ABSTRACT
The optimal conditions for preparing Zn-Flammulina velutipes polysaccharide (Zn-FVP) chelate were studied by using L9 (34) orthogonal test. The results showed that the optimized conditions for the chelating reaction were as follows: initial concentration of Zn (II), 6 mg/mL; ratio of purified polysaccharide (FVPp) to Zn (II), 1:2; chelating time, 8 h; and pH, 5. Chelating rate achieved 98.11% under the optimal conditions. Characteristic bands for OH stretching at 3000–3500 cm−1 and bands corresponding to C-H variable angle vibration between 1000–1100 cm−1 showed some obvious differences for chelation of polysaccharides and zinc. The qualitative spectrum of Zn-FVP indicated the presence of ZnSO4 and the abundant Zn (II) groups were widely present in the chelated samples. The anti-inflammatory effect of FVPp and Zn-FVP on Lipopolysaccharide-stimulated RAW 264.7 cells was evaluated. In the range of 5–50 μg/mL, Zn-FVP did not exhibit obvious inhibition of the survival rate of cells. Zn-FVP in noncytotoxic levels showed strong suppressive effect on the inflammatory cytokines (IL-6, TNF-α, INF-γ and NO) and these provided a theoretical base for the anti-inflammatory mechanism of Zn-FVP.
1. Introduction
Flammulina velutipes, one of the most popular edible mushrooms, is currently artificially cultivated on large-scale in China and Japan. F. velutipes has become popular due to its high nutritional value and delicious taste. Most studies have paid much attention on the active constituents and pharmacological properties of F. velutipes. Polysaccharides, one of the most important components of mushrooms, have exhibited extensive and powerful benefits applicable in developing novel drugs and functional foods, which are correlated to their immune-modulating, anti-oxidation, anti-tumor and nontoxic effects (He et al., Citation2016; Hou et al., Citation2013; Sang, Yong, Sang, & Jin, Citation2015; Zhang, Cui, Cheung, & Wang, Citation2007; Zhao, Xu, Ye, & Dong, Citation2013). Several polysaccharides have been isolated from F. velutipes fruiting bodies or mycelia, and their anti-oxidation, anti-tumor and immuno-regulatory effects were studied (Ikekawa et al., Citation1982; Miao, Wang, & Wu, Citation2003; Wu & Gao, Citation2002; Wu, Duan, Liu, & Cen, Citation2010; Yang, Fang, Liang, & Hu, Citation2011; Zhao, Dong, Chen, & Hu, Citation2010).
As one of the essential trace elements, zinc (Zn) can modulate cellular signal recognition, second messenger metabolism, protein kinase, and protein phosphatase activities (Beyersmann & Haase, Citation2001). Zn is well known to play an important role in immune system, and with Zn deficiency, animals have exhibited depressed immune function, frequent infections, dermatitis, diarrhea, alopecia, and mental disturbances (Walsh, Sandstead, Prasad, Newberne, & Fraker, Citation1994; Zalewski, Citation1996). For public health, particularly in recent years, Zn deficiency is becoming a major cause of morbidity (Caulfield, de Onis, Blossner, & Black, Citation2004; Caulfield, Richard, & Black, Citation2004). By source, Zn can be divided into two categories: organic zinc is bound to an organic ligand such as a polysaccharide or an amino acid chelate, amino acid complex and protein; inorganic zinc is generally bound to an inorganic salt such as chloride, sulfate, carbonate or oxide. Currently, zinc supplements are mostly inorganic and have lower absorbability than the organic one. Furthermore, it would be toxic to humans for excessive intake. Inorganic zinc can be converted into organic zinc by biotransformation, which has low toxicity, high absorbability and better biological potency (Li, Zhu, Zhang, Chen, & Song, Citation2011; Sibikina, Iozep, & Moskvin, Citation2009). Comparison of growth performance in weanling pigs fed diets supplemented with zinc-polysaccharide or zinc oxide was studied by Buff, Bollinger, Ellersieck, Brommelsiek, and Veum (Citation2005) and found that diets containing 300 or 450 ppm Zn as Zn-polysaccharides (Zn-PS) almost had the same growth performance in weanling pigs with that of pigs fed 2,000 ppm Zn as ZnO, but feeding 300 ppm Zn as Zn-PS decreased Zn excretion by 76% compared to feeding 2,000 ppm Zn as ZnO. The trace elements chelate was easily absorbed, especially when combining the trace elements with protein, polysaccharide, or other organic molecules. The trace elements chelate could also play a constructive role in physiological functions. What is more, the content of Zn was relatively high in F. velutipe, which was about 35% of the Zn combined with polysaccharide.
NO production, which is stimulated by LPS, has been demonstrated to contribute to septic shock (Jacobs & Ignarro, Citation2001). TNF-α, INF-γ, and IL-6 may induce necrosis, apoptosis, and activates several other cytokines. Studies have been carried out to investigate the effects of polysaccharides on expressions of pro-inflammatory and anti-inflammatory cytokine in lipopolysaccharide (LPS)-stimulated RAW264.7 cells. Sulfated polysaccharide from Ecklonia cava was shown to dose-dependently inhibit the expression levels of NO by LPS-stimulated RAW 264.7 macrophages (Kang et al., Citation2011). The addition of sulfated Astragalus polysaccharide also downregulated the mRNA expression of TNF-α in LPS-infected CaCO2 cells in a dose-dependent manner (Wang et al., Citation2013). This study proved that Zn-FVP significantly downregulated the expression of these inflammation indicators (P < .05), especially at the highest dose of 50 μg/mL.
Nowadays, there are many studies related to the antioxidant and anti-inflammatory activities of polysaccharides (Chen et al., Citation2014; Jiang, Citation2014; Kozarski et al., Citation2012; Zeng, Zhang, & Jia, Citation2014); however, few investigations have been carried out on the chelation of Zn with polysaccharides from edible mushroom. At the same time, reports about the bioactivities of the complex of Zn with F. velutipes purified polysaccharide (FVPp) are few. Therefore, Zn-Flammulina velutipes polysaccharide (Zn-FVP) was prepared and the anti-inflammatory activity of the polysaccharides before and after chelation was comparatively studied in this study. This study not only provides more evidence that Zn-FVP exerts anti-inflammatory activity in macrophage cells, but also shows the potential of Zn-FVP as a therapeutic drug. Zn and FVP are functional components of foods, and their synergy on the bioactivities is worth noting. Moreover, structural characteristics of FVPp and Zn-FVP were analyzed.
2. Materials and methods
2.1. Materials and reagents
F. velutipes was purchased from Jiangsu Tianfeng Biological Technology Co., Ltd. (Nanjing, China). The zinc (1000 μg/mL) standard solution was purchased from the national standard material center (China). DEAE Cellulose-52 and Sephadex G-100 were purchased from Whatman Co. (Maidstone, Kent, UK) and Pharmacia Co. (Sweden), respectively. The RAW264.7 cells were obtained from the CBCAS (Cell Bankof the Chinese Academic of Sciences, Shanghai, China). Dimethylsulfoxide (DMSO), LPS and 3-(4,5-dimethylthiazol -2-yl)-2,5-diphenyl tetrazolium bromide (MTT) were from Sigma Chemical Co.(St. Louis, MO, USA). Dulbecco’s minimal essential medium (DMEM), fetal bovine serum (FBS), penicillin and streptomycin were purchased from GIBCO (GrandIsland, NY, USA). Mouse IL-6, TNF-α, INF-γ, and NO ELISA kits were purchased from Jiancheng Biological Technology Co., Ltd. (Nanjing, China). All other reagents were of analytical grade.
2.2. Isolation and purification of F. velutipes polysaccharide
According to our previous study (Liu et al., Citation2015), F. velutipes polysaccharide (FVP) was separated as follows. Briefly: (1) fresh F. velutipes was dried at 60oC, powdered and sieved through 300 mesh; (2) the extraction was carried out under the optimized condition (ratio of water to dry material, 30 mL/g; temperature, 75°C; and time, 2 h); (3) crude polysaccharides extract was deproteinized with Sevag reagent and was centrifuged for 15 min at 5000 rpm, then it was concentrated into 50–100 mL by rotary evaporation; (4) a four-fold volume anhydrous ethanol was added to the solution to precipitate the polysaccharide and the precipitate was washed successively with anhydrous ethanol and acetone, dialyzed against deionized water, and lyophilized to gain crude polysaccharides (FVPc).
Crude polysaccharide was purified by DEAE Cellulose-52 ion chromatography and Sephadex G-100 gel chromatography according to our previous study (Yang et al., Citation2012; Zhao, Fu, Chen, Yang, & Hu, Citation2015). Three fractions (F1, F2, and F3) were obtained after the purification by DEAE-52 cellulose column (2.6 cm × 50 cm) with a stepwise elution by NaCl solution (0, 0.1, 0.3, and 0.5 M), and then the water-eluted fraction F1 was chosen for further purification with a Sephadex G-100 column (2.6 cm × 60 cm) and eluted with water at a flow rate of 0.25 mL/min. The purified fraction was lyophilized and named as water-soluble polysaccharide (FVPp).
2.3. Measurement of molecular weights
In order to determine the average molecular weights of the sample, the freeze-dried sample was introduced into TSK gel G4000 PWXL column. The eluent was H2O/N2 with a flow rate of 0.7 mL/min at 35°C. Before the sample analysis, Pullulan standards (20, 100, 200, 400, 800, and 1600 kDa) were also used for comparison. The retention time was plotted against average molecular weight of the Pullulan, and then the molecular weight of FVPp was calculated (Yang et al., Citation2012).
2.4. Optimization of chelation parameters of Zn-FVP
Zinc sulfate (ZnSO4·7H2O),0.4 g, 0.5 g, and 0.6 g was dissolved in 100 mL ultrapure water to final zinc concentration of 4, 5, 6 mg/mL, respectively. FVPp was added into the solution at a ratio of 4:10, 5:10, 6:10 (mg/ mg) to Zn (II) for 6, 8, 10 h, respectively, at 30°C. After chelation reaction, a four-fold volume anhydrous ethanol was added to the solution to precipitate the Zn-FVP. The concentration of zinc in the supernatant was determined by FAAS and the chelation rate was calculated (Ma, Qin, Chen, & Yi, Citation2010). Based on single-factor test results (data not shown), a L9(34) orthogonal test was used to optimize the chelating reaction conditions. The variables included the initial concentration of zinc ions, the ratio of FVPp to Zn (II) (m/m), chelating time, and pH. The parameters of L9(34) orthogonal test are given in . The experiment was done in triplicate. Chelating rate was calculated as follows:
where C0 is the concentration of Zn (II) (mg/mL) in the solution before chelation, C1 is the concentration of Zn (II) (mg/mL) in the solution after chelation.
Table 1. Orthogonal factor level of chelating reaction.
2.5. Fourier transform infrared spectroscopy
Fourier transform infrared (FTIR) spectra of polysaccharides were detected using the KBr disc technique using an FTIR spectrophotometer (Thermo Scientific, USA) and scanned in the range of 400–4000 cm−1 (Souza et al., Citation2012).
2.6. X-ray diffraction analysis
X-ray diffraction (XRD) analysis for the chemical composition of polysaccharides was recorded with the D8 ADVANCE system. The powders of FVPp and Zn-FVPp were attached to the sample holder and the measurements were recorded in the range of 5–80°C (Martucci et al., Citation2015; Taboada, Larrañaga, Laserna, & Arkarazo, Citation2015).
2.7. Cell evaluation
2.7.1. Cell culture
The RAW264.7 cells were cultured at 37 °C in a humidified atmosphere of 5% CO2. Cells were grown in DMEM medium supplemented with 10% fetal bovine serum (FBS), penicillin (100 U/mL), and streptomycin (100 μg/mL).
2.7.2. Cytotoxic analysis
The RAW264.7 cells were seeded in 96-well plates at a density of 2 × 105 cells/mL and cultured 24 h before treatment. Cells stimulated by LPS (10 μg/mL) or not were divided into two groups, which were both incubated with the different concentrations of samples for 24 h. Then, 10 μL MTT (5000 μg/mL) was added into each well. After 4 h, the supernatant was removed and 100 μL DMSO was added to dissolve the crystals. The absorbance at 570 nm was measured after the plates were shaken for 10 min (Meng et al., Citation2014). Three replicates were carried out for each of the different treatments. The optical density (OD) value indicated the cell viability.
2.7.3. Cytokines assay
The RAW264.7 cells were seeded at a density of 2 × 105 cells/mL and incubated for 24 h. Stimulated by LPS (10 μg/mL), different concentrations of FVP were added into the each well . After being cultured for 24 h, Culture supernatants were taken, and the concentration of TNF-α, INF-γ, NO and IL-6 (Liu et al., Citation2015) were determined by sandwich immunoassays using ELISA kits according to the manufacturer’s instructions.
2.8. Statistical analysis
All experiments were performed in triplicate and the data were recorded as the mean ± SD. ANOVA was used to determine the significance of results between different treatments. SPSS version 19 software was used for the statistical analyses.
3. Results
3.1. Isolation and purification of FVP
Crude polysaccharide was extracted from F. velutipes powder and separated by DEAE Cellulose-52 ion chromatography and 3 main fractions were eluted ((a). As the largest component, F-1 was the water-eluted component and was chosen to be further purified by Sephadex G-100 gel chromatography. FVPp was obtained after elution by ultrapure water on the Sephadex G-100 gel resin ((b)). Lyophilized FVPp was white crystal as shown in (a).
3.2. Measurement of molecular weights
The apparent molecular weight of FVPp was determined by HPLC gel filtration chromatography, and the column was calibrated with Pullulan standard of known molecular weight. As shown in , the average molecular weight of FVPp was 890 KDa.
3.3. Optimization of chelation parameters of Zn-FVP
To obtain higher chelating rate, the chelating conditions were optimized using L9(34) orthogonal test, and the levels of every parameter were chosen by single-factor experiments (data not shown). The results of orthogonal test and extreme difference analysis are presented in and .
Table 2. Orthogonal results of chelating reaction.
Table 3. Analysis of variance.
The maximum rate of chelation was 97.28% among the 9 groups; however, the group of A2B3C2D1 was the optimal condition based on the K values listed in . Then chelating rate was determined and 98.11% chelating rate was obtained. Under this condition, the content of Zinc in Zn-FVP was 0.175 mg/mg (Zn was 1.91 × 10−4 mg/mg before chelation). White crystal Zn-FVP was obtained after freeze-drying ((b)). Therefore, the optimized chelation conditions were: initial concentration of Zn (II), 6 mg/mL, ratio of purified polysaccharide to Zn (II), 1:2; chelating time, 8 h and pH, 5.
3.4. FTIR spectroscopy of polysaccharides
The FTIR spectra of crude polysaccharide are shown in (a) and exhibit the typical signals in the range from 400 cm−1 to 4000 cm−1. The characteristic strong broad absorption at 3369.08 cm−1 corresponded to the O-H groups. The absorption peaks at 2936.92 cm−1 and 2362.84 cm−1 indicated the aliphatic C–H stretching vibrations (Santhiya, Subramanian, Natarajan, & Colloid, Citation2002). The presence of amide bands at 1637.99 cm−1 and 1407.81 cm−1 indicated the presence of some residual protein in the polysaccharide mixture. The strong band at 1636 cm−1 (1650–1600 cm−1) was overlapping with absorption of aromatics(C=O and C=C) (MacCarthy & Rice, Citation1985).
Figure 4. (a) Fourier transform infrared (FTIR) spectroscopy of crude polysaccharide, (b) Fourier transform infrared (FTIR) spectroscopy of FVPp and (c) Fourier transform infrared (FTIR) spectroscopy of Zn-FVP.
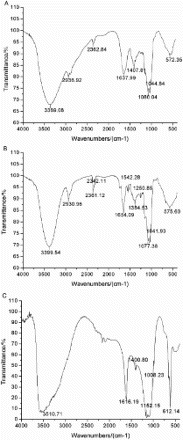
As shown in (b), characteristic band for O-H stretching at 3399.54 cm−1 and bands at ca. 2930.96 cm−1, 2361.12 cm−1 for C-H stretching were also present in FVPp. The absorption bands at 1654 cm−1 and 2342 cm−1 represent the -C≡N and N-H vibrations. Signals at ca.1550 cm−1 and 1650 cm−1 were assigned to the N-H stretching. This relatively intense band was overlapped with the phenolic C=C stretching vibrations. Additionally, the band near to 1250 cm−1 correlated to S=O groups. The results of FTIR spectroscopy could be used to elucidate the configuration of the polysaccharide and it was a furanose for there being two intense absorption bands between 1100 cm−1 and 1010 cm−1.
As shown in (c), FTIR analysis of crude polysaccharide, purified polysaccharide, and Zn-FVP confirmed the spectral pattern typical of polysaccharides with similar structural characteristics. The spectra of Zn-FVP were dominated by a broad band at about 3510 cm−1 for the stretching vibration modes of OH groups. The bands in the region of 2946 cm−1 were assigned to the C-H stretching. A new absorption band appeared at 1616 cm−1, which was indicative of the N-H stretching vibration or aromatic (C=C, C=O). What is more, the signal close to 1400 cm−1 was due to the C-H variable angle vibration.
3.5. X-ray diffraction (XRD) analysis
The XRD measurement was carried out to provide qualitative information of different elements in the FVPp and Zn-FVP. Results obtained from XRD analysis are shown in (a)–(b).
Figure 5. (a) X-ray diffraction (XRD) spectroscopy of FVPp and (b) X-ray diffraction (XRD) spectroscopy of Zn-FVP.
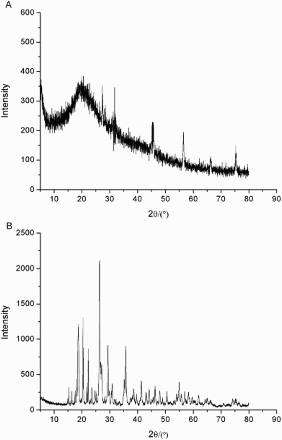
Due to the FVPp being amorphous and did not have periodical structure, (a) could only show a diffuse region corresponding to the maximum value of the diffraction when the X ray passed through the FVPp. It was difficult or even unable to make a judgment on the chemical composition of FVPp.
The qualitative spectrum of Zn-FVP indicated the presence of ZnSO4 ((b)). This result was also supported by FTIR analysis and indicated that the abundant SO42− and Zn (II) groups were widely present in the chelated samples.
3.6. Cell evaluation
3.6.1. Drug safety evaluation of FVP in RAW264.7 cells
Compared to the FVPc and FVPp, Zn-FVP supplementation in RAW264.7 cells decreased the OD570 value in a dose-dependent manner for all concentrations (12.5, 25, 50, 100, 200 and 400 μg/mL) (). Furthermore, Zn-FVP at high concentrations (100–400 μg/mL) significantly reduced the survival of macrophage (P < 0.05) while it had little effect on cells at low concentrations (12.5, 25 and 50 μg/mL) (P > 0.05). After co-cultured with LPS, Zn-FVP at different concentrations also downregulated the survival rate of cells (). However, the low concentrations (5–50 μg/mL) of Zn-FVP did not show obvious inhibition of RAW 264.7 cells (P > 0.05) compared with high concentrations, and the survival rate of RAW 264.7 cells at 50 μg/mL was 83.07%.
3.6.2. Inflammatory cytokines
TNF-α, INF-γ and IL-6 are pro-inflammatory cytokines produced during acute inflammation and responsible for a wide range of intracellular signaling events. NO is an inflammation mediator involved in both the initiation and the amplification of the inflammatory response. Previous studies have demonstrated that LPS stimulation increased the expression of TNF-α (Wang et al., Citation2013).
As shown in (A)–(D), the expression of inflammatory cytokines (TNF-α, INF-γ and IL-6) and mediators (NO) were assayed. It was obvious that LPS stimulation led to a robust increase in the productions of TNF-α, INF-γ, IL-6 and NO. Compared with the control group (10 μg/mL LPS with 0 μg/mL Zn-FVP), Zn-FVP significantly downregulated the expression of these inflammation indicators (P < 0.05), especially at the highest dosage of 50 μg/mL. Meanwhile, FVPc and FVPp activated the productions of TNF-α, IL-6, and NO compared with Zn-FVP ().
Table 4. Effect of FVP on secretion of the inflammatory cytokines and mediators (NO) in LPS-treated RAW264.7 cells.
As shown in (A), the expression of IL-6 was lower for the Zn-FVP treatments than for the FVPc treatments at all four concentrations (P < 0.05). Zn-FVP was not effective at concentrations of 25 μg/mL (P > 0.05) compared with FVPp. FVPc was effective at concentrations of 12.5 μg/mL and 25 μg/mL (P < 0.05). FVPp was not effective at concentrations of 25 μg/mL and 50 μg/mL (P > 0.05).
As shown in (B), regarding the expression of INF-γ, no significant difference was observed for FVPc at all of the concentrations (P > 0.05); FVPp was not effective at concentrations of 25 μg/mL and 50 μg/mL (P > 0.05). However, at the same concentrations, Zn-FVP was always effective compared with FVPc and FVPp (P < 0.05).
As shown in (C), no differences in the expression of TNF-α were found between the FVPc, FVPp, and Zn-FVP at a concentration of 5 μg/mL (P > 0.05). Zn-FVP was effective at concentrations of 50 μg/mL (P < 0.05) compared with FVPc and FVPp. Zn-FVP was not effective at concentrations of 5 μg/mL, 12.5 μg/mL, and 50 μg/mL (P > 0.05).
As shown in (D), no differences were found between the FVPc and Zn-FVP treatments according to the expression of NO at a concentration of 50 μg/mL (P > 0.05). And no differences were found between the FVPp and Zn-FVP treatments at concentrations of 12.5 μg/mL and 25 μg/mL (P > 0.05). Zn-FVP was effective at a concentration of 50 μg/mL compared with other three concentrations (P < 0.05).
4. Discussion
It was found that the influence to the chelating rate decreased in the order: A > B > D > C according to the R-values. ANOVA and F tests were performed to evaluate the significance of each factor. As shown in , reaction time affected the chelating rate significantly (p < 0.1); the other three factors had no significant effect on the chelating rate in the chosen range of the levels. But chelation was inhibited when the pH was lower than 4 in our single experiments.
Several studies (He & Lu, Citation2011; Li, Citation2010) have been published on the chelation of polysaccharide and zinc. The free N-H and O-H groups may contribute to the chelation with the metal ions, because they could adsorb metal ions selectively and form netted structure by the cage molecules. Characteristic bands for OH stretching at 3000–3500 cm−1 and bands corresponding to C-H variable angle vibration between 1000–1100 cm−1 showed some obvious differences for chelation of polysaccharides and zinc. At the same time, the absorption peak of N-H at ca.1654 cm−1 shifted to the 1616 cm−1, and the shift of the absorption bands groups may have contributed to the linkage of N-H and zinc ions. The absorption band at 620 cm−1 was related to the sulfate group, which indicated that physisorption played an important role in the chelation reaction.
XRD showed the existence of new functional groups by the appearance of new peaks .Compared to chelated samples, Zn signal was not observed in FVPp. New peaks were attributed to the changes of structure in Zn-FVP compared with FVPp.
The high dose of Zn (>4 × 10−4 mg/mg) could inhibit the immunoregulation activities (John & Van, Citation1982) of the body, and significantly reduced the learning and memory ability of the mice. On the contrary, similar to most plant polysaccharides, FVPc and FVPp had been proved to be helpful on promoting the proliferation of cells (Wasser, Citation2002). Zn-FVP was considered safe when the OD570 value was not significantly lower than the control group. Therefore, low concentrations (5, 12.5, 25 and 50 μg/mL) of FVP were used in the LPS-induced inflammatory study.
5. Conclusion
FVPp was separated and Zn-FVP was prepared in this study. Chelation conditions of FVPp with Zn were optimized by using L9(34) orthogonal test, and a white crystal of Zn-FVP was obtained. Chelating rate achieved 98.11% under the optimal conditions. Additionally, the structure of FVPp and Zn-FVP were characterized by FTIR and XRD.
Zn-FVP was shown to be dose-dependent to inhibit the expression of LPS-induced inflammation indicators (TNF-α, INF-γ, IL-6, and NO).The treatment of RAW 264.7 cells (cultured with Zn-FVP) did not show obvious effect on decreasing cell viability in the range of 5–50 μg/mL by the MTT assay, which demonstrated that Zn-FVP in non-cytotoxic levels suppressed LPS-induced inflammatory responses in macrophages. Therefore, Zn-FVP may prove to be an effective therapeutic drug.
Disclosure statement
No potential conflict of interest was reported by the authors.
Notes on contributors
Shuwen Zhao is a master, College of Food Science and Technology, Nanjing Agricultural University.
Biao Li is a master, College of Food Science and Technology, Nanjing Agricultural University.
Guitang Chen is a professor, Department of Food Quality and Safety, China Pharmaceutical University.
Qiuhui Hu is a professor, College of Food Science and Technology, Nanjing Agricultural University, and College of Food Science and Engineering, Nanjing University of Finance and Economics.
Liyan Zhao is a professor, College of Food Science and Technology, Nanjing Agricultural University.
References
- Beyersmann, D., & Haase, H. (2001). Functions of zinc in signaling, proliferation and differentiation of mammalian cells. BioMetals, 14, 331–341. doi: 10.1023/A:1012905406548
- Buff, C. E., Bollinger, D. W., Ellersieck, M. R., Brommelsiek, W. A., & Veum, T. L. (2005). Comparison of growth performance and zinc absorption, retention, and excretion in weanling pigs fed diets supplemented with zinc-polysaccharide or zinc oxide. Journal of Animal Science, 83, 2380–2386. doi: 10.2527/2005.83102380x
- Caulfield, L. E., de Onis, M., Blossner, M., & Black, R. E. (2004). Under nutrition as an underlying cause of child deaths associated with diarrhea, pneumonia, malaria, and measles. The American Journal of Clinical Nutrition, 80, 193–198.
- Caulfield, L. E., Richard, S. A., & Black, R. E. (2004). Undernutrition as an underlying cause of malaria morbidity and mortality in children less than five years old. The American Journal of Tropical Medicine and Hygiene, 71, 55–63.
- Chen, Y., Zhang, H., Wang, Y. X., Nie, S. P., Li, C., & Xie, M. Y. (2014). Acetylation and carboxymethylation of the polysaccharide from Ganoderma atrum and their antioxidant and immunomodulatingactivities. Food Chemistry, 156, 279–288. doi: 10.1016/j.foodchem.2014.01.111
- He, J. P., & Lu, L. (2011). Study on Flammulina velutipes Polysaccharide/Zn (II) Chelate. Science and Technology of Food Industry, 12, 301–303.
- He, T. B., Huang, Y. P., Yang, L., Liu, T. T., Gong, W.-Y., Wang, X.-J., … Hu, J.-M. (2016). Structural characterization and immunomodulating activity of polysaccharide from Dendrobium officinale. International Journal of Biological Macromolecules, 83, 34–41. doi: 10.1016/j.ijbiomac.2015.11.038
- Hou, Y. L., Ding, X., Hou, W. R., Zhong, J., Zhu, H. Q., Xu, T., & Li, J. (2013). Anti-microorganism, anti-tumor, and immune activities of a novel polysaccharide isolated from Tricholoma matsutake. Pharmacognosy Magazine, 9, 244–249. doi: 10.4103/0973-1296.113278
- Ikekawa, T., Ikeda, Y., Yoshioka, Y., Nakanishi, K., Yokoyama, E., & Yamazaki, E. (1982). Studies on antitumor polysaccharides of Flammulina velutipes (Curt. ex Fr.) Sing. II. The structure of EA3 and further purification of EA5. Journal of Pharmacobiodynamics, 5, 576–581. doi: 10.1248/bpb1978.5.576
- Jacobs, A. T., & Ignarro, L. J. (2001). Lipopolysaccharide-induced expression of interferon-beta mediates the timing of inducible nitric-oxide synthase induction in RAW 264.7 macrophages. Journal of Biological Chemistry, 276, 47950–47957.
