ABSTRACT
Caseinate was glycated by lactose and oligochitosan via the Maillard reaction and transglutaminase catalysis to yield two glycated caseinates (GC-I and GC-II), followed by trypsin hydrolysis to generate GC-I hydrolysate and GC-II hydrolysate, respectively. In comparison with caseinate hydrolysate, only GC-I hydrolysate showed decreased His, Lys, Tyr, and Val contents. All three hydrolysates in dose-dependent manner exerted in vitro immuno-modulatory abilities via activating three immune cells in terms of lymphocytes, macrophages, and natural killer (NK) cells, enhancing the secretion of seven cytokines in lymphocytes and macrophages, but inhibiting the secretion of one cytokine (interleukine-4) in splenocytes. However, GC-I hydrolysate and GC-II hydrolysate showed respective lower and higher immuno-modulatory abilities than caseinate hydrolysate in the most cases. Amino acid losses of GC-I hydrolysate made partially contribution to its impaired immuno-modulatory ability. It is concluded that lactose glycation and oligochitosan glycation of caseinate could lessen and enhance immuno-modulation of tryptic caseinate hydrolysate, respectively.
GRAPHICAL ABSTRACT
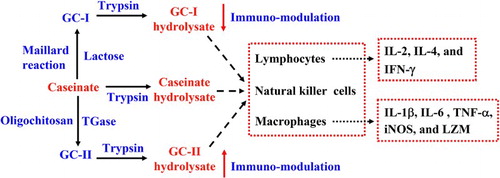
1. Introduction
Caseinate is one of these protein ingredients with good nutritive values and desired functional properties, and thus often used in food processing (Oliver, Melton, & Stanley, Citation2006). However, chemical modifications of proteins can lead to both structure and property changes. The well-known Maillard reaction involving proteins and reducing saccharides has been studied extensively in the recent years to assess its efficiency in property modification of caseinate and other proteins (Ajandouz, Desseaux, Tazi, & Puigserver, Citation2008; Renn & Sathe, Citation1997), as early stage of this reaction can conjugate saccharide moieties into proteins to yield glycated proteins (Arena, Salzano, Renzone, D’Ambrosio, & Scaloni, Citation2014; Martins & van Boekel, Citation2005). With this reaction, glucosamine-glycated casein has enhancements in solubility, emulsifying activity, and foaming capacity (Ramezani, Esmailpour, & Aminlari, Citation2008), while soy protein conjugated by acacia gum, dextran, or xylose has enhanced solubility (Mu, Zhao, Zhao, Cui, & Liu, Citation2011), higher emulsifying, and gelling properties (Cabodevila, Hill, Armstrong, De Sousa, & Mitchell, Citation1994; Diftis & Kiosseoglou, Citation2006). However, protein glycation of the Maillard-type has some defects, such as formation of toxic products and especially nutritional loss (e.g. damage of Lys or other sensitive amino acids) (Brands, Alink, van Boekel, & Jongen, Citation2000). Beside these defects, potential impact of protein glycation of Maillard-type on biofunction of proteins is still not fully assessed. In the recent, transglutaminase (TGase, EC 2.3.2.13) together with amino-containing saccharides is an alternative way to generate glycated proteins (Jiang & Zhao, Citation2010). Under the catalysis of TGase, glucosamine and oligochitosan can glycate caseinate, soy protein, and protein hydrolysates (Fu & Zhao, Citation2016; Hong, Gottardi, Ndagijimana, & Betti, Citation2014; Jiang & Zhao, Citation2010; Song & Zhao, Citation2013). This enzymatic protein glycation approach can overcome these defects (nutritional loss and formation of toxic by-products) found in the Maillard reaction approach. TGase is thus employed in several studies to conjugate both glucosamine and oligochitosan into proteins and peptides (Hong, Ndagijimana, & Betti, Citation2016; Jiang & Zhao, Citation2011; Song & Zhao, Citation2013), to modify their rheological, gelling, emulsifying, and colloidal properties (Fu & Zhao, Citation2016, Citation2017; Song & Zhao, Citation2013). From the chemical point of view, glycated proteins generated from the Maillard reaction and TGase approaches will be different in their structures. Protein glycation via the Maillard reaction is mainly achieved by connecting the Lys residues of proteins with the carbonyl groups of reducing saccharides. However, in the case of TGase approach, the Gln residues of proteins are connected with the primary amines of the amino-containing saccharides, accompanied by unavoidable protein cross-linking. The two approaches thus confer glycated proteins with different glycation sites (i.e. structural features) and especially modified functional properties. Future consideration should focus on biological properties of the glycated proteins, such as these important bioactivities. In the body, proteins will be degraded by proteases, resulting in the generation of protein hydrolysates or digests. Peptides mostly have anti-oxidative (Kleekayai et al., Citation2015), anti-hypertensive (Fu et al., Citation2016), immunological (Gauthier, Pouliot, & Saint-Sauveur, Citation2006; Mercier, Gauthier, & Fliss, Citation2004), and other activities. It is still unknown that if prior protein glycation via the two approaches might decrease or increase bioactivities of protein hydrolysates.
