ABSTRACT
An effective double antibody sandwich ELISA (DAS-ELISA) method based on monoclonal (mAb) and chicken egg yolk IgY antibodies was developed to determine phosvitin (PV) content in therapeutic and functional products. Leghorn laying hens were immunized with purified PV to produce anti-PV IgY antibody in the egg yolk. High anti-PV IgY titer obtained from the egg yolks collected during 4–10 weeks of the immunization period contained approximately 6.2% of specific anti-PV IgY in total IgY. The PV detection range of the DAS-ELISA and biotinylated DAS-ELISA was 16.8–90 and 7.5–40 ng/mL, respectively. However, biotinylated DAS-ELISA was the better method for PV quantification in terms of accuracy and sensitivity. This highly efficient PV detection method may recuperate the performance of the existing protein assay methods as well as facilitate future research on PV bioactivities and applications.
Introduction
Phosvitin (PV), one of the highly phosphorylated proteins in nature, is produced by proteolytic cleavage of chicken vitellogenin (a metal-binding protein), which is disseminated in the hen blood and picked up by developing oocytes (Richards, Citation1997). Vitellogenin is cleaved into lipovitellin and PV yolk proteins, sometimes during or after transport into the oocyte (Yamamura et al., Citation1995).
PV accounts for 60% of total egg yolk phosphoproteins and contains about 90% of the egg yolk phosphorus (Xu, Katayama, & Mine, Citation2007). The numerous phosphorylated serine residues in PV exhibit a strong affinity for divalent metals such as iron, calcium and magnesium (Grizzuti & Perlmann, Citation1973), which helps in preventing metal-induced lipid oxidation in lipid-containing food and biological systems (Lu & Baker, Citation1986). PV has also displayed strong antimicrobial and emulsifying properties (Gujral, Yoo, Bamdad, Suh, & Sunwoo, Citation2017; Khan, Babiker, Azakami, & Kato, Citation1998). It is considered that the emulsifying activity of PV is better than that of bovine serum albumin, β-casein and soy protein (Chung & Ferrier, Citation1992; Khan et al., Citation1998).
In recent years, PV has gained the attention of researchers, scientists and industries as an ingredient for food and nutraceutical formulations, due to its phosphopeptide content and unique bioactive properties (Samaraweera, Zhang, Lee, & Ahn, Citation2011). PV is considered as an efficient source of bioactive phosphopeptides, however, the high cost along with complicated detection and purification methods hinders its applications, particularly in the food industry. Also, there is a huge potential of PV applications in the cosmetic industry as naturally derived antioxidant and anti-aging copper-binding peptides. To the best of our knowledge, there is no well-established method for direct detection or quantification of PV. Currently available methods for PV detection include only an indirect determination of phosphate content and semi-quantitative assessment of the phosphorylation level. Accordingly, an efficient, precise and reproducible method for quantitation of PV is necessary for the development of extraction and purification techniques.
Antibodies, being highly specific in nature, are persuasive candidates for PV protein identification (Jensenius, Andersen, Hau, Crone, & Koch, Citation1981). Antibodies are typically produced by immunization of animals, mostly mammals. However, mammalian antibodies are produced by invasive means in limited quantities, and their purification is time-consuming, as well as expensive. As an alternative, the use of avian antibodies has increased spectacularly to replace mammalian antibodies. The major advantage of avian antibodies is ease of harvest from their eggs, instead of serum. Also, an egg-laying hen antibody productivity is much higher than that of a similar-sized mammal (Hau & Hendriksen, Citation2005). Today, hens are recognized as an expedient and economical source of antibodies.
Our aim in this study is to develop an accurate ELISA method based on monoclonal (mAb) and chicken egg yolk IgY polyclonal antibodies for the quantitation of microgram to nanogram levels of PV. The quantitation method developed here, may be applied in studies on PV for its accurate quantification and to further improve the detection methods of other challenging proteins.
Materials and methods
Materials
PV (from chicken egg yolk), phosphatase, Freund’s incomplete adjuvant, purified chicken IgG, rabbit anti-chicken IgG, rabbit anti-chicken IgG conjugated with horseradish peroxidase and streptavidin-HRPO were purchased from Sigma (St. Louis, MO, USA). Biotinaimdohexanoic acid-3-sulfo-N-hydroxysuccinimide ester (NHS-PEO4-Biotin), Slide-A-Lyzer®, 1-Step™ ABTS (2,2′-Azinobis [3-ethylbenzothiazoline-6-sulfonic acid]) substrate solution and tetramethylbenzidine (TMB) were purchased from Thermo Fisher Scientific (Burlington, ON, Canada) and KPL (Gaithersburg, MD, USA), respectively. Anti-PV (PPP-6) mAb was obtained from Myungji University (Yongin, Korea). Bio-Rad protein assay kit, glycine, Precision Plus Protein Standard, sodium dodecyl sulfate (SDS), Mini-PROTEAN® TGX™ Any kD precast gels in Tris-Glycine-SDS buffer was purchased from Bio-Rad Laboratory (Mississauga, ON, Canada). All analytical grade chemicals and solvents were from commercial sources.
Experimental design
The schematic representation of the development of an ELISA-based detection system for PV is demonstrated in .
Production of anti-PV polyclonal IgY
Production of polyclonal IgY directed against PV was followed by chicken immunization. The Sigma PV was dephosphorylated with acid phosphatase as described by Miller, Benore-Parsons, and White (Citation1982). The dephosphorylated PV was suspended in sterilized PBS at a concentration of 100 μg/mL and emulsified with an equal volume of Freund’s incomplete adjuvant. Twelve 23-weeks-old Single Comb White Leghorn chickens were intramuscularly injected with the emulsified saline with or without PV at four different sites (0.25 mL per site) of breast muscles (two sites per left or right breast muscle), as previously described (Sunwoo, Gujral, Lutz, & Suresh, Citation2012). Two booster immunizations were given at the end of 2nd and 8th week after the initial immunization. Eggs were collected daily and stored at 4°C until the extraction of the antibodies.
Purification of anti-PV IgY
The egg yolk from immunized hens was physically separated from egg white and mixed gently with 8 volumes of cold distilled water (acidified with 0.1 M HCl to give a pH of 4.0) to avoid possible disruptions of egg yolk granules due to the presence of high concentrations of acid. Cold acidified distilled water (pH 2.0) was then added to make the final dilution of 1:10. After mixing well, the mixture was adjusted to a pH of 5.0–5.2 and incubated at 4°C for 12 h. Water-soluble fraction (WSF) was obtained by centrifugation (3125 × g) at 4°C for 20 min. The supernatant was collected as the IgY-rich WSF and titrated by Indirect ELISA (described in the following section) using Sigma PV as coating antigen. The fractions found to have high titers, particularly the fractions collected during weeks 4–10 of the immunization period were further purified by ammonium sulfate (40%) followed by Sephacryl S-300 gel filtration column (GE Healthcare Bio-Sciences Corp, Piscataway, NJ, USA) (Ishikawa, Tamaki, Arihara, & Itoh, Citation2007). Control IgY antibody was extracted from eggs obtained from non-immunized chickens.
Detection of anti-PV IgY by Indirect ELISA
Microtiter plates were coated with 100 μL per well of PV in carbonate-bicarbonate buffer (0.05 M, pH 9.6) at a concentration of 8 μg/mL and incubated at 37°C for 1 h. The plates were then washed four times with PBS containing 0.05% Tween 20 (PBS-Tween). After washing, 100 μL of 1% BSA solution (w/v) in PBS-Tween was added to each well, and the plates were incubated at 37°C for 30 min. The BSA solution was then discarded, and the wells were washed. Egg yolk WSF (diluted 1:1000 in PBS-Tween) containing specific IgY against PV or non-immunized egg yolk IgY antibody (control) was added to each well (100 μL per well), and the plates were further incubated at 37°C for 1 h. After washing the plates, 100 μL of rabbit anti-chicken IgG conjugated with horseradish peroxidase (HRPO, diluted 1:5000 in PBS-Tween) was added to each well and incubated at 37°C for 1 h. The plates were washed again with PBS-Tween, followed by the addition of 100 μL of 1-Step™ ABTS Substrate Solution without stop solution. OD405 was taken after 30 min using an ELISA Vmax kinetic microplate reader (Molecular Devices Corp, Sunnyvale, CA, USA). The optical density value for antibody activity was determined by subtracting the value of the control antibody from the value for specific antibody.
Total IgY concentration
To quantify the total IgY concentration purified from egg yolks, ELISA was performed as mentioned in the section on titer of anti-PV IgY by Indirect ELISA. A microtiter plate was coated with 100 μL of rabbit anti-chicken IgG per well at a final concentration of 20 μg/mL. Samples of the PV-specific IgY were diluted 1:10,000 in PBS. Specific and non-specific IgY were reconstituted and serially diluted in PBS. Two-fold serial dilutions of purified chicken IgG in PBS (0.5–0.031 μg/mL) were used as the reference antibody to prepare a standard curve. Total and specific IgY concentrations were determined using the five-point standard curve.
Total protein assay
The Bio-Rad protein assay, based on the method of Bradford, was performed using purified chicken IgY (1 mg protein/mL) as the reference protein. Serial dilutions of the purified IgY, and the reference protein in PBS (0.5–0.0625 mg/mL) were analyzed using the microtiter plate. OD595 was taken after 5 min using an ELISA Vmax kinetic microplate reader.
Electrophoresis and Western blot
Sigma PV samples were run on Mini-PROTEAN® TGX™ Any kD Precast Gels in Tris-Glycine-SDS buffer and stained with a solution of 0.05% Coomassie Blue R-250, 0.1 M aluminum nitrate, 25% isopropanol, 10% acetic acid and 1% TritonX-100. Gels were destained with 7% acetic acid. Bio-rad Precision Plus Protein Standards were loaded as a molecular weight marker at a constant voltage (100 V). Images of gels were scanned in the Bio-Rad Gel Doc™ EZ Imager (Bio-Rad Laboratory, Mississauga, ON, Canada).
For Western blot assay, the gel was electrophoretically transferred to nitrocellulose membrane in Tris-borate electrophoresis buffer without SDS at 40 V for 2 h. Nitrocellulose membrane was then soaked in 2% BSA in PBS (pH 7.2) for 1 h at room temperature. After washing with PBS (three times for 5 min each), the nitrocellulose membrane was incubated with anti-PV IgY antibodies (in PBS containing 1% BSA) for 1 h at room temperature. The incubation was followed by washing in PBS and 1 h incubation with rabbit anti-chicken IgG conjugated with HRPO (diluted 1:3000 in PBS-Tween). Finally, the samples were treated with 0.05% diaminobenzidine tetra-hydrochloride in PBS containing hydrogen peroxide (0.01%, w/v) and cobalt chloride (0.033%, w/v) for 5 min to develop the color. Stained blots were then washed five times with water and dried.
Double antibody sandwich ELISA for PV quantification
A microtiter plate was coated with 100 µL of anti-PV mAb (PPP-6, 1 µg/mL) in 0.05 M carbonate buffer, pH 9.6, at 4°C overnight. Unbound sites on the microtiter plate binding were blocked with 120 µL of 3% BSA for 45 min. After each step the plate was washed four times with PBS-Tween. The PV was serially diluted from 0.7 to 180 µg/mL in PBS, pH 7.0 (containing 0.05% Tween 20) and added to each well, and then the plate was incubated at 37°C for 1 h. The plate was washed, and 100 μL of the anti-PV IgY (1 µg/mL) was added to each well and incubated at 37°C for 1 h. After incubation, the plate was washed, and rabbit anti-chicken IgG conjugated to HRPO was added and incubated at 37°C for 45 min. TMB substrate was added and OD at 650 nm was taken after 10 min using an ELISA Vmax kinetic microplate reader. The antibody titer was determined by subtracting the value of the control antibody from that of specific antibody. The schematic representation of double antibody sandwich ELISA (DAS-ELISA) steps is shown in (A).
Biotinylated DAS-ELISA for PV quantification
Anti-PV IgY polyclonal antibodies were biotinylated using long arm NHS-PEO4-Biotin. One mg of anti-PV IgY in PBS (pH 7.2) was added to 20 μL of long chain biotin (3 mg/mL) and then incubated at room temperature for 1 h. A 10 μL volume glycine (100 μg/μL) was added and the solution kept on a shaker for 10 min. The solution was then dialyzed in a Slide-A-Lyzer against PBS (pH 7.2) overnight at 4°C.
Except indicated otherwise, the plates were washed four times with PBS-Tween between each step. A microtiter plate was coated with 100 µl of anti-PV mAb (PPP-6, 1 µg/mL) in 0.05 M carbonate buffer, pH 9.6, at 4°C overnight. Unbound sites on the microtiter plate were blocked with 120 µL of 3% BSA for 45 min. The Sigma PV was serially diluted from 0.3 to 160 ng/mL in PBS, pH 7.0, containing 0.05% Tween 20 and added to each well and then incubated at 37°C for 1 h. The plate was washed, and 100 μL of the biotinylated anti-PV IgY (1 µg/mL) was added to each well and incubated at 37°C for 1 h. After incubation, the plate was washed and streptavidin-HRPO was added and incubated at 37°C for 45 min. TMB substrate was added and OD650 was taken after 10 min using an ELISA Vmax kinetic microplate reader. The antibody titer was determined by subtracting the value of the control antibody from that of specific antibody. The experimental steps of DAS-ELISA are illustrated in (B).
Validation of the assay
The DAS-ELISA and biotinylated DAS-ELISA methods developed were validated by determining the specificity, accuracy, precision and sensitivity (US department of Health and Human Services Food and Drug Administration (FDA), Center for Drug evaluation and research (CDER), Center for Veterinary Medicine (CVM). Guidance, September Citation2013). To determine the accuracy, recovery studies were performed. A saline solution was spiked with Sigma PV standard at levels of 1, 10, 50 and 100 µg/mL sample in a total volume of 10 mL. For the determination of recovery rates, the extractions were done in triplicates and analyzed by the DAS-ELISA and biotinylated DAS-ELISA. Intra-assay (within plate) precision was determined as the mean coefficients of variation (CV) based on five replicates per day for 3 days. Inter-assay (between plates) precision was also determined as the mean CV by triplicate analyses on five plates per day for 3 days. The accuracy and precision determined at each concentration level should not exceed 15% of the CV.
To determine the sensitivity of the method, the limit of detection (LOD) and limit of quantification were calculated as follows:
Three saline solutions were determined on the basis of five experiments. Linearity and range of detection were determined from serially diluted Sigma PV standard curve plot.
Statistical analysis
The student t-test (one-tailed t-test) was used to analyze the significant difference in antibody titer between weeks of the immunization period (p < .05).
Results
Production of anti-PV IgY
The anti-PV IgY titer of hyperimmunized egg yolks against PV was determined by Indirect ELISA method during the immunization period (). A primary antibody response was determined by the slight increase of IgY titer after the first PV immunization. After the first booster at 2nd week, a rapid secondary response characterized with a high PV-specific IgY activity was peaked at 5th week (p < .05). Thereafter the titer decreased, but reached a peak, a week after the second booster at 8th week (p < .05). As a result, a high antigen-binding specificity of anti-PV IgY could be obtained from the egg yolks collected from immunized eggs during weeks 4–10 of the immunization period.
Figure 3. The anti-PV IgY antibody levels in the WSF of egg yolk (at 1:1000 dilution) measured by Indirect ELISA and expressed as absorbance at 405 nm. Values are the means of quadruple samples. Vertical bars indicate the standard deviation. X axis indicates the weeks of immunization. Arrows indicate immunization time points.
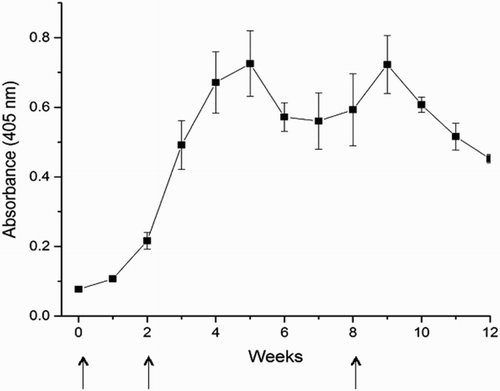
The crude WSF of egg yolk was isolated, further purified using ammonium sulfate precipitation, followed by Sephacryl S-300 gel chromatography. The content of total IgY in the egg yolk was found to be constant (9.0 ± 1.1 mg/mL) among the chickens immunized with PV during the experimental period, as reported previously (Sunwoo, Gujral, & Suresh, Citation2011). The total content of IgY in the hyperimmunized egg yolks was approximately 1.1 times greater than that of the yolks from non-immunized chickens (p > .05). As expected, the PV-specific IgY was not detected in the non-immunized control group, while the proportion of PV-specific IgY in the total egg yolk IgY was 6.2% in the immunized egg yolks ().
Table 1. Concentration of total IgY and specific IgY purified from the hyperimmunized and non-immunized egg yolks.
The gel electrophoretogram of PV is shown in . Sigma PV showed high purity with a dominant 37 kDa band ((A), Lane 2). Western blot analysis confirmed the specificity of IgY antibodies to Sigma PV ((B), Lane 2). Non-specific IgY was used as negative control in place of a primary anti-PV IgY antibody to evaluate non-specific staining ((C)).
Double antibody sandwich ELISA
Sigma PV was used to prepare the standard curve of DAS-ELISA using PV-specific mAb as capture and IgY as detection antibodies ((A)). The lower LOD of PV was determined to be 5.6 μg/mL. The linear range of the assay, as determined using a five-point calibration curve (R2= 0.99; Y= 0.0971 + 0.0067X), was 16.8–90 µg/mL. At lower or higher concentrations, reproducibility and repeatability of PV content were not validated.
Biotinylated DAS-ELISA
Sigma PV was used to prepare a standard curve of biotinylated DAS-ELISA using PV-specific mAb as capture and biotinylated IgY as detection antibodies ((B)). The LOD of PV was determined to be 2.5 ng/mL. The linear range of the assay, as determined using a five-point calibration curve (R2 = 0.99; Y= 0.1302 + 0.0189X), was 7.5–40 ng/mL. At lower or higher concentrations, reproducibility and repeatability of PV content were not validated.
Accuracy
Blank saline solutions, spiked with four different amounts of Sigma PV, were used to determine the recovery rates as shown in . The recovery of the Sigma PV in the saline solutions determined by DAS-ELISA and biotinylated DAS-ELISA was between 80–92% and 88–97%, respectively, independent of spiked PV level. The biotinylated DAS-ELISA being a more accurate assay was selected for the further validation.
Table 2. Recovery (%) of PV from saline solution sample spiked with 1–100 µg/mL Sigma PV.a
Intra- and inter-assay precision
Five saline solutions were spiked with the different concentrations of the Sigma PV and analyzed by the developed biotinylated DAS-ELISA to determine the intra-assay and inter-assay precision (). The PV content in the saline solutions ranged from 0.05 to 68.9 µg/mL. The intra-assay precision expressed as the percentage of CV (% CV) was 7.6% average of five spiked saline solutions. The inter-assay precision was 9.8% in the saline solutions.
Table 3. Intra- and inter-assay variances (% CV) of biotinylated DAS-ELISA for PV quantification in five different saline solutions.
Discussion
Egg yolk is a mixture of lipids, lipoproteins, PV and other water-soluble proteins (WSP). The WSP fraction, including α-, β- and γ-livetins, was separated from the lipids and granular proteins using the acidified water (pH 5.0–5.2) dilution method (De Meulenaer & Huyghbaert, Citation2001; Yoo, Bamdad, Gujral, Suh, & Sunwoo, Citation2017). The remaining granules are the yolk micelles containing lipovitellin and PV (Ren, Li, Offengenden, & Wu, Citation2015). PV is one of the most highly phosphorylated proteins in nature, which contains more than 80% of the phosphorous present in the egg yolk (Joubert & Cook, Citation1958). The high density of polar residues results in a substantial number of charged side chains in physiological pH which lead to different functionalities such as metal chelating, antioxidant and antimicrobial activities (Sunwoo & Gujral, Citation2014).
There is limited information available on the quantification of PV. The highly phosphorylated nature of PV makes it difficult to be determined accurately by normal colorimetric assays such as Bradford, bicinchoninic acid and Lowry protein assays due to the interference from their phosphate groups (Hatta et al., Citation1997). Therefore, this study introduces, for the first time, a simple and reliable method to obtain protein-specific IgY antibodies for direct quantification of PV in combination with mAb antibodies.
The chicken was used to generate antibody employed in the development of PV detection system. The avian antibodies are transferred from mother hens’ sera to egg yolks to confer passive immunity to their offspring in the form of IgY antibody as high as that contained in 30 mL blood per egg (Carlander, Wilhelmson, & Larsson, Citation2001). This process of accumulating antibodies in the egg yolk by transfer of IgG from serum to egg yolk has been exploited, leading to increased use of chicken egg yolk for the production of antibodies to various antigens (Gujral, Suresh, & Sunwoo, Citation2012; Lee, Sunwoo, Menninen, & Sim, Citation2002; Palaniyappan, Das, Kammila, Suresh, & Sunwoo, Citation2012). Compared to conventional mammalian serum antibodies, the ability to generate a high amount of chicken IgY within a short period is a major advantage in producing significant antigen-specific IgY (Schade et al., Citation1994). Therefore, egg yolk IgY can be produced in large quantities and high titers, which may replace other sources of polyclonal and mAbs conventionally used in ELISA or other immunological assays, without compromising the accuracy of the assay. The use of chickens in antibody production is more ethical and would negate the need for blood collection from animals in the laboratory and considerably reduce research cost (Schade et al., Citation1994).
For the first time, chickens were used to produce antibody against chicken PV, although PV is a chicken self-protein. Chickens proved to be highly efficient producers of anti-PV IgY antibodies (). The antibody was produced by the immunization of dephosphorylated PV to chickens. The conformational change of dephosphorylated PV exposed hidden antigenic sites therefore triggering the chicken immune response. In literature, the percentage of specific antibody in total IgY reported ranged from 5% (anti-insulin antibody) to 28% (anti-mouse IgG antibody) (Hatta et al., Citation1997). In this study, 6.2% of the PV-specific IgY per total IgY was obtained (). The Western blot results revealed that anti-PV IgY has distinctive paratopes specific for PV (). Correspondingly, anti-PV IgY did not cross-react with the other egg proteins purchased from Sigma, that is, egg white lysozyme (14 kDa), ovalbumin (44 kDa), egg yolk IgY antibody (180 kDa), determined by an ELISA (data not shown).
Two ELISA systems (DAS-ELISA and biotinylated DAS-ELISA) were developed and validated via mAb and egg yolk IgY. The biotinylated ELISA ensured a detection range of 7.5–40 ng/mL () and had higher accuracy of 88–97% (). This biotinylated DAS-ELISA system is also highly sensitive with over 2000 times lower LOD, compared to the DAS-ELISA. Precision studies that were also carried out to validate the developed assay revealed remarkably low intra- and inter-assay variation coefficients ().
Conclusion
The newly developed IgY-based biotinylated DAS-ELISA method provides a highly specific, sensitive, accurate and precise detection system for PV, with a working detection range of 7.5–40 ng/mL. The ability to detect nanogram levels of PV allows this system to be widely used as a valuable research tool, assisting researchers in characterization, purification and optimization of the analytical performance of PV. Since there is no well-established detection or quantification method for PV, this expounded system has tremendous potential to quantify PV, a promising protein for use in food, nutraceutical and pharmaceutical industries.
Disclosure statement
No potential conflict of interest was reported by the authors.
Notes on contributors
Dr Naiyana Gujral, PhD, Postdoctoral Fellow, Faculty of Pharmacy and Pharmaceutical Sciences. Publications on research and development of antibody- and protein-based therapeutics and diagnostics.
Heejoo Yoo MSc. graduate, Faculty of Pharmacy and Pharmaceutical Sciences.
Dr Fatemeh Bamdad, PhD, Research Associate, Faculty of Pharmacy and Pharmaceutical Sciences. Publications on various aspects of protein and peptide purification, identification, and biological activities.
