ABSTRACT
This study was conducted to evaluate the interactions between the gut microbiota, ochratoxin A and functional food such as honey and quercetin, and the consequences of these interactions on ochratoxin-induced DNA damage in blood, liver and kidney cells. Honey (2 g kg−1) or Quercetin (50 mg kg−1) was applied to mice by intragastric application every day for 15 days, immediately before ochratoxin treatment (100 µg kg−1). We investigated colonic probiotic bacteria count, β-glucuronidase activity, the alkaline comet assay in blood, liver and kidney, the number of cells in the peritoneal cavity, macrophage spreading index and hematological and biochemical parameters. Honey and QU may reduce ochratoxin-induced DNA damage in the liver and kidney, β-glucuronidase activity and inflammation, partly through increasing the colon Bifidobacteria and Lactobacilli counts. The obtained results suggest that honey and QU counteracted the OTA-induced toxicity due to their bifidogenic activity and antigenotoxic activity.
GRAPHICAL ABSTRACT
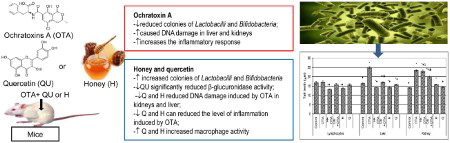
Introduction
Mycotoxins, secondary metabolites of fungi, have significant human and animal health, economic and international trade implications (Malir, Ostry, & Novotna, Citation2013; Ostry, Malir, & Ruprich, Citation2013; Pfohl-Leszkowicz & Manderville, Citation2007). Ochratoxin A (OTA), a mycotoxin produced by several Aspergillus and Penicillium species, is classified as a group 2B carcinogen (Limonciel & Jennings, Citation2014) by the International Agency for Research on Cancer. Ingestion of OTA-contaminated feed or food has been associated with nephrotoxic (Malir et al., Citation2013; Pfohl-Leszkowicz & Manderville, Citation2012), genotoxic (Gupta, Bandopadhyay, Paul, & Majumder, Citation1979) and carcinogenic (Pfohl-Leszkowicz & Manderville, Citation2007) effects, and perturbation of normal blood coagulation (Hassan et al., Citation2012) and immune response (Marin-Kuan, Cavin, Delatour, & Schilter, Citation2008). The carcinogenic molecular mechanisms of OTA are based on the formation of DNA adducts or epigenetic changes (Fusi et al., Citation2010; Kocic et al., Citation2014). Several epigenetic mechanisms including protein synthesis inhibition, oxidative stress, alterations of cell signaling pathways and apoptosis in cell lines have been reported for OTA carcinogenicity (Gupta et al., Citation1979; Pfohl-Leszkowicz & Manderville, Citation2007; Salminen et al., Citation2010). A tolerable daily intake of 5 ng/kg b.w. was derived for OTA on the basis of the lowest observed adverse effect level (Cencic & Chingwaru, Citation2010).
Food contaminants entering the body through the oral route are directly exposed to the action of gut microflora. Gastrointestinal (GI) microflora is increasingly being recognized as one of the factors that influence human health as well as animal health. This microflora is in a dynamic equilibrium that may be altered by diet (Bennett & Klich, Citation2003; Davis & Milner, Citation2009; Saarela, Lahteenmaki, Crittenden, Salminen, & Mattila-Sandholm, Citation2000). Several studies showed that colonic probiotic bacteria can remove mycotoxins via physical binding as a mechanism for mutagen removal (Bennett & Klich, Citation2003; Davis & Milner, Citation2009; Greiner, Papineni, & Umar, Citation2014; Saarela et al., Citation2000). In addition, the severity of mycotoxin poisoning can be amplified by factors such as vitamin deficiency, caloric deprivation, alcohol abuse and infectious disease status (Greiner et al., Citation2014).
The intestinal microflora plays a considerable role in the metabolism of endogenous and exogenous substances in the diet, and is thought to participate in both beneficial and deleterious effects on health. Several attempts to improve bacterial metabolism have been made. One strategy is to supplement the diet with substances that promote the growth of lactic acid bacteria (LAB) which are thought to play an important role in maintaing health by stimulating the immune system, protecting the host from invading bacteria, viruses and toxins, enhancing signaling in host cells to reduce inflammatory response, and aiding digestion and assimilation of food. These bacteria create conditions unfavorable for the growth of potentially pathogenic species (Oršolić & Bašić, Citation2008; Saulnier, Spinler, Gibson, & Versalovic, Citation2009) by producing strong acids as metabolic end products (acetate, lactate), organic acids, hydrogen peroxide and bacteriocins and they play a role in synthesizing B vitamins, folic acid, vitamin K and lysozyme as well as metabolizing bile acids, sterols and xenobiotics (Oršolić & Bašić, Citation2008; Saulnier et al., Citation2009). Furthermore, as dietary supplements LAB may help to prevent constipation and regulate transit time, prevent diarrhea and colonic cancer, reduce blood lipid levels and stimulate the immune system by increasing the phagocytic activity of macrophages, and T and B cells.
Among foods that possess the characteristic of functionality, we may include all those originating in the beehive: honey, propolis and royal jelly. Honey is regarded as a functional food largely due to the presence of phenolic compounds that are able to act as free radical scavengers, thus protecting cells and living tissues against oxidative stress. This oxidative stress has been associated with enhanced cellular ageing that is itself a feature of degenerative diseases, cancer, cardiovascular diseases, GI diseases and related diseases (Oršolić, Citation2009; Oršolić & Bašić, Citation2008). The benefits of bee honey to human health have long been recognized. Beside antioxidant activity, honey possesses chemopreventive, anti-atherogenic, immunoregulatory, antimicrobial, wound healing and bifidogenic properties. It contains more than 180 substances, including amino acids, vitamins, minerals and enzymes (Kenjeric, Mandic, Primorac, Bubalo, & Perl, Citation2007; Oršolić, Citation2009). In general, it is composed primarily of fructose and glucose but also contains 4–5% fructooligosaccharides (FOS), which serve as prebiotic agents (Kenjeric et al., Citation2007; Oršolić, Citation2009).
Numerous flavonoids, including polyphenol quercetin, were determined in many European monofloral types of honey (Piljac-Žegarac, Stipčević, & Belščak, Citation2009; Tomas-Barberan, Martos, Ferreres, Radovic, & Anklam, Citation2001; Yao, Jiang, Singanusong, Datta, & Raymont, Citation2004). Quercetin (3,3’,4’,5,7-pentahydroxyflavone) is one of the most abundant flavonoids in the human diet, found in fruits and vegetables such as blueberries, onions, broccoli and leek (Manach, Scalbert, Morand, Rémésy, & Jiménez, Citation2004), herbal medicine and red wine (Boots, Haenen, & Bast, Citation2008) as well as in honey.
It was also suggested that quercetin acts as an antioxidant by inhibiting xanthine oxidase, lipoxygenase and NADPH oxidase, oxidative enzymes that play key roles in the initial process of free radical-induced cellular damage (Benkovic et al., Citation2008; Moskaug, Carlsen, Myhrstad, & Blomhoff, Citation2004; Oršolić et al., Citation2007, Citation2010, Citation2011; Oršolić & Car, Citation2014; Sirovina et al., Citation2013). In addition, much attention has been focused on the influence of quercetin on signaling pathways and its indirect interaction with the endogenous antioxidant defense system (Oršolić & Car, Citation2014; Sirovina et al., Citation2013) such as phase I and II detoxifying enzymes, and its ability to increase the expression of the rate limiting enzyme c-glutamylcysteine synthetase in the synthesis of glutathione (GSH) and induce xenobiotic responsive elements in CYP 450 family genes (Myhrstad, Carlsen, Nordström, Blomhoff, & Moskaug, Citation2002; Yao et al., Citation2007). Furthermore, Yao et al. (Citation2007) demonstrated that plant-derived flavonoids or polyphenols, including quercetin, may act as non-stressful and non-cytotoxic heme oxigenase-1 (HO-1) inducers maximizing cellular intrinsic antioxidant potential, while Morales et al. (Citation2006) suggested that renal protection exerted by quercetin is based on its ability to increase metallothionein in kidneys. It has been demonstrated that dietary fiber has a significant protective effect against colon cancer by acidifying the colonic contents. Polyphenols, including QU, are also potential modulators of digestive fermentation processes because, in part, they escape absorption in the small intestine and reach the large intestine, where they themselves or their metabolites affect colonic/cecal bacteria (Juśkiewicz et al., Citation2012).
There is evidence that colonic probiotic bacteria, including LAB such as Lactobacillus acidophilus, and Bifidobacteria (De Petrino, De Jorrat, De Budeguer, & Perdigón, Citation1997; Pirker et al., Citation2013), may positively modulate certain colon microbial enzymes that play a role in diet-related diseases (Oršolić, Citation2009). For example, β-glucuronidase is an inducible enzyme elaborated by anaerobic E. coli, Peptostreptococcus, Bacteroides and Clostridia. Increased activity of this enzyme has been implicated in increased enterohepatic recirculation of toxins, hormones, drugs and carcinogens. In fact, excessive β-glucuronidase activity may be a primary factor in the etiology of colon cancer.
Complete elimination of any natural toxin from foods is an unattainable objective but we proposed that interventions by increased Lactobacillus and Bifidobacteria, FOS, high-fiber diet and lowering colonic pH may help to reduce β-glucuronidase levels.
Thus, this work could be summarized as follows:
Firstly, we determined in vivo if honey and quercetin are able to counteract the effects of mycotoxin on the growth of the endogenous colonic probiotic bacteria, activity of colon microbial enzyme β-glucuronidase, inflammatory and immune response in peritoneal cavity and peripheral blood of mice, and evaluated antigenotoxic effect of honey and quercetin against OTA-induced DNA damage on leukocytes, liver and kidney cells. Secondly, this work suggested the capacity of functional food ingredients as an alternative, non-pharmaceutical approach in maintaining human gut health and well-being through promotion of gut microbial homeostasis.
Material and methods
Animals
The present study was approved by the ethical committee (Faculty of Science, University of Zagreb, Croatia). Male Swiss albino mice 2–3 months old, weighing 20–25 g, obtained from Department of Animal Physiology, Faculty of Science, University of Zagreb, were used in this study. Ten animals were kept in the same cage and feed a standard diet for laboratory animals (Standard Diet GLP, 4RF21, Mucedola, Settimo Milanese MI, Italy), with access to water ad libitum. The Standard Diet GLP, 4RF21 contained protein (18.82%), crude fat (3.47), crude fiber (5.93%), moisture (9.28%) and ash (6.81%). We used 12 h regime changes of day and night. The research was conducted in accordance with the Law on the Protection of laboratory animals (NN #135/06) and carried out in compliance with the Guide for the Care and Use of Laboratory Animals, DHHS Publ. # (NIH) 86-123.
Ochratoxin A
OTA was purchased from Sigma–Aldrich Chemical Company, St. Louis (Cat. No. 01877). OTA was given to mice by intragastric (ig) application every day for 15 days at a dose of 100 µg kg−1 body weight (Kamp et al., Citation2005).
Honey
Fresh samples of honey from wild flowers were collected from beehives in areas nearby Zagreb and stored in the dark at 4°C until use. Under aseptic conditions, dilutions of honey were prepared using sterile distilled water. Honey was given to mouse by ig application every day for 15 days at a dose of 2 g kg−1 body weight (Oršolić, Citation2009).
Determination of bioactive component of honey
All of the chemicals and reagents used were of analytical grade and purchased from Sigma (St. Louis, USA) and Merck (Darmstadt, Germany). Each honey sample (4 g) was distributed into test tubes and diluted to 20 mL with distilled water, using a vortex mixer. The solution was then filtered through Whatman No.1 and analyzed for total phenolic (TP) content and total flavonoid (TF) content. All analyses were performed in triplicate and the data were expressed as means ± standard deviations (SD).
Determination of total polyphenols
Phenolic compounds in honey were estimated by a spectrophotometric determination with a modified Folin-Ciocalteu method (Singleton, Orthofer, & Lamuela-Raventos, Citation1999). Briefly, 1 mL of diluted honey sample (0.2 g mL−1) was mixed with 1 mL of Folin and Ciocalteu’s phenol reagent. After 3 min, 1 mL of 10% sodium carbonate solution was added to the mixture and adjusted to 10 mL with distilled water. The mixture was kept in the dark for 90 min, after which the absorbance was read at 725 nm by a T 80 UV/VIS spectrophotometer (ChromoTek GmbH, Germany). Gallic acid was used to calculate the standard curve (0–100 μg mL−1). Estimation of the phenolic compounds was carried out in triplicate. The results are presented as mean values ± standard deviations and expressed as milligrams of gallic acid equivalents (GAEs) per kg of honey.
Determination of TFs
TF contents of honey were determined according to the colorimetric assay developed by Singleton et al. (Citation1999). One milliliter of diluted honey (0.2 g mL−1) was mixed with 4 mL of distilled water. At zero time, 0.3 mL of (5% w/v) NaNO2 was added. After 5 min, 0.3 mL of (10% w/v) AlCl3 was added. After 6 min, 2 mL of 1 M solution of NaOH were added. After that, the volume was immediately made up to 10 mL, by the addition of 2.4 mL of distilled water. The mixture was shaken vigorously and the absorbance of the mixture was read at 415 nm. A calibration curve was prepared using a standard solution of quercetin (0–100 μg mL−1). The results were also expressed as mg quercetin equivalents (QUEs) per kg of honey.
Quercetin
Quercetin (Quercetin dehydrate 98%, Aldrich Ch. Co. Inc. Milwauke WI, USA) was used in the study. Before use QU was dissolved in ethanol and further dilutions were made in water. The final concentration of ethanol was equal to 0.5% (v/v). Quercetin was given to mice by ig application every day for 15 days at a dose of 50 mg kg−1 body weight (Benkovic et al., Citation2008; Oršolić et al., Citation2007, Citation2010, Citation2011; Oršolić & Car, Citation2014; Sirovina et al., Citation2013).
Experimental design
A total of 60 Swiss albino mice were used in the study. Honey (2 g kg−1) or quercetin (50 mg kg−1) was applied to mice by ig application every day for 15 days immediately before OTA treatment at a dose of 100 µg kg−1 body weight. Mice were randomly divided into 6 groups, as follows:
Group (i): control animals (healthy animals); mice received ig vehicle (0.5% ethanol) every day for 15 days;
Group (ii): OTA animals; mice received ig OTA at a dose of 100 µg kg−1 body weight every day for 15 days;
Group (iii): received honey (H) ig at a dose of 2 g kg−1 body weight + OTA, every day for 15 days and served as H-preventive-treated OTA group;
Group (iv): received quercetin (QU) ig at a dose of 50 mg kg−1 body weight + OTA, every day for 15 days and served as QU-preventive-treated OTA group.
Groups (v and vi) received test components only (H or QU).
All mice were fed and caged under consistent conditions during the study. The dosing was adjusted according to the status of the mouse’s weight on a daily basis. One hour after the last dose of OTA and/or honey and QU, animals were weighed, and anesthetized using ketamine (Narketan®10, Vetoquinol, Lure, France) at a dose of 100 mg kg−1 and Xylapan® (Vetoquinol Biowet, Gorzow, Poland) at a dose 5 mg kg−1. Exsanguinations were performed from axillary blood vessels for hematological and biochemical parameters analysis and comet assay. The blood was immediately placed on ice prior to isolation of the serum by centrifugation.
After disinfection of the external abdominal region, each animal was inoculated with 3 mL of saline solution and after gentle agitation of the abdominal wall, the solution containing peritoneal cells was removed for cellular evaluation. Liver and kidney tissues were carefully dissected from their attachments and totally excised. The following variables were analyzed: total cell number in peritoneal cavity, the functional activity of macrophages and the comet assay from peripheral blood, liver and kidney cells.
In addition, fresh fecal samples (weight 0.1 g) were collected from each mouse for count of the colonies of probiotic bacteria (Lactobacilli and Bifidobacteria) and colonic microbiota enzyme activities (β-glucuronidase). Experimental groups were additionally monitored for body weight gains (body weight was recorded at the beginning and on termination of the study).
Count of the total number of cells present in the peritoneal cavity
The total number of cells present in the peritoneal cavity was determined by counting in a Bürker-Türk chamber.
Determination of functional activity of macrophages
The functional activity of macrophages in the peritoneal cavity was determined by the spreading technique (Oršolić & Car, Citation2014). Thus, 103 cells in 0.1 mL of the cellular suspension obtained from the peritoneal cavity were placed in duplicate over glass cover slips at room temperature for 15 min. The non-adherent cells were removed by washing with phosphate buffered saline, and the adherent cells were incubated in culture medium 199 containing 10 nM HEPES at 37°C for 1 h. Following this, the culture medium was removed and the cells were fixed with 2.5% glutaraldehyde. Then, the cells were stained with a 5% solution of Giemsa and examined by optical microscopy. The percentage of spread cells was determined using a ×40 objective. Spread cells were those that exhibited cytoplasmic elongation, while the non-spread cells were rounded (Oršolić & Car, Citation2014). Using an ocular grid, 200 macrophages were scored as either round or spread. An index of macrophage spreading (SI) was then calculated for each monolayer of each glass cover slips, as follows:
SI = (number of spreading macrophages × 100)/200 adherent cells, that is, SI = % of spreading macrophages.
Analysis of hematological and biochemical parameters
Blood samples are taken from the axillary plexus of blood vessels of experimental animals and placed in a heparinized glass vacutainer with the addition of EDTA (Becton Dickinson, Plymouth, United Kingdom) and kept at 4°C for 2–4 hours to determine the hematological parameters.
To obtain the serum, blood samples were immediately placed in tubes without anticoagulant and coagulated blood was centrifuged at 3000 rpm for 10 minutes. After centrifugation, serum was collected in 1.5 mL polypropylene tubes (Eppendorf AG, Hamburg, Germany) and stored at −20°C until determination of the following biochemical parameters: aspartate aminotransferase (AST), alanine aminotransferase (ALT), alkaline phosphatase (AP), urea, blood glucose levels (glucose), lactate dehydrogenase (LDH) and total protein.
The hematological parameters determined were the number of erythrocytes (Red blood cells, RBC), the mean corpuscular volume of erythrocytes (MCV), hemoglobin (Hgb), hematocrit (Hct), mean corpuscular hemoglobin (MCH), mean corpuscular hemoglobin concentration (MCHC), total leukocyte count (White blood cells, WBC), the total number of platelets (Plt), the percentage of the individual leukocytes in peripheral blood cells as well as percentage (or number) of neutrophils, lymphocytes and basophiles. Blood samples were analyzed by standard laboratory methods. For the determination of hematological and biochemical parameters, we used blood cell counter Cell-Dyn® 3200 (Abbott, USA), and the biochemical apparatus Alcylon 300 (Abbott, USA).
The alkaline comet assay
To evaluate the impact of OTA and natural products honey and QU on DNA molecule, the comet test was carried out under alkaline conditions, basically as described by Singh, McCoy, Tice, and Schneider (Citation1988). Fully frosted slides were covered with 1% normal melting point (NMP) agarose (Sigma, St. Louis, MO). After solidification, the gel was scraped off the slide. The slides were then coated with 0.6% NMP agarose. When this layer had solidified, a second layer containing the whole blood sample or liver and kidney cells mixed with 0.5% low melting point (LMP) agarose (Sigma) was placed on the slides. After 10 min of solidification on ice, the slides were covered with 0.5% LMP agarose. The slides were then immersed for 1 h in ice-cold freshly prepared lysis solution (2.5 M NaCl, 100 mM disodium EDTA, 10 mM Tris-HCl, 1% sodium sarcosinate (Sigma), pH 10) with 1% Triton X-100 (Sigma) and 10% dimethyl sulfoxide (Kemika, Zagreb, Croatia) to allow DNA unfolding. The slides were then placed on a horizontal gel electrophoresis tank, facing the anode. The unit was filled with fresh electrophoresis buffer (300 mM NaOH, 1 mM disodium EDTA, pH 13.0) and the slides were placed in this alkaline buffer for 20 min to achieve complete DNA unwinding and expression of alkali-labile sites. Denaturation and electrophoresis were performed at 4°C under dim light. Electrophoresis was carried out for 20 min at 25 V (300 mA). After electrophoresis, the slides were rinsed gently three times with neutralization buffer (0.4 M Tris-HCl, pH 7.5) to remove excess alkali and detergents. Each slide was stained with ethidium bromide (20 µg mL−1) and covered with a coverslip. The slides were then stored in sealed boxes at 4°C until analysis. A total of one hundred randomly captured comets from each slide were examined at 250× magnification using an epifluorescence microscope (Zeiss, Germany) connected through a black and white camera to an image analysis system (Comet Assay II; Perceptive Instruments Ltd., UK). A computerized image analysis system was used to acquire images, compute the integrated intensity profile for each cell, estimate the comet cell components and evaluate the range of derived parameters. To quantify DNA damage, three comet parameters were evaluated: tail length, tail intensity (% of DNA in tail) and tail moment. Tail moment was calculated as (tail length × % of DNA in tail)/100.
Collection of samples for colonic probiotic bacteria count and β-glucuronidase activity
After a 15-day period of intragastric application of OTA, either alone or in combination with honey or quercetin, and/or co-administration ochratoxin with test components to mice, all experimental groups of mice were killed by Ketamine/Xylapan anesthesia. Their ceca were removed and fresh fecal samples (weight 0.1 g) were collected from each mouse. The cecum content samples were immediately (within 30 min) placed in anaerobe jars and kept at 4°C until analyzed (a maximum of 6 hours.). The cecum contents were diluted 10-fold in pre-reduced Ringer solution containing 0.5% of cysteine and subsequently homogenized. Each cecal digest homogenate was serially diluted from 10−1 to 10−9. Dilutions were subsequently plated on selective agar media, in triplicate, for the determination of colonic probiotic bacteria count.
Colonic probiotic bacteria (Lactobacilli and Bifidobacteria) counts
The endogenous populations of colonic probiotic bacteria (Lactobacilli and Bifidobacteria) were counted. Dilutions of 10−5, 10−6, 10−7, 10−8 and 10−9 of cecum content samples were plated (0.1 mL) on the Petri dishes of various media and incubated for 48 h at 37°C in anaerobic atmosphere using Gen Kits in Oxoid jars. Bacteria were detected on selective media as follows: deMan, Rogosa, Sharpe (MRS) medium (Oxoid, Hampshire, United Kingdom) for Lactobacilli and Tryptone Phytone Yeast medium (Oxoid) for Bifidobacteria. After incubation, the colonies were counted. Data were expressed as CFU per gram of content [log10 (CFU/g)].
Bacterial β-glucuronidase activity
Bacterial β-glucuronidase activity was assayed in the cecum content digest with P-nitrophenyl-β-D-glucuronide (Sigma, St. Louis, MO) (Djouzi & Andrieux, Citation1997). Cecum dilution was performed at 4°C. The reaction mixture contained 0.3 mL substrate solution (5 M P-nitrophenyl-β-D-glucuronide) and 0.2 mL of the diluted cecum (1 part of cecum and 2 parts phosphate buffer pH 6.4, w/v) and mixed well, incubated at 37°C for 48 h. The concentration of released P-nitrophenol was measured from the optical absorption at 400 nm after addition of 2.5 mL NaOH 0.1 N to stop reaction. Enzyme activity was expressed as moles of product formed/g cecum content.
Statistical analysis
The parameters of tail intensity and tail moment were evaluated using Statistica 5.0 package (StaSoft, Tulsa, OK). Each sample was characterized for the extent of DNA damage by considering the mean ± SE. In order to normalize distribution and to equalize the variances, a logarithmic transformation of data was applied. Multiple comparisons between groups were done by ANOVA on log-transformed data. Post-hoc analysis of differences was done by the Scheffé test. Other results are expressed as means ± SE obtained from two experiments. The significance of differences between means of the groups was tested by Student’s t-test. The level of statistical significance was set at P < .05.
Results
TP and flavonoid content of honey
Polyphenols/flavonoids are important groups of compounds regarding the appearance and the functional, health-promoting or health-improving properties of honey. The TP content of honey was investigated by the Folin-Ciocalteu assay and the mean values and standard deviation are shown in . The total polyphenol content was 572.2 ± 2.05 mg GAE/kg honey and the TF content was 59.85 ± 3.0 mg QU/kg honey.
Table 1. Total phenols and flavonoid content in Croatian honey.
Effect of OTA, honey or quercetin on body weight
There were no significant alterations in body weights in any of the groups before OTA treatment. However, we observed a time-dependent decrease in the body weight of OTA group throughout 15 days. In the first week of OTA application, a ∼5% decrease in the body weight was evident, and at the termination of the experiments body weight of the OTA group was ∼9% lower than in control group. Co-administration of honey and QU with OTA did not cause significant changes in the body weight of mice (data not shown). The honey and QU applied alone had no significant effect on body weight gains.
Co-administration of honey or quercetin with OTA increased counts of colonic probiotic bacteria
LAB are proposed to have several beneficial effects, including the inactivation and physical binding of carcinogens. Since several studies showed that microflora may be altered by diet and that colonic probiotic bacteria can remove mycotoxins via physical binding as mechanism for mutagen removal, we determined the effect of honey or quercetin on the endogenous colonic probiotic bacteria count, activity of colonic microbial enzyme β-glucuronidase and OTA-induced DNA damage.
Analysis of the number of colonies of probiotic bacteria showed that the intake of honey and quercetin increased the number of probiotic bacteria in relation to the control (). Addition of honey to Standard Diet GLP, 4RF21 increased the counts of Bifidobacteria (9.322 ± 0.20 vs. 6.35 ± 0.23 log10 CFU/g) and Lactobacilli (6.70 ± 0.81 vs. 5.07 ± 0.70 log10 CFU/g) in comparison to control. In the combined treatment (H + OTA), number of Bifidobacteria or Lactobacilli was higher for 1.14 ± 0.22 or 0.75 ± 0.22, respectively, compared to the OTA-treated group.
Table 2. Effect of honey, quercetin and OTA on lactic acid bacteria (LAB) growth (log10 CFU/g).
Administration of OTA in the presence of QU (OTA + QU group) increased the mean counts of Bifidobacteria by 1.14 ± 0.32 log10 CFU/g (P < .05) and Lactobacilli by 0.38 ± 0.17 log10 CFU/g in comparison to the OTA group, respectively. Administration of QU alone did not cause a significant increase in the number of colonies of Lactobacilli and Bifidobacteria compared to control.
Quercetin reduces the activity of β-glucuronidase in the cecum content
shows the activity of β-glucuronidase in the cecum content. There were no significant differences between the groups of mice administered with honey, OTA, H + OTA and the control regarding β-glucuronidases activity in the cecum contents (P ≥ .05). Quercetin administration alone or in combination with OTA (QU + OTA) to mice resulted in significant decreases (P < .05) in fecal β-glucuronidase activity after two weeks as compared to control and OTA-treated mice.
Table 3. Effect of honey, quercetin and OTA on bacterial glucuronidase activity in the cecum content.
Honey or quercetin protected kidney and liver tissues cells from OTA-induced DNA damage
In the present study, by using the alkaline comet assay, we evaluated whether honey and quercetin could protect mouse lymphocytes, and kidney and liver tissues cells against the OTA-induced genotoxicity.
A significant increase in DNA damage parameters (tail length, tail intensity and tail moment) was detected in the liver and kidney cells of OTA-exposed animals compared to the control group (P < .05), while changes in the level of DNA damage in the lymphocytes of OTA-exposed animals were less than in the liver and kidney (–). Honey or QU co-administration with OTA every day for 15 days significantly reduced DNA damage in liver and kidney cells compared to OTA. Co-treatment of QU or honey with OTA reduced the tail length in liver by 20% and 43%, respectively, compared to treatment with OTA (). In the renal cells, co-treatment of QU or honey with OTA leads to reduction of the tail length by 19.6% and 7.6% ().
Table 4. Comet assay responses in lymphocytes of the mice treated with honey, quercetin and OTA.
Table 5. Comet assay responses in liver cells of the mice treated with honey, quercetin and OTA.
Table 6. Comet assay responses in kidney cells of the mice treated with honey, quercetin and OTA.
Hematological and biochemical parameters
We noticed increased number of erythrocytes, hemoglobin and hematocrit in groups that received quercetin and honey, either administered alone or in combination with OTA (P ≤ .05) (). Furthermore, we noticed increase in the number of leukocytes in the group that received H + OTA as a result of increases in the number of active macrophages and lymphocytes. OTA administration caused significant elevation in serum ALP, ALT, AST, LDH and urea while level of total proteins was decreased.
Table 7. Effect of honey, quercetin and OTA on hematological parameters in mice.
Significant decreases in their mean serum levels were observed in groups treated with honey or quercetin compared to the OTA-treated group ().
Table 8. Effect of honey, quercetin and OTA on biochemical parameters in mice.
Effect of honey, quercetin and OTA on total number of inflammatory cells and macrophage activity
The total number of inflammatory cells was increased in OTA-treated mice compared to other groups, but without statistical significance (). A higher macrophage spreading index was observed in the group of mice treated with OTA by 15.05% compared to the control. Honey or QU in combination with OTA decreased the percentage of the macrophage spreading index by 12.08% or 5.8% compared to OTA.
Table 9. Cell number and macrophage spreading index of mice treated with honey, quercetin and OTA.
Discussion
Since several studies have shown that microflora may be altered by diet and that colonic probiotic bacteria can remove mycotoxins via physical binding or inactivation (Bennett & Klich, Citation2003; Davis & Milner, Citation2009; Greiner et al., Citation2014; Haskard, El-Nezami, Kankaanpaa, Salminen, & Aholmas, Citation2001; Rafter, Citation2002; Saarela et al., Citation2000; Saulnier et al., Citation2009), we determined the effect of honey or quercetin on the endogenous colonic probiotic bacteria, activity of colonic microbial enzyme β-glucuronidase and OTA-induced DNA damage.
Our main results show that: (a) OTA reduced endogenous populations of colonic probiotic bacteria (Lactobacilli and Bifidobacteria) in the cecum of mice; (b) honey and quercetin increased the number of Lactobacilli and Bifidobacteria colonies whether administered alone or in combination with OTA; (c) quercetin significantly reduced β-glucuronidase activity in the cecum content; (d) OTA caused significant damage to the DNA of the liver and kidneys as assessed by the alkaline comet assay; (e) Quercetin and honey reduced the level of DNA damage induced by OTA in kidneys and liver; (f) Quercetin and honey reduced the level of inflammation induced by OTA in the peritoneal cavity of mice; and (g) honey and quercetin stimulated the non-specific host immune system through increased macrophage activity and by enhancing the local and systemic immunity by increased Bifidobacteria and Lactobacilli counts.
The present study shows that the stimulating effect of honey on colonic probiotic bacteria is more pronounced on the growth of intestinal Bifidobacteria spp. than Lactobacilli spp. (). Increase in probiotic growth, a prebiotic effect attributed to honey, has already been reported in the literature (Ezz El-Arab, Girgis, Hegazy, & Abd El-Khalek, Citation2006; Gobin, Vučković, & Lušić, Citation2014; Rosendale et al., Citation2008). Honey contains many oligosaccharides and low molecular weight polysaccharides likely to resist degradation by host enzymes, and thus is available as a nutrient source for the microflora in the large bowel. In a recent review of functional foods, honey was listed as a source of a functional component in the class/component “prebiotics” (Ezz El-Arab et al., Citation2006; Rosendale et al., Citation2008), which is confirmed by our data.
The decrease in the count of colonic probiotic bacteria after the administration of mycotoxin may be due to the changes in the bacterial surface during the growth phase, as a result of mycotoxin binding (Ezz El-Arab et al., Citation2006; Rosendale et al., Citation2008; Saarela et al., Citation2000). Furthermore, it is important to point out that honey not only increases the colonic probiotic bacteria counts but is also able to reduce pathogen growth in a dose-dependent manner due to its numerous antimicrobial properties (Ezz El-Arab et al., Citation2006; Gobin et al., Citation2014; Oršolić, Citation2009).
Also, our results indicated that quercetin might act as a metabolic prebiotic and exert a significant positive effect on the intestinal environment by modulation of the cecal microflora population. Analysis of the number of colonies of probiotic bacteria showed that the intake of quercetin increases the number of Bifidobacteria in relation to the control (). Improvement of microflora populations with quercetin level is consistent with the results from Marotti, Bonetti, Biavati, Catizone, & Dinelli (Citation2007) and Lee, Jenner, Low, & Lee (Citation2006). They found that selected strains of Bifidobacteria, being active in the biotransformation of flavonoid glycosides present in common bean seeds and seedlings, could improve the nutritional and health properties of flavonoid-based products. On the other hand, unabsorbed polyphenols and their metabolites selectively inhibit the growth of pathogens and stimulate growth of Lactobacilli and Bifidobacteria (Jakesevic et al., Citation2011; Lee et al., Citation2006; Parkar, Stevenson, & Skinner, Citation2008; Puupponen-Pimiä et al., Citation2005; Tzounis et al., Citation2008).
Parkar et al. (Citation2008) demonstrated that several polyphenols might inhibit the growth and adhesion of gut pathogens to a human gut cell line, and enhance the proliferation and adhesion of a probiotic. So, consumption of fruits and vegetables containing these polyphenols, such as citrus and pip fruit, therefore has potential to influence gut microflora and improve intestinal microbial imbalance caused by stress and other factors, thereby promoting gut health in general (Parkar et al., Citation2008; Sengottuvelan & Nalini, Citation2006).
The gut microflora can play a crucial role in the nutritional and health status of the host via modulation of the immune and metabolic functions. Effects on immune function are demonstrated through the increase of hematological parameters () and functional ability of macrophages (). In the present report, the macrophage spreading index was taken as the primary parameter reflecting the degree of macrophage activation. We observed that the macrophage spreading index of peritoneal macrophages was increased in mice treated with honey or QU () suggesting the immunomodulatory activities of honey and QU. Studies by McLoone, Warnock, & Fyfe (Citation2016) and Abuharfeil, Al-Oran, & Abo-Shehada (Citation1999) also reported immunomodulatory activity of honey. The macrophage spreading index was also increased in animals treated with the mycotoxin, possibly as an indicator of inflammatory reactions. Several reports have shown that mycotoxins, such as aflatoxin, ochratoxin, patulin or fumonisin, are able to affect the inflammatory response acting at different levels. They can directly affect the viability of phagocytes (macrophages and neutrophils) and impair the activity or the secretory functions of these cells (Jakesevic et al., Citation2011; Oswald et al., Citation2005). In order to more precisely identify the effect of ochratoxin on the functional ability of macrophages, in future studies we should explore effects of OTA on the secretory ability of macrophages by monitoring production of inflammatory cytokines, nitric oxide and hydrogen peroxide) and the phagocytic ability.
It has been demonstrated that prebiotic ingestion increases the number and metabolic activity of LAB in the colon of humans and animals (Oršolić & Bašić, Citation2008; Saulnier et al., Citation2009). Furthermore, reduced activity of pro-carcinogenic enzymes in humans also was shown as a consequence of prebiotic intake (Bennett & Klich, Citation2003; Oršolić, Citation2009). It is known that OTA can be transformed by hydrolysis, hydroxylation and oxidation into a series of metabolites and that one of the most important metabolization reactions is glucuronidation, mainly in liver microsomes. So, OTA-conjugates have been detected in liver (8–17%) and intestinal tissue (6%) (Li, Marquardt, Frohlich, Vitti, & Crow, Citation1997).
In the present study, QU preparations alone and/or in combination with OTA significantly suppressed bacterial β-glucuronidase activity in feces and cecal digest. This is important because many of the glucuronides are conjugated products of detoxification by the liver and their hydrolysis may liberate free toxins including carcinogens which may then act on the colonocytes or may be absorbed by the intestines (Cencic & Chingwaru, Citation2010; Fusi et al., Citation2010; Salminen et al., Citation2010). The provided data suggest that the inhibition of β-glucuronidase in the intestinal microflora is a major mechanism of the protective effects of quercetin.
Accordingly, QU and dietary preparations with QU may play positive roles in minimizing the risk of colonic diseases by inhibiting the enzyme activity of undesirable intestinal microbiota. Other authors suggest that intestinal polyphenol metabolites are involved in the modulation of enzymes important for the detoxification process and inflammation (Oršolić, Citation2009). Some polyphenol-containing dietary components may also influence bacterial metabolizing enzymes and thus influence overall cancer risk. For example, resveratrol supplementation significantly reduced activities of fecal and host colonic mucosal enzymes, such as β-glucoronidase, β-glucosidase, β-galactosidase, mucinase and nitroreductase activities (Sengottuvelan & Nalini, Citation2006).
It is possible that the decreased activity of toxin release is crucial in reducing the levels of DNA damage in the kidney and liver. Our data demonstrated that oral application of the carcinogens OTA to mice resulted in DNA damage in liver and kidney cells ( and ) and slightly increased the number of inflammatory cells (). The toxic effect of OTA on liver and kidney is evident in the increase in serum parameters such as ALP, ALT, AST, LDH and urea, while decreased levels of serum proteins () indicate changes in renal function (Oršolić et al., Citation2010; Oršolić & Car, Citation2014). However, when OTA was administered in combination with honey or QU, DNA damage was significantly reduced as well as the number of immflamatory cells in the peritoneal cavity, together with pronounced decrease in the activities of all serum parameters. It is possible that some of the above-mentioned explanations, related to the increase of probiotic bacteria and their effect in the detoxification process, enzyme activity and formation of physical barrier to toxins along with antioxidant and anti-inflammatory characteristics of honey and QU, may be crucial protection against OTA-induced toxicity to the kidneys, the liver, the immune system and the body in general (Benkovic et al., Citation2008; Kenjeric et al., Citation2007; Morales et al., Citation2006; Moskaug et al., Citation2004; Oršolić, Citation2009; Oršolić et al., Citation2007, Citation2010, Citation2011; Oršolić & Car, Citation2014; Piljac-Žegarac et al., Citation2009; Sirovina et al., Citation2013). Furthermore, recent literature data show that that endogenous-glucuronidase in the tissues of humans and animals (pig, rat and mouse) hydrolyzes flavonoid glucuronides to their aglycones during the tissue extraction, leading to a higher antioxidant and anti-inflammatory activity, which may be the mechanism responsible for the anti-inflammatory activity of dietary flavonoids within the inflammation sites.
Interestingly, our data are in an agreement with those of other authors who demonstrate that several strains of Lactobacilli and Bifidobacteria were effective in protecting rats from DNA damage, as measured by the comet assay (Pool-Zobel et al., Citation1996).