- Jiang, C., Li, X., Jiao, Y., Jiang, D., Zhang, L., Fan, B., & Zhang, Q. (2014). Optimization for ultrasound-assisted extraction of polysaccharides with antioxidant activity in vitro from the aerial root of Ficusmicrocarpa. Carbohydrate Polymers, 110, 10–17. doi: 10.1016/j.carbpol.2014.03.027
- John, F., & Van, V. (1982). Amounts of twelve elements required to induce Se-VE deficiency in duckling. American Journal of Veterinary Research, 43, 851–857.
- Kang, S. M., Kim, K. N., Lee, S. H., Ahn, G., Cha, S.-H., Kim, A.-D., … Jeon, Y.-J. (2011). Anti-inflammatory activity of polysaccharide purified from AMG-assistant extract of Ecklonia cava in LPS-stimulated RAW 264.7 macrophages. Carbohydrate Polymers, 85, 80–85. doi: 10.1016/j.carbpol.2011.01.052
- Kozarski, M., Klaus, A., Nikšić, M., Vrvić, M. M., Todorović, N., Jakovljević, D., & Van Griensven Leo, J. L. D. (2012). Antioxidative activities and chemical characterization of polysaccharide extracts from the widely used mushrooms Ganoderma applanatum, Ganoderma lucidum, Lentinus edodes and Trametes versicolor. Journal of Food Composition and Analysis, 26, 144–153. doi: 10.1016/j.jfca.2012.02.004
- Li, S. T., Zhu, Y. L., Zhang, C., Chen, H. W., & Song, H. Y. (2011). Effect of intra-polysaccharide zinc-riched of Cordyceps militaris on micronucleus of Vicia faba root tip cells induced by Mitomycin-c. Science and Technology of Food Industry (in Chinese), 32, 146–152.
- Li, Z. Z. (2010). Preparation of Boletus polysaccharide zinc complexes. Research and Development of Foods (in Chinese), 3, 123–125.
- Liu, F., Pei, F., Mariga, A. M., Gao, L., Chen, G., & Zhao, L. (2015). Separation and speciation analysis of zinc from Flammulina velutipes. Journal of Food and Drug Analysis, 23, 630–635. doi: 10.1016/j.jfda.2014.06.008
- Ma, L. H., Qin, W. D., Chen, X. H., & Yi, J. W. (2010). Preparation and antioxidant activity evaluation of Flammulina velutipes polysaccharide/Fe(II) chelate. Food Science (in Chinese), 31, 202–207.
- MacCarthy, P., & Rice, J. A. (1985). Spectroscopic methods (other than NMR) for determining functionality in humic substances. In G. R. Aiken, G. R. McKnight, R. L. Wershaw, & P. MacCarthy (Eds.), Humic substances in soil, sediment, and water (pp. 527–560). New York, NY: Wiley–Interscience.
- Martucci, A., Rodeghero, E., Pasti, L., Bosi, V., & Cruciani, G. (2015). Adsorption of 1,2-dichloroethane on ZSM-5 and desorption dynamics by in situ synchrotron powder X-ray diffraction. Microporous and Mesoporous Materials, 215, 175–182. doi: 10.1016/j.micromeso.2015.05.035
- Meng, L. Z., Feng, K., Wang, L. Y., Cheong, K. L., Nie, H., Zhao, J., & Li, S. P. (2014). Activation of mouse macrophages and dendriticcells induced by polysaccharides from a novel Cordyceps sinensis fungus UM01. Journal of Functional Foods, 9, 242–253. doi: 10.1016/j.jff.2014.04.029
- Miao, L. C., Wang, L. Q., & Wu, D. (2003). Experimental study on the effect of FVP on antitumor and antileukemia in mice. Pharmaceutical Journal of Chinese People’s Liberation Army, 19, 171–173.
- Sang, C. J., Yong, T. J., Sang, M. L., & Jin, H. K. (2015). Immune-modulating activities of polysaccharides extracted from brown algae Hizikia fusiforme. Bioscience, Biotechnology, and Biochemistry, 79, 1362–1365. doi: 10.1080/09168451.2015.1018121
- Santhiya, D., Subramanian, S., Natarajan, K. A., & Colloid, I. J. (2002). Surface chemical studies on sphalerite and galena using extracellular polysaccharides isolated from Bacillus polymyxa. Science, 256, 237–248.
- Sibikina, O. V., Iozep, A. A., & Moskvin, A. V. (2009). Polysaccharide complexes with metal cations: Structure and application. Pharmaceutical Chemistry Journal, 43, 341–345. doi: 10.1007/s11094-009-0292-1
- Souza, B. W. S., Cerqueira, M. A., Bourbon, A. I., Pinheiro, A. C., Martins, J. T., Teixeira, J. A., … Vicente, A. A. (2012). Chemical characterization and antioxidant activity of sulfated polysaccharide from the red seaweed Gracilaria birdiae. Food Hydrocolloids, 27, 287–292. doi: 10.1016/j.foodhyd.2011.10.005
- Taboada, N. P., Larrañaga, A., Laserna, O. G., & Arkarazo, I. M. (2015). The relevance of the combination of XRD and Raman spectroscopy for the characterization of the CaSO4–H2O system compounds. Microchemical Journal, 122, 102–109. doi: 10.1016/j.microc.2015.04.010
- Walsh, C. T., Sandstead, H. H., Prasad, A. S., Newberne, P. M., & Fraker, P. J. (1994). Zinc health effects and research priorities for the 1990's. Environmental Health Perspectives, 102, 5–46. doi: 10.1289/ehp.941025
- Wang, X. F., Wang, S. Y., Li, Y. L., Wang, F., Yang, X. J., & Yao, J. H. (2013). Sulfated Astragalus polysaccharide can regulate the inflammatory reaction induced by LPS in CaCO2 cells. International Journal of Biological Macromolecules, 60, 248–252. doi: 10.1016/j.ijbiomac.2013.05.037
- Wasser, S. P. (2002). Medicinal mushrooms as a source of antitumor and immuno- modulating polysaccharides. Applied Microbiology and Biotechnology, 3, 258–274.
- Wu, D., Duan, W., Liu, Y., & Cen, Y. (2010). Anti-inflammatory effect of the polysaccharides of golden needle mushroom in burned rats. International Journal of Biological Macromolecules, 46, 100–103. doi: 10.1016/j.ijbiomac.2009.10.013
- Wu, X. Z., & Gao, X. D. (2002). The fiver protective and antitumor effects of extract from Flammulina velutipes in mice. Biochemical Pharmacology, 23, 176–178.
- Yang, W., Fang, Y., Liang, J., & Hu, Q. (2011). Optimization of ultrasonic extraction of Flammulina velutipes polysaccharides and evaluation of its acetylcholinesterase inhibitory activity. Food Research International, 44, 1269–1275. doi: 10.1016/j.foodres.2010.11.027
- Yang, W., Pei, F., Shi, Y., Zhao, L., Fang, Y., & Hu, Q. (2012). Purification, characterization and anti-proliferation activity of polysaccharides from Flammulina velutipes. Carbohydrate Polymers, 88, 474–480. doi: 10.1016/j.carbpol.2011.12.018
- Zalewski, P. D. (1996). Zinc and immunity: Implications for growth, survival and function of lymphoide cells. Journal of Nutritional Immunology, 4, 39–80.
- Zeng, W. C., Zhang, Z., & Jia, L. R. (2014). Antioxidant activity and characterization of antioxidant polysaccharides from pine needle (Cedrus deodara). Carbohydrate Polymers, 108, 58–64. doi: 10.1016/j.carbpol.2014.03.022
- Zhang, M., Cui, S. W., Cheung, P. C. K., & Wang, Q. (2007). Antitumor polysaccharides from mushrooms: A review on their isolation process, structural characteristics and antitumor activity. Trends in Food Science and Technology, 18, 4–19. doi: 10.1016/j.tifs.2006.07.013
- Zhao, L., Dong, Y., Chen, G., & Hu, Q. (2010). Extraction, purification, characterization and antitumor activity of polysaccharides from Ganoderma lucidum. Carbohydrate Polymers, 80, 783–789. doi: 10.1016/j.carbpol.2009.12.029
- Zhao, L., Fu, Y., Chen, C., Yang, W., & Hu, Q. (2015). Ultrasonic-assisted extraction and chromatography separation of polysaccharides from the base of Flammulina velutipes stipe. Separation Science and Technology, 50, 824–832. doi: 10.1080/01496395.2014.964728
- Zhao, Z. Y., Xu, X. J., Ye, Q. W., & Dong, L. L. (2013). Ultrasound extraction optimization of Acanthopanax senticosus polysaccharides and its antioxidant activity. International Journal of Biological Macromolecules, 59, 290–294. doi: 10.1016/j.ijbiomac.2013.04.067