Dr Joo-Won Suh, Professor, Center for Nutraceutical and Pharmaceutical Materials, Science Campus. An expert in microbiology, biotechnology, applied microbiology, biochemistry and cell biology.
Dr Hoon Sunwoo, Associate Professor, Faculty of Pharmacy and Pharmaceutical Sciences. An expert in pharmaceutical biotechnology and immunology. Publications include The Amazing Egg and various articles on biological activities of peptides and antibodies.
ORCID
Hoon Sunwoo http://orcid.org/0000-0002-1547-5936
Additional information
Funding
References
- Carlander, D., Wilhelmson, M., & Larsson, A. (2001). Limited day to day variation of IgY levels in eggs from individual laying hens. Food and Agricultural Immunology, 13, 87–92. doi:10.1080/09540100120055657
- Chung, S. L., & Ferrier, L. K. (1992). pH and sodium-chloride effects on emulsifying properties of egg-yolk phosvitin. Journal of Food Science, 57, 40–42. doi:10.1111/j.1365-2621.1992.tb05419.x
- De Meulenaer, B., & Huyghbaert, A. (2001). Isolation and purification of chicken egg yolk immunoglobulins: A review. Food and Agricultural Immunology, 13, 275–288. doi:10.1080/09540100120094537
- Grizzuti, K., & Perlmann, G. E. (1973). Binding of magnesium and calcium-ions to phosphoglycoprotein phosvitin. Biochemistry, 12, 4399–4403. doi: 10.1021/bi00746a016
- Gujral, N., Suresh, M. R., & Sunwoo, H. H. (2012). Quantitative double antibody sandwich ELISA for the determination of gliadin. Journal of Immunoassay and Immunochemistry, 33, 339–351. doi:10.1080/15321819.2012.655823
- Gujral, N., Yoo, H. J., Bamdad, F., Suh, J. W., & Sunwoo, H. H. (in press). Growth inhibitory effects of egg-yolk IgY and phosvitin on enterotoxigenic Escherichia coli K88 and K99 in vitro. Current Pharmaceutical Biotechnology.
- Hatta, H., Tsuda, K., Ozeki, M., Kim, M., Yamamoto, T., Otake, S., … Michalek, S. M. (1997). Passive immunization against dental plaque formation in humans: Effect of a mouth rinse containing egg yolk antibodies (IgY) specific to Streptococcus mutans. Caries Research, 31, 268–274. doi:10.1159/000262410
- Hau, J., & Hendriksen, C. F. (2005). Refinement of polyclonal antibody production by combining oral immunization of chickens with harvest of antibodies from the egg yolk. ILAR Journal, 46, 294–299. doi:10.1093/ilar.46.3.294
- Ishikawa, S. I., Tamaki, S., Arihara, K., & Itoh, M. (2007). Egg yolk protein and egg yolk phosvitin inhibit calcium, magnesium, and iron absorptions in rats. Journal of Food Science, 72, S412–S419. doi:10.1111/j.1750-3841.2007.00417.x
- Jensenius, J. C., Andersen, I., Hau, J., Crone, M., & Koch, C. (1981). Eggs-conveniently packaged antibodies – Methods for purification of yolk IgG. Journal of Immunological Methods, 46, 63–68. doi:10.1016/0022-1759(81)90333-1
- Joubert, F. J., & Cook, W. H. (1958). Preparation and characterization of phosvitin from hen egg yolk. Canadian Journal of Biochemical Physiology, 36, 399–408. doi:10.1139/o58-045
- Khan, M. S., Babiker, E. E., Azakami, H., & Kato, A. (1998). Effect of protease digestion and dephosphorylation on high emulsifying properties of hen egg yolk phosvitin. Journal of Agriculture and Food Chemistry, 46, 4977–4981. doi: 10.1021/jf980319r
- Lee, E. N., Sunwoo, H. H., Menninen, K., & Sim, J. S. (2002). In vitro studies of chicken egg yolk antibody (IgY) against Salmonella enteritidis and Salmonella typhimurium. Poultry Science, 81, 632–641. doi:10.1093/ps/81.5.632
- Lu, C. L., & Baker, R. C. (1986). Characteristics of egg-yolk phosvitin as an antioxidant for inhibiting metal-catalyzed phospholipid oxidations. Poultry Science, 65, 2065–2070. doi:10.3382/ps.0652065
- Miller, M. S., Benore-Parsons, M., & White, H. B. (1982). Dephosphorylation of chicken riboflavin-binding protein and phosvitin decreases their uptake by oocytes. Journal of Biological Chemistry, 257, 6818–6824.