The mechanisms involved in the cellular toxicity of OTA on liver and kidney are still unresolved; it is assumed that its toxicity may be the result of three major effects:(a) inhibition of ATP synthesis; (b) inhibition of protein synthesis; and (c) enhanced lipid peroxidation (Limonciel & Jennings, Citation2014; Malir et al., Citation2013; Ostry et al., Citation2013; Pfohl-Leszkowicz & Manderville, Citation2007, Citation2012). In addition, some epigenetic factors are also included in the OTA toxicity such as Nrf2 inhibition (Limonciel & Jennings, Citation2014; Malir et al., Citation2013). Furthermore, activation of Nrf2 by pre-incubation with the quercetin prevented OTA-induced cell death (Morales et al., Citation2006; Ramyaa, Krishnaswamy, & Padma, Citation2014), which can be a possible mechanism in our conditions. The effect of polyphenolic/flavonoid components, including quercetin, on epigenetic and genetic processes, mechanisms of DNA damage repair, genomic stability, down-regulation of NF-κB and COX-2 and inhibition of carcinogenesis is also confirmed (Benkovic et al., Citation2008; Morales et al., Citation2006; Moskaug et al., Citation2004; Oršolić, Citation2009, Citation2010; Oršolić et al., Citation2007, Citation2010, Citation2011; Oršolić & Car, Citation2014; Piljac-Žegarac et al., Citation2009; Ramyaa et al., Citation2014; Sirovina et al., Citation2013).
Because previous studies implicate oxidative pathways in the toxicity of OTA, compounds with antioxidant properties could be potentially efficacious in protecting against the toxic effects of this mycotoxin, as is confirmed by our results ( and ). Flavonoids have high antioxidant activities as free radical scavengers and as inhibitors of enzymes generating reactive oxygen species (Benkovic et al., Citation2008; Kenjeric et al., Citation2007; Morales et al., Citation2006; Moskaug et al., Citation2004; Oršolić, Citation2009, Citation2010; Oršolić et al., Citation2007, Citation2010, Citation2011; Piljac-Žegarac et al., Citation2009; Sirovina et al., Citation2013). Flavonoids may preserve kidney and liver cell function by reducing ochratoxin oxidative stress-induced tissue damage and therefore protect against the progression of carcinogenesis ( and ).
Many authors have demonstrated that honey serves as a source of natural antioxidants (Kenjeric et al., Citation2007; Oršolić, Citation2009; Piljac-Žegarac et al., Citation2009; Tomas-Barberan et al., Citation2001) and reduces the risk of heart disease, cancer, cataracts and inflammatory processes (Oršolić, Citation2009). Since some of these diseases are a consequence of oxidative damage, it seems that part of the therapeutic properties of honey are due to its antioxidant capacity, especially a high content of phenolic components. In addition, a possible synergistic effect between other minor honey components could also be involved. Al-Waili (Citation2003) showed that honey increased the amount and activity of antioxidant agents such as vitamin C by 47%, beta-carotene by 3%, uric acid by 12% and glutathione reductase by 7%. These compounds reduce the extent of lipid peroxidation, which is a consequence of toxic metabolites generated by ochratoxin. It is possible that the antioxidant capacity of honey is a result of the combined activity of a wide range of compounds including phenolics, peptides, organic acids, enzymes, Maillard reaction products and possibly other minor components and that the phenolic compounds contribute significantly to the antioxidant capacity of honey but are not solely responsible for it.
According to the results of this study, it can be concluded that several natural components of the diet including honey and QU exhibit potent antigenotoxic activity and bifidogenic effect, and thus might partly counteract OTA toxicity. The protective effects of QU against OTA-induced intestinal toxicity may be due, at least in part, to the inhibition of β-glucuronidase in the intestinal microflora. This positive effect of quercetin may also refer to the gut, liver and kidney because a close anatomical and functional relationship between these organs exists. It seems that various co-operative and synergistic action mechanisms of the probiotic bacteria, QU and various polyphenols present in honey may lead to the protection of the whole organism against OTA-induced tissue damage.
Authorship
N.O. – conceived and designed the study, conducted experiments resulting in the data, performed the experiments, participated in the data acquisition, analyzed and interpreted the data, obtained the funding, supervised research personnel, performed statistical analysis and wrote the manuscript and made critical revision of the manuscript for important intellectual content. M.J.J. and S.T. – participated in the entire data acquisition.
Notes on contributors
Nada Oršolić is a Ph.D. at Faculty of Science, University of Zagreb. Presently, she is Full Professor and working as a teacher and researcher at the Department of Animal Physiology. Her interest is in Cancer immunology, chemoprevention, chemotherapy, and radioprotection with natural products, free radical biology and cancer biochemistry, nutrition and cancer, flavonoids and anticancer drug design, Cytotoxic and genotoxic effects of inhalation anaesthetics alone or in combination with chemotherapy and radiotherapy and protective effects of polyphenol/flavonoids compounds against oxidative stress in different chronic diseases such as cancer, diabetes, osteoporosis, inflammation and autoimmune diseases. She is a lecturer of the following courses at the Department of Animal Physiology: Animal physiology, Experimental animals in biological research, Immunology, Immunology and immunogenetics, Comparative immunology, General oncology, Molecular oncology, Immunobiology of tumour and metastasis, Tumour biology, Physiology of oxidative stress in human and animals, Physiological mechanism in toxicology, Chemoprevention and biotherapy of tumour, Mutagens and antimutagens, Animal models in rodents in experimental oncology.
Maja Jazvinšćak Jembrek is a Ph.D. at Rudjer Bošković Institute (Zagreb, Croatia). Her research interest includes protective effects of phytonutrients against oxidative stress, particularly on neuronal cells. She is also working as a teacher at the Department of Psychology of Catholic University of Croatia.
Svjetlana Terzić is a Ph.D. on veterinary microbiology and immunology. She is head of Laboratory for veterinary medical product of the Croatian Veterinary Institute. Her interest is in animal health and veterinary medicinal product.
Additional information
Funding
References
- Abuharfeil, N., Al-Oran, R., & Abo-Shehada, M. (1999). The effect of bee honey on the proliferative activity of human B-and T-lymphocytes and the activity of phagocytes. Food and Agricultural Immunology, 11(2), 169–177. doi: 10.1080/09540109999843
- Al-Waili, N. S. (2003). Effects of daily consumption of honey solution on hematological indices and blood levels of minerals and enzymes in normal individuals. Journal of Medicinal Food, 6, 135–140. doi: 10.1089/109662003322233549
- Benkovic, V., Horvat Knezevic, A., Dikic, D., Lisicic, D., Orsolic, N., Basic, I., … Kopjar, N. (2008). Radioprotective effects of propolis and quercetin gamma-irradiated mice evaluated by the alkaline comet assay. Phytomedicine, 15, 851–858. doi: 10.1016/j.phymed.2008.02.010
- Bennett, J. W., & Klich, M. (2003). Mycotoxins. Clincal Microbiology Reviews, 16(3), 497–516. doi: 10.1128/CMR.16.3.497-516.2003
- Boots, A. W., Haenen, G. R., & Bast, A. (2008). Health effects of quercetin: From antioxidant to nutraceutical. European Journal Pharmacology, 585(2–3), 325–337. doi: 10.1016/j.ejphar.2008.03.008
- Cencic, A., & Chingwaru, W. (2010). The role of functional foods, nutraceuticals, and food supplements in intestinal health. Nutrients, 2(6), 611–625. doi: 10.3390/nu2060611
- Davis, C. D., & Milner, J. A. (2009). Gastrointestinal microflora, food components and colon cancer prevention. Journal of Nutritional Biochemistry, 20(10), 743–752. doi: 10.1016/j.jnutbio.2009.06.001
- De Petrino, S. F., De Jorrat, M. E. B. B., De Budeguer, M. V. G., & Perdigón, G. (1997). Influence of the oral administration of different lactic acid bacteria onintestinal microflora and igA-secreting cells in mice treated with ampicillin. Food and Agricultural Immunology, 9(4), 265–275. doi: 10.1080/09540109709354957
- Djouzi, Z., & Andrieux, C. (1997). Compared effects of three oligosaccharides on metabolism of intestinal microflora in rats inoculated with a human faecal flora. British Journal of Nutrition, 78, 313–324. doi: 10.1079/BJN19970149
- Ezz El-Arab, A. M., Girgis, S. M., Hegazy, E. M., & Abd El-Khalek, A. B. (2006). Effect of dietary honey on intestinal microflora and toxicity of mycotoxins in mice. BMC Complementary and Alternative Medicine, 6, 1–13. doi: 10.1186/1472-6882-6-6
- Fusi, E., Rebucci, R., Pecorini, C., Campagnoli, A., Pinotti, L., Saccone, F., … Baldi, A. (2010). Alpha-tocopherol counteracts the cytotoxicity induced by ochratoxin A in primary porcine fibroblasts. Toxins, 2, 1265–1278. doi: 10.3390/toxins2061265
- Gobin, I., Vuckovic, D., & Luic, D. (2014). Antibacterial properties of honey. Medicina Fluminensis, 50(2), 150–157.