The immune system is a sophisticated and intricate network comprising immune organs, tissues, cells, molecules, and proteins that function collectively to protect the body against foreign invasions such as toxins, parasites, and germs (Ahmad, Jantan, Kumolosasi, & Bukhari, Citation2015; Calder et al., Citation2006). Main immune factors of antigen-specific immune responses include T-lymphocytes and their cytokines such as interleukine-2 (IL-2), IL-4, interferon-γ (IFN-γ), and others (Parkin & Cohen, Citation2001). Macrophages are regarded as important immune effector cells due to their regulations of innate and adaptive immunity and their roles in phagocytosis (Kim et al., Citation2013). Macrophages can stimulate lymphocytes and other immune cells (Luft, Citation2008), and defend against pathogen invasion by producing these pro-inflammatory cytokines such as tumor necrosis factor-α (TNF-α) and IL-1 (Balkwill, Citation1992), or by releasing those cytotoxic and inflammatory molecules such as nitric oxide (NO) and reactive oxygen species (ROS) (Carini et al., Citation2004; Goossens, Grooten, De Vos, & Fiers, Citation1995). In addition, NK cells play a particularly important role in the suppression of tumor growth and metastasis (Lee, Lee, & Lim, Citation2014; Vivier, Tomasello, Baratin, Walzer, & Ugolini, Citation2008). These immune cells and cytokines can be used to assess in vitro immuno-modulatory activities of food components such as whey protein, ovomucoid glycopeptides of chicken egg white, tryptic protein hydrolysates, and soluble plant polysaccharides (Hao & Zhao, Citation2016; Hou, Fan, Li, Xue, & Yu, Citation2012; Matsuda, Gu, Tsuruta, & Nakamura, Citation1985; Mercier et al., Citation2004). A glycated lysozyme (LZM) via the Maillard reaction has also been assessed for its immune activities using these immune cells and cytokines (Ha et al., Citation2013). In general, immune functions of the body can be improved by the so-called immuno-modulatory peptides via modulating lymphocyte proliferation, NK cell activity, antibody synthesis, and cytokine secretion (FitzGerald & Murray, Citation2006). This fact implies that the effect of prior protein glycation on immuno-modulatory abilities of the resultant protein hydrolysates is an important issue, and should be given a detailed investigation.
In this study, two glycated caseinates (GC-I and GC-II) were prepared using caseinate, lactose, and oligochitosan via the Maillard reaction and TGase approaches, respectively; after that, they were hydrolyzed by trypsin to generate two tryptic hydrolysates. The two hydrolysates were assessed for their immuno-modulatory activities to three immune cells (splenocytes, macrophages, and NK cells) and their effects on the yields of seven cytokines including IL-2, IL-4, and IFN-γ (secreted by splenocytes) as well as IL-6, IL-1β, TNF-α, inducible nitric oxide synthase (iNOS), and LZM (secreted by macrophage model). Tryptic caseinate hydrolysate was used as control to compare immuno-modulatory activity changes. Amino acid profiles of these hydrolysates were analyzed and used to confirm their activity differences. The aim of this study was to assess if caseinate glycation of the two types might impact immuno-modulatory ability of tryptic caseinate hydrolysate, and to reveal possible benefit or disadvantage of protein glycation of the two types on immuno-modulatory abilities of proteins.
2. Materials and methods
2.1. Materials and chemicals
Concanavalin A (Con A), sodium caseinate, and lactose were bought from Sigma-Aldrich Co. (St. Louis, MO, USA). Oligochitosan with a declared deacetylation degree of 75% and an average molecular weight of 1 kDa was obtained from Zhejiang Golden-Shell Biochemical Co. (Hangzhou, Zhejiang, China). Phosphate-buffered saline (PBS) was bought from Solarbio Science and Technology Co. Ltd. (Beijing, China). TGase with a measured activity of 92 units (U) per gram was obtained from Jiangsu Yiming Fine Chemical Industry Co. Ltd. (Jiangsu, China), whilst trypsin (EC 3.4.21.4) with a measured activity of 120 kU per gram was bought from Beijing Aoboxing Biotechnologies Inc. (Beijing, China). Typan blue and neutral red both were bought from Amresco Inc. (Los Angeles, CA, USA). The RPMI-1640 was purchased from HyClone Co. (Logan, UT, USA). Fetal bovine serum (FBS) was obtained from Wisent Inc. (Montreal, Quebec, Canada). Other chemicals used were of analytical grade. Water used in the chemical experiments was redistilled water whilst that used in cell experiments was ultrapure water.
The EnzycChromTM Galactose Assay Kit was purchased from BioAssay Systems (Hayward, CA, USA). The Cell Counting Kit-8 (CCK-8) was purchased from Dojindo Laboratories (Kyushu, Japan). The Mouse iNOS and LZM enzyme-linked immunosorbent assay (ELISA) kits were purchased from Beijing Equation Biotechnology Science and Technology Co. Ltd. (Beijing, China). All cytokines kits were brought from Wuhan Boster Biological Engineering Co. Ltd. (Wuhan, Hubei, China).
2.2. Animals and cells
Female BALB/c mice were purchased from Beijing Vital River Experimental Animal Technical Co. Ltd. (Beijing, China). All mice were 6–8-week-old, and maintained in animal facility for at least one week before experiment. All animal procedures were performed in compliance with the guidelines of the Animal Care and Use Committee of Northeast Agricultural University (Harbin, Heilongjiang Province, China).
The YAC-1 cells and RAW 264.7 murine macrophages were purchased from the Cell Bank of the Chinese Academy of Sciences (Shanghai, China).
2.3. Preparation of two glycated caseinates and tryptic hydrolysates
Lactose-glycated caseinate was prepared via the Maillard reaction using the conditions as previously described (Wang & Zhao, Citation2017). In brief, the reaction system composed of caseinate (50 g/L) and lactose (80 g/L) at a pH value of 6.7, and was heated at a water bath (100°C) for 3 h with constant agitation. The mixture was rapidly cooled in an ice bath to stop the reaction, and then subjected to isoelectric precipitation (pH 4.5) followed by isoelectric washing of three times to remove the free lactose. The collect precipitate was lactose-glycated caseinate and named as GC-I.
Oligochitosan-glycated caseinate was prepared as per the study (Song & Zhao, Citation2014) with minor change. Caseinate was dispersed in water at a pH value of 7.5 to achieve a protein concentration of 80 g/L, and then mixed with oligochitosan (pH 7.5). This mixing resulted in 1:3 molar ratio of acyl donor to oligochitosan acceptor, and caseinate content of 50 g/L. TGase was used at 10 U/g protein to induce protein glycation and cross-linking at 37°C for 3 h. After then, the mixture was heated at 85°C for 5 min to inactivate TGase, cooled rapidly to ambient temperature, and then subjected to isoelectric precipitation and two isoelectric washings to remove the free oligochitosan. The collect precipitate was oligochitosan-glycated caseinate, and named as GC-II.
GC-I (or GC-II) was dispersed in water at pH 7.0, added with trypsin of 7 kU/g protein, and kept at a water bath of 37°C for 4 h to trigger protein hydrolysis. After that, the mixture was heated rapidly for 5 min at a boiling bath, cooled to ambient temperature, adjusted to pH 7.0 via adding 0.5 mol/L NaOH, and centrifuged at 5000×g for 20 min to obtain tryptic GC-I (or GC-II) hydrolysate. The two hydrolysates were freeze-dried and used in later evaluations. Caseinate was also treated similarly to generate tryptic caseinate hydrolysate (as control). The three hydrolysates were used at three dose levels (25, 50, and 100 µg/mL) to evaluate their in vitro immune activities.
2.4. Chemical analyses
Protein contents of all samples were assessed using the Kjeldahl method and a suggested conversion factor of 6.38 (AOAC, Citation2005). For GC-II hydrolysate, nitrogen amount arisen from the connected oligochitosan was calculated from the measured glucosamine content (as below), and then deducted from the assaying result.
GC-I hydrolysate was hydrolyzed by 2 mol/L trifluoroacetic acid at 100°C for 4 h as previously described (Račaitytė, Kiessig, & Kálmán, Citation2005), cooled to ambient temperature, neutralized into pH 7.0 using 2 mol/L NaOH, and then detected for galactose content as per the study (Wang & Zhao, Citation2017) using the galactose assay kit and procedure provided by the kit producer. Lactose content (g/kg protein) was thereby calculated chemically.
Glucosamine content was detected by high-performance liquid chromatography (HPLC) using pre-column derivatization and fluorescence detection. GC-II hydrolysate was hydrolyzed by 6 mol/L HCl at 100°C for 4 h, cooled to ambient temperature, and then neutralized into pH 7.0 using 2 mol/L NaOH. Glucosamine analysis followed a reported method (Anumula, Citation1994). Glucosamine content (g/kg protein) was calculated from standard curve generated from serial glucosamine solutions.
Amino acid contents (g/kg protein) of the samples were assayed by Heilongjiang Provincial Academy of Agricultural Sciences using an automated amino acid analyzer (L-880 Hitachi Co. Ltd., Tokyo, Japan) and following the procedure recommended by the instrument manufacture.
2.5. Assay of lymphocyte proliferation
Mouse splenocytes were prepared as previously described (Hao & Zhao, Citation2016), and then seeded onto three wells of a 96-well plate (100 μL per well). Serial solutions of the three hydrolysates of 80 μL were added into each well to achieve final hydrolysate levels of 25–100 μg/mL. Complete RPMI-1640 medium containing 10% FBS alone was used as blank. Con A of 20 μL was added into each well to achieve final contents of 5 μg/mL. After incubation at 37°C in 5% CO2 for 24–48 h, CCK-8 of 20 μL was added into each well, and the cells were incubated for another 4 h. The absorbance was measured at 450 nm by a microplate reader (Bio Rad Laboratories, Hercules, CA, USA). Lymphocyte proliferation was calculated as previously described (Wang et al., Citation2013).
2.6. Assay of macrophage phagocytosis
Macrophage phagocytosis was assayed as per the study (Wang et al., Citation2013). The RAW 264.7 murine macrophages were diluted to a final density of 2×105 cells/mL in the RPMI 1640-FBS medium (containing 10% FBS), plated onto three wells of a 96-well plate (100 μL per well), cultured at 37°C in 5% CO2 for 12 h. After discarding the suspension, the RPMI 1640-FBS medium (containing 10% FBS) and the three hydrolysates (25–100 µg/mL) of 100 μL were added into each well. Complete RPMI-1640 medium containing 10% FBS alone was used as blank. The cells were incubated for 24–48 h. After discarding the suspension again, neutral red solution (1%, in 0.9% NaCl) of 100 μL was added into each cell. After further incubation of 30 min, the cells were washed three times with 0.1 mol/L PBS (pH 7.2) to remove excess neutral red. A mixture composed of equal volume of acetic acid (1%, w/w) and ethanol (95%, v/v) was added at 100 μL per well. The cells were incubated again for 2 h. The absorbance was measured at 540 nm by the microplate reader. Macrophage phagocytosis was calculated as previously described (Wang et al., Citation2013).
2.7. Assay of NK cell activity
NK cell activity was assayed using a CCK-8 assay kit. Splenocytes were prepared as effector cells, while YAC-1 cells were used as target cells. Splenocytes (5 × 105 cells/mL) and YAC-1 cells (1 × 104 cells/mL) of 50 μL were plated onto three wells of a 96-well plate. The three hydrolysates (25–100 μg/mL) of 50 μL were also added into each well. The cells were incubated at 37°C for 24–48 h, and then CCK-8 of 15 μL was added into each well. After another 4 h of co-culture, the absorbance was measured at 450 nm by the microplate reader. NK cell activity was calculated as previously described (Yuan et al., Citation2010).
2.8. Measurements of IL-2, IL-4, IFN-γ, IL-1β, IL-6, TNF-α, iNOS, and LZM
Splenocytes or macrophages (2 × 105 cells/mL) of 2 mL were put into three wells of a 6-well plate. These hydrolysates (25–100 μg/mL) of 1 mL were added into each well. The cells were cultured at 37°C for 24–48 h, and centrifuged at 2000 × g for 20 min to collect cellular supernatants to assay the respective levels of IL-2, IL-4, IFN-γ, IL-1β, IL-6, TNF-α, iNOS, and LZM, using the respective ELISA kits and protocols provided by the kit manufacturers.
2.9. Statistical analysis
All preparations and analyses were carried out at least in triplicate. All reported data were expressed as means ± standard deviations using the SPSS 16.0 software (SPSS Inc., Chicago, IL, USA). The differences between the mean values of multiple groups were analyzed by one-way analysis of variance (ANOVA) with Duncan’s multiple range tests.
3. Results
3.1. Saccharide and essential amino acid contents of the tryptic hydrolysates
The three hydrolysates prepared in this study were assessed for their lactose and glucosamine contents. Caseinate hydrolysate did not contain any lactose and glucosamine; however, GC-I hydrolysate and GC-II hydrolysate contained lactose and glucosamine of 10.6 and 5.7 g/kg protein (), respectively. The results indicated that caseinate was conjugated with lactose and oligochitosan during the preparation of GC-I and GC-II, resulting in GC-I hydrolysate and GC-II hydrolysate containing detectable lactose and glucosamine, respectively. In other words, GC-I hydrolysate and GC-II hydrolysate contained some glycated peptides.
Table 1. Saccharide and 10 amino acid contents (g/kg protein) of the tryptic hydrolysates from caseinate, GC-I, and GC-II.
The three hydrolysates were also detected for their amino acid contents. The results for 10 amino acids are listed in . In comparison with caseinate hydrolysate, GC-I hydrolysate showed lower contents in His, Lys, Tyr, and Val, but GC-II hydrolysate was less different from caseinate hydrolysate in these amino acids. GC-I hydrolysate and GC-II hydrolysate thus might have different immuno-modulatory activities, verifying potential impacts of the two protein glycation approaches on immuno-modulation of tryptic caseinate hydrolysates.
3.2. Activation of the tryptic hydrolysates on three immune cells
The three hydrolysates were used at three levels to assess their activation on three immune cells with two incubation times (24 and 48 h) (). All hydrolysates could activate the three immune cells, and higher hydrolysate levels brought greater activation (i.e. higher values of these indices). In comparison with caseinate hydrolysate, GC-I hydrolysate totally showed 4.8–19.6% weaker activation, whereas GC-II hydrolysate exerted 1.6–17.3% stronger activation. The detailed results are summarized as below.
Table 2. Effects of the tryptic hydrolysates of caseinate, GC-I, and GC-II at three doses on three immune cells.
When lymphocytes were incubated with the three hydrolysates for 24 h, caseinate hydrolysate resulted in lymphocyte proliferation of 1.257–1.402 while GC-I hydrolysate and GC-II hydrolysate led to lymphocyte proliferation of 1.193–1.309 and 1.293–1.456, respectively. GC-I hydrolysate and GC-II hydrolysate showed respective weaker and stronger activation than caseinate hydrolysate. If another incubation time of 48 h was used, caseinate hydrolysate, GC-I hydrolysate, and GC-II hydrolysate rendered higher lymphocyte proliferation because the measured index values were enhanced to 1.293–1.496, 1.224–1.334, and 1.337–1.520, respectively. GC-II hydrolysate (or GC-I hydrolysate) was 1.6–3.9% more active (or 5.1–10.8% weaker) than caseinate hydrolysate to activate lymphocytes. That is, lactose glycation and oligochitosan glycation brought respective GC-I hydrolysate and GC-II hydrolysate with lower and higher lymphocyte activation.
When macrophages were incubated for 24 h, caseinate hydrolysate, GC-I hydrolysate, and GC-II hydrolysate led to respective macrophage phagocytosis of 1.133–1.380, 1.079–1.310, and 1.189–1.477. GC-I hydrolysate and GC-II hydrolysate had respective weaker and stronger abilities than caseinate hydrolysate to increase macrophage phagocytosis. If an incubation time of 48 h was used, respective phagocytosis values were increased into 1.224–1.480, 1.145–1.401, and 1.280–1.565. Regarding caseinate hydrolysate, GC-II hydrolysate showed 4.6–7.0% higher activation but GC-I hydrolysate displayed 4.8–6.5% weaker activation. The results also proved that lactose glycation and oligochitosan glycation resulted in respective GC-I hydrolysate and GC-II hydrolysate with lower and higher abilities to activate macrophages.
The three hydrolysates had different NK cell activities. With an incubation time of 24 h, caseinate hydrolysate, GC-I hydrolysate, and GC-II hydrolysate showed respective NK cell activities of 31.5–45.1%, 25.7–41.4%, and 35.8–52.9%. GC-I hydrolysate and GC-II hydrolysate had respective weaker and stronger NK cell activities than caseinate hydrolysate. With another incubation time of 48 h, the three hydrolysates showed increased activities of 37.3–53.8%, 30.0–48.4%, and 41.7–58.4%, respectively. In general, GC-II hydrolysate had 8.6–17.3% activity increases but GC-I hydrolysate showed 8.2–19.6% activity decreases compared with caseinate hydrolysate. That is, lactose glycation, and oligochitosan glycation conferred GC-I hydrolysate and GC-II hydrolysate with decreased and enhanced NK cell activities, respectively.
3.3. Effects of the tryptic hydrolysates on IL-2, IL-4, and IFN-γ secretion in splenocytes
IL-2, IL-4, and IFN-γ yields in splenocytes were used to depict splenocyte activation of the three hydrolysates. All hydrolysates could increase IL-2 and IFN-γ yields but decrease IL-4 production in dose-dependent manner (). However, the three hydrolysates also showed different abilities to activate splenocytes. In comparison with caseinate hydrolysate, GC-I hydrolysate and GC-II hydrolysate displayed respective 6.8–19.9% lower and 3.7–28.7% higher activities. The detailed results are listed below.
Table 3. Effects of the tryptic hydrolysates of caseinate, GC-I, and GC-II on the secretion of three cytokines in splenocytes.
When splenocytes were incubated for 24 h, caseinate hydrolysate resulted in IL-2 yields of 42.3–52.2 pg/mL, whilst GC-I hydrolysate and GC-II hydrolysate led to IL-2 yields of 38.9–47.3 and 46.5–56.6 pg/mL, respectively. With another incubation time of 48 h, the three hydrolysates enhanced IL-2 yields into 48.6–57.2, 45.3–52.0, and 50.4–60.2 pg/mL, respectively. In comparison with caseinate hydrolysate, GC-II hydrolysate had 3.7–9.9% higher activity but GC-I hydrolysate showed 6.8–9.4% lower activity. Similar results could be found using IFN-γ as evaluation index. With incubation time of 24 h, the three hydrolysates rendered IFN-γ yields of 30.0–46.8, 26.9–42.4, and 38.6–50.9 pg/mL, respectively. With another incubation time of 48 h, the three hydrolysates increased IFN-γ yields into 37.4–51.6, 32.7–47.5, and 43.9–58.4 pg/mL, respectively. In general, GC-II hydrolysate was more active (8.8−28.7% higher), whereas GC-I hydrolysate was weaker (7.9–12.6% lower) than caseinate hydrolysate to increase IFN-γ production. Lactose glycation and oligochitosan glycation were thus verified to confer GC-I hydrolysate and GC-II hydrolysate with lower and higher abilities to enhance IL-2 and IFN-γ secretion in splenocytes, respectively.
When being incubated for 24 h, IL-4 yields in response to caseinate hydrolysate, GC-I hydrolysate, and GC-II hydrolysate were 20.1–25.6, 24.1–28.2, and 18.1–23.8 pg/mL, respectively. Longer incubation time of 48 h led to much decreased IL-4 yields of 18.5–23.3, 20.1–26.4, and 16.7–20.9 pg/mL, respectively. Regarding caseinate hydrolysate, GC-I hydrolysate and GC-II hydrolysate showed 8.6–19.9% lower and 7.0–10.3% higher activities to decrease IL-4 secretion. Lactose and oligochitosan glycation were thus suggested conferring GC-I hydrolysate and GC-II hydrolysate with respective lower and higher abilities to inhibit IL-4 secretion in splenocytes.
3.4. Activation of the tryptic hydrolysates on RAW 264.7 macrophages
Macrophage activation of the three hydrolysates was reflected by the secretion of IL-1β, IL-6, TNF-α, iNOS, and LZM in the treated macrophages. The results () showed that all hydrolysates were able to increase IL-1β, IL-6, TNF-α, iNOS, and LZM secretion in the macrophages, and higher hydrolysate levels resulted in higher yields of the five immune molecules (i.e. greater macrophage activation). Detailed yields of the five immune molecules responsible to the three hydrolysates are briefly listed as follows:
When macrophages were incubated for 24 h, caseinate hydrolysate, GC-I hydrolysate, and GC-II hydrolysate led to respective IL-1β yields of 77.1–108.4, 73.8–102.4, and 84.8–116.2 pg/mL. Incubation time of 48 h brought greater macrophage activation, as respective IL-1β yields were increased to 87.6–116.5, 82.9–108.9, and 92.6–123.7 pg/mL. Regarding caseinate hydrolysate, GC-II hydrolysate showed 5.7–10.0% higher activity but GC-I hydrolysate exhibited 4.2–6.5% lower activity.
When macrophages were incubated for 24 h, the three hydrolysates brought IL-6 yields of 25.2–38.2, 23.6–34.8, and 27.6–44.2 pg/mL, respectively. With another incubation time of 48 h, IL-6 yields were enhanced into 29.9–45.6, 27.7–42.5, and 31.6–49.6 pg/mL, respectively. In comparison with caseinate hydrolysate, GC-II hydrolysate showed 5.7–15.7% higher activity but GC-I hydrolysate exhibited 6.3–8.9% lower activity.
With an incubation time of 24 h, the three hydrolysates led to respective TNF-α yields of 39.2–102.9, 30.1–89.4, and 46.8–121.2 pg/mL. With another incubation time of 48 h, the three hydrolysates increased respective TNF-α yields to 55.6–128.0, 47.1–105.8, and 69.6–142.8 pg/mL. Compared to caseinate hydrolysate, GC-II hydrolysate was 11.6–25.2% more active but GC-I hydrolysate was 13.1–23.2% weaker.
Using an incubation time of 24 h, the three hydrolysates resulted in respective iNOS yields of 138.5–329.9, 106.9–261.3, and 179.3–363.1 pg/mL. Using another incubation time of 48 h, iNOS yields were risen to 167.2–426.9, 124.9–374.2, and 209.5–449.2 pg/mL, respectively. In comparison with caseinate hydrolysate, GC-II hydrolysