su, Y.-W., Chiou, W.-F., Chao, S.-H., Lee, M.-H., Chen, C.-C., & Tsai, Y.-C. (2011). Ligustilide prevents LPS-induced iNOS expression in RAW 264.7 macrophages by preventing ROS production and down-regulating the MAPK, NF-κB and AP-1 signaling pathways. International Immunopharmacology, 11(9), 1166–1172. doi:10.1016/j.intimp.2011.03.014
- Tian, K., Yu, X., Zhao, T., Feng, Y., Cao, Z., Wang, C., … Gao, G. F. (2007). Emergence of fatal PRRSV variants: Unparalleled outbreaks of atypical PRRS in China and molecular dissection of the unique hallmark. PLoS ONE, 2(6), e526. doi:10.1371/journal.pone.0000526
- Wang, C., Muttil, P., Lu, D., Beltran-Torres, A. A., Garcia-Contreras, L., & Hickey, A. J. (2009). Screening for potential adjuvants administered by the pulmonary route for tuberculosis vaccines. The AAPS Journal, 11(1), 139–147. doi:10.1208/s12248-009-9089-0
- Wongyanin, P., Buranapraditkul, S., Yoo, D., Thanawongnuwech, R., Roth, J. A., & Suradhat, S. (2012). Role of porcine reproductive and respiratory syndrome virus nucleocapsid protein in induction of interleukin-10 and regulatory T-lymphocytes (Treg). The Journal of General Virology, 93(Pt 6), 1236–1246. doi:10.1099/vir.0.040287-0
- Xiao, S., Jia, J., Mo, D., Wang, Q., Qin, L., He, Z., … Chen, Y. (2010). Understanding PRRSV infection in porcine lung based on genome-wide transcriptome response identified by deep sequencing. PloS One, 5(6), e11377. doi:10.1371/journal.pone.0011377