- Greiner, A. K., Papineni, R. V., & Umar, S. (2014). Chemoprevention in gastrointestinal physiology and disease. Natural products and microbiome. American Journal of Physiology-Gastrointestinal and Liver Physiology, 307(1), G1–G15. doi: 10.1152/ajpgi.00044.2014
- Gupta, M., Bandopadhyay, S., Paul, B., & Majumder, S. K. (1979). Hematological changes produced in mice by ochratoxin A. Toxicology, 14(1), 95–98. doi: 10.1016/0300-483X(79)90095-7
- Haskard, C. A., El-Nezami, H. S., Kankaanpaa, P. E., Salminen, S., & Aholmas, J. T. (2001). Surface binding of aflatoxin B1 by lactic acid bacteria. Applied and Environmental Microbiology, 67, 3086–3091. doi: 10.1128/AEM.67.7.3086-3091.2001
- Hassan, Z. U., Khan, M. Z., Saleemi, M. K., Khan, A., Javed, I., & Noreen, M. (2012). Immunological responses of male white leghorn chicks kept on ochratoxin A (OTA)-contaminated feed. Journal of Immunotoxicology, 9(1), 56–63. doi: 10.3109/1547691X.2011.627393
- Jakesevic, M., Aaby, K., Borge, G. I., Jeppsson, B., Ahrné, S., & Molin, G. (2011). Antioxidative protection of dietary bilberry, chokeberry and Lactobacillus plantarum HEAL19 in mice subjected to intestinal oxidative stress by ischemia-reperfusion. BMC Complementary and Alternative Medicine, 11, 401. doi: 10.1186/1472-6882-11-8
- Juśkiewicz, J., Zary-Sikorska, E., Zduńczyk, Z., Król, B., Jarosławska, J., & Jurgoński, A. (2012). Effect of dietary supplementation with unprocessed and ethanol-extracted apple pomaces on caecal fermentation, antioxidant and blood biomarkers in rats. British Journal of Nutrition, 107(8), 1138–1146. doi: 10.1017/S0007114511004144
- Kamp, H. G., Eisenbrand, G., Janzowski, C., Kiossev, J., Latendresse, J. R., Schlatter, J., & Turesky, R. J. (2005). Ochratoxin A induces oxidative DNA damage in liver and kidney after oral dosing to rats. Molecular Nutrition & Food Research, 49(12), 160–167. doi: 10.1002/mnfr.200500124
- Kenjeric, D., Mandic, M. L., Primorac, L., Bubalo, D., & Perl, A. (2007). Flavonoid profile of Robinia honeys produced in Croatia. Food Chemistry, 102, 683–690. doi: 10.1016/j.foodchem.2006.05.055
- Kocic, G., Cukuranovic, J., Stoimenov, T. J., Cukuranovic, R., Djordjevic, V., Bogdanovic, D., & Stefanovic, V. (2014). Global and specific histone acetylation pattern in patients with Balkan endemic nephropathy, a worldwide disease. Renal Failure, 21, 1–5.
- Lee, H. C., Jenner, A. M., Low, C. S., & Lee, Y. K. (2006). Effect of tea phenolics and their aromatic fecal bacterial metabolites on intestinal microbiota. Research in Microbiology, 157, 876–884. doi: 10.1016/j.resmic.2006.07.004
- Li, S., Marquardt, R. R., Frohlich, A. A., Vitti, T. G., & Crow, G. (1997). Pharmacokinetics of ochratoxin A and its metabolites in rats. Toxicology and Applied Pharmacology, 145, 82–90. doi: 10.1006/taap.1997.8155
- Limonciel, A., & Jennings, P. (2014). A review of the evidence that ochratoxin A is an Nrf2 inhibitor: Implications for nephrotoxicity and renal carcinogenicity. Toxins (Basel), 6(1), 371–379. doi: 10.3390/toxins6010371
- Malir, F., Ostry, V., & Novotna, E. (2013). Toxicity of the mycotoxin ochratoxin A (OTA) in the light of recent data. Toxin Reviews, 32, 19–33. doi: 10.3109/15569543.2013.782504
- Manach, C., Scalbert, A., Morand, C., Rémésy, C., & Jiménez, L. (2004). Polyphenols: Food sources and bioavailability. The American Journal Clinical Nutrition, 79(5), 727–747.
- Marin-Kuan, M., Cavin, C., Delatour, T., & Schilter, B. (2008). Ochratoxin A carcinogenicity involves a complex network of epigenetic mechanisms. Toxicon, 52(2), 195–202. doi: 10.1016/j.toxicon.2008.04.166
- Marotti, I., Bonetti, A., Biavati, B., Catizone, P., & Dinelli, G. (2007). Biotransformation of common bean (Phaseolus vulgaris L.) flavonoid glycosides by bifidobacterium species from human intestinal origin. Journal of Agricultural and Food Chemistry, 55, 3913–3919. doi: 10.1021/jf062997g
- McLoone, P., Warnock, M., & Fyfe, L. (2016). Honey: An immunomodulatory agent for disorders of the skin. Food and Agricultural Immunology, 27(3), 338–349. doi: 10.1080/09540105.2015.1104653
- Morales, A. I., Vicente-Sánchez, C., Jerkic, M., Santiago, J. M., Sánchez-González, P. D., Pérez-Barriocanal, F., & López-Novoa, J. M. (2006). Effect of quercetin on metallothionein, nitric oxide synthases and cyclooxygenase-2 expression on experimental chronic cadmium nephrotoxicity in rats. Toxicology and Applied Pharmacology, 210, 128–135. doi: 10.1016/j.taap.2005.09.006
- Moskaug, J. Ø., Carlsen, H., Myhrstad, M., & Blomhoff, R. (2004). Molecular imaging of the biological effects of quercetin and quercetin-rich foods. Mechanisms of Ageing and Development, 125, 315–324. doi: 10.1016/j.mad.2004.01.007
- Myhrstad, M. C., Carlsen, H., Nordström, O., Blomhoff, R., & Moskaug, J. Ø. (2002). Flavonoids increase the intracellular glutathione level by transactivation of the gamma-glutamylcysteine synthetase catalytical subunit promoter. Free Radical Biology and Medicine, 32, 386–393. doi: 10.1016/S0891-5849(01)00812-7
- Oršolić, N., Benković, V., Horvat-Knežević, A., Kopjar, N., Kosalec, I., Bakmaz, M., … Bašić, I. (2007). Assessment by survival analysis of the radioprotective properties of propolis and its polyphenolic compounds. Biological and Pharmaceutical Bulletin, 30, 946–951. doi: 10.1248/bpb.30.946
- Oršolić, N., Benković, V., Lisičić, D., Đikić, D., Erhardt, J., & Horvat-Knežević, A. (2010). Protective effects of propolis and related polyphenolic/flavonoid compounds against toxicity induced by irinotecan. Medical Oncology, 27(4), 1346–1358. doi: 10.1007/s12032-009-9387-5
- Oršolić, N., & Car, N. (2014). Quercetin and hyperthermia modulate cisplatin-induced DNA damage in tumor and normal tissues in vivo. Tumour Biology, 35(7), 6445–6454. doi: 10.1007/s13277-014-1843-y
- Oršolić, N., Gajski, G., Garaj-Vrhovac, V., Dikić, D., Prskalo, Z. Š., & Sirovina, D. (2011). DNA-protective effects of quercetin or naringenin in alloxan-induced diabetic mice. European Journal of Pharmacology, 656(1-3), 110–118. doi: 10.1016/j.ejphar.2011.01.021
- Oršolić, N. (2009). Bee honey and cancer. Journal of ApiProduct and ApiMedical Science, 1(4), 93–103. doi: 10.3896/IBRA.4.01.4.01
- Oršolić, N. (2010). A review of propolis antitumor action in vivo and in vitro. Journal of ApiProduct and ApiMedical Science, 2(1), 1–20. doi: 10.3896/IBRA.4.02.1.01
- Orolic, N., & Baic, I. (2008). Recent progress in medicinal plants. In J. N. Govil, & V. K. Singh (Eds.), Honey bee products and their polyphenolic compounds in treatment of diabetes. Phytopharmacology and therapetutic values IV (Vol. 22, pp. 455–553). New Delhi: Studium Press, LLC.
- Ostry, V., Malir, F., & Ruprich, J. (2013). Producers and important dietary sources of ochratoxin A and citrinin. Toxins (Basel), 5(9), 1574–1586. doi: 10.3390/toxins5091574
- Oswald, I. P., Marin, D. E., Bouhet, S., Pinton, P., Taranu, I., & Accensi, F. (2005). Immunotoxicological risk of mycotoxins for domestic animals. Food Additves & Contaminants, 22(4), 354–360. doi: 10.1080/02652030500058320
- Parkar, S. G., Stevenson, D. E., & Skinner, M. A. (2008). The potential influence of fruit polyphenols on colonic microflora and human gut health. International Journal of Food Microbiology, 124(3), 295–298. doi: 10.1016/j.ijfoodmicro.2008.03.017
- Pfohl-Leszkowicz, A., & Manderville, R. A. (2007). Ochratoxin A: An overview on toxicity and carcinogenicity in animals and humans. Molecular Nutrition & Food Research, 51, 61–99. doi: 10.1002/mnfr.200600137
- Pfohl-Leszkowicz, A., & Manderville, R. A. (2012). An update on direct genotoxicity as a molecular mechanism of ochratoxin A carcinogenicity. Chemical Research in Toxicology, 25, 252–262. doi: 10.1021/tx200430f
- Piljac-Žegarac, J., Stipčević, T., & Belščak, A. (2009). Antioxidant properties and phenolic content of different floral origin honeys. Journal of ApiProduct and ApiMedical Science, 1(2), 43–50. doi: 10.3896/IBRA.4.01.2.04
- Pirker, A., Stockenhuber, A., Remely, M., Harrant, A., Hippe, B., Kamhuber, C., … Haslberger, A. G. (2013). Effects of antibiotic therapy on the gastrointestinal microbiota and the influence of Lactobacillus casei. Food and Agricultural Immunology, 24(3), 315–330. doi: 10.1080/09540105.2012.689816
- Pool-Zobel, B. L., Neudecker, C., Domizlaff, I., Ji, S., Schillinger, U., Rumney, C., … Rowland, I. (1996). Lactobacillus- and bifidobacterium-mediated antigenotoxicity in the colon of rats. Nutrition and Cancer, 26, 365–380. doi: 10.1080/01635589609514492
- Puupponen-Pimiä, R., Nohynek, L., Hartmann-Schmidlin, S., Kähkönen, M., Heinonen, M., Määttä-Riihinen, K., & Oksman-Caldentey, K.-M. (2005). Berry phenolics selectively inhibit the growth of intestinal pathogens. Journal of Applied Microbiology, 98, 991–1000. doi: 10.1111/j.1365-2672.2005.02547.x
- Rafter, J. (2002). Lactic acid bacteria and cancer: Mechanistic perspective. British Journal of Nutrition, 88(Suppl. 1), S89–94. doi: 10.1079/BJN2002633
- Ramyaa, P., Krishnaswamy, R., & Padma, V. V. (2014). Quercetin modulates OTA-induced oxidative stress and redox signalling in HepG2 cells – Up regulation of Nrf2 expression and down regulation of NF-κB and COX-2. Biochimica and Biophysica Acta, 1840(1), 681–92. doi: 10.1016/j.bbagen.2013.10.024
- Rosendale, D. I., Maddox, I. S., Miles, M. C., Rodier, M., Skinner, M., & Sutherland, J. (2008). High-throughput microbial bioassays to screen potential New Zealand functional food ingredients intended to manage the growth of probiotic and pathogenic gut bacteria. International Journal of Food Science & Technology, 43, 2257–2267. doi: 10.1111/j.1365-2621.2008.01863.x
- Saarela, M., Lahteenmaki, L., Crittenden, R., Salminen, S., & Mattila-Sandholm, T. (2000). Gut bacteria and health foods – The European perspective. International Journal of Food Microbiology, 278, 99–117.
- Salminen, S., Nybom, S., Meriluoto, J., Collado, M. C., Vesterlund, S., & El-Nezami, H. (2010). Interaction of probiotics and pathogens-benefits to human health? Current Opinion in Biotechnology, 21(2), 157–167. doi: 10.1016/j.copbio.2010.03.016
- Saulnier, D. M., Spinler, J. K., Gibson, G. R., & Versalovic, J. (2009). Mechanisms of probiosis and prebiosis: considerations for enhanced functional foods. Current Opinion in Biotechnology, 20(2), 135–141. doi: 10.1016/j.copbio.2009.01.002
- Sengottuvelan, M., & Nalini, N. (2006). Dietary supplementation of resveratrol suppresses colonic tumour incidence in 1,2-dimethylhydrazine-treated rats by modulating biotransforming enzymes and aberrant crypt foci development. British Journal of Nutrition, 96, 145–153. doi: 10.1079/BJN20061789
- Singh, N. P., McCoy, M. T., Tice, R. R., & Schneider, E. L. (1988). A simple technique for quantitation of low levels of DNA damage in individual cells. Experimental Cell Research, 175, 184–191. doi: 10.1016/0014-4827(88)90265-0
- Singleton, V. L., Orthofer, R., & Lamuela-Raventos, R. M. (1999). Analysis of total phenols and other oxidation substrates and antioxidants by means of Folin-Ciocalteu reagent. Methods in Enzymology, 299, 152–178. doi: 10.1016/S0076-6879(99)99017-1
- Sirovina, D., Oršolić, N., Zovko Končić, M., Kovačević, G., Benković, V., & Gregorović, G. (2013). Quercetin vs chrysin: Effect on liver histopathology in diabetic mice. Human & Exsperimental Toxicology, 32, 1058–1066. doi: 10.1177/0960327112472993
- Tomas-Barberan, F. A., Martos, I., Ferreres, F., Radovic, B. S., & Anklam, E. (2001). HPLC flavonoid profiles as markers for the botanical origin of European unifloral honeys. Journal of the Science of Food and Agriculture, 81(5), 485–496. doi: 10.1002/jsfa.836
- Tzounis, X., Vulevic, J., Kuhnle, G. G. C., George, T., Leonczak, J., Gibson, G. R., & Spencer, J. P. E. (2008). Flavonol monomer-induced changes to the human faecal microflora. British Journal of Nutrition, 99, 782–792. doi: 10.1017/S0007114507853384
- Yao, L., Jiang, Y., Singanusong, R., Datta, N., & Raymont, K. (2004). Phenolic acids and abscisic acid in Australian eucalyptus honeys and their potential for floral authentication. Food Chemistry, 86, 169–177. doi: 10.1016/j.foodchem.2003.08.013
- Yao, P., Nussler, A., Liu, L., Hao, L., Song, F., Schirmeier, A., & Nussler, N. (2007). Quercetin protects human hepatocytes from ethanol-derived oxidative stress by inducing heme oxygenase-1 via the MAPK/Nrf2 pathways. Journal of Hepatology, 47, 253–261. doi: 10.1016/j.jhep.2007.02.008