- Palaniyappan, A., Das, D., Kammila, S., Suresh, M. R., & Sunwoo, H. H. (2012). Diagnostics of severe acute respiratory syndrome-associated coronavirus (SARS-CoV) nucleocapsid antigen using chicken immunoglobulin Y. Poultry Science, 91, 636–642. doi:10.3382/ps.2011-01916
- Ren, J. D., Li, Q. Y., Offengenden, M., & Wu, J. P. (2015). Preparation and characterization of phosphopeptides from egg yolk phosvitin. Journal of Functional Foods, 18, 190–197. doi:10.1016/j.jff.2015.07.007
- Richards, M. P. (1997). Trace mineral metabolism in the avian embryo. Poultry Science, 76, 152–164. doi: 10.1093/ps/76.1.152
- Samaraweera, H., Zhang, W. G., Lee, E. J., & Ahn, D. U. (2011). Egg yolk phosvitin and functional phosphopeptides – review. Journal of Food Science, 76, R143–R150. doi:10.1111/j.1750-3841.2011.02291.x
- Schade, R., Burger, W., Schoneberg, T., Schniering, A., Schwarzkopf, C., Hlinak, A., & Kobilke, H. (1994). Avian egg yolk antibodies. The egg laying capacity of hens following immunization with antigens of different kind and origin and the efficiency of egg yolk antibodies in comparison to mammalian antibodies. ALTEX, 11, 75–84.
- Sunwoo, H. H., & Gujral, N. (2014). Chemical composition of egg and egg products. In P. C-K. Cheung (Ed.), Handbook of food chemistry (pp. 1–27). New York, NY: Springer Berlin Heidelberg. doi:10.1007/978-3-642-41609-5_28-1
- Sunwoo, H. H., Gujral, N., Lutz, S., & Suresh, M. R. (2012). Double antibody sandwich ELISA and rapid immunoswab assay for detection of gliadin in food. Food and Agricultural Immunology, 23, 169–181. doi:10.1080/09540105.2011.608121
- Sunwoo, H., Gujral, N., & Suresh, M. (2011). Competitive and double antibody sandwich ELISA for the quantification of lactoferrins by using monoclonal and chicken egg yolk IgY antibodies. Journal of Immunoassay and Immunochemistry, 32, 79–92. doi:10.1080/15321819.2010.543218
- US department of Health and Human Services Food and Drug Administration (FDA), Center for Drug evaluation and research (CDER), & Center for Veterinary Medicine (CVM). Guidance for Industry, Bioanalytical method validation: Draft guidance, September 2013, Biopharmaceutics. Retrieved from www.fda.gov/downloads/Drugs/GuidanceComplianceRegulatoryInformation/Guidances/UCM368107.pdf
- Xu, X., Katayama, S., & Mine, Y. (2007). Antioxidant activity of tryptic digests of hen egg yolk phosvitin. Journal of the Science of Food and Agriculture, 87, 2604–2608. doi:10.1002/jsfa.3015
- Yamamura, J., Adachi, T., Aoki, N., Nakajima, H., Nakamura, R., & Matsuda, T. (1995). Precursor-product relationship between chicken vitellogenin and the yolk proteins: The 40 kDa yolk plasma glycoprotein is derived from the C-terminal cysteine-rich domain of vitellogenin II. Biochimica et Biophysica Acta, 1244, 384–394. doi: 10.1016/0304-4165(95)00033-8
- Yoo, H. J., Bamdad, F., Gujral, N., Suh, J. W., & Sunwoo, H. H. (2017). High hydrostatic pressure-assisted enzymatic treatment improves antioxidant and anti-inflammatory properties of phosvitin. Current Pharmaceutical Biotechnology, 18, 158–167. doi:10.2174/1389201017666161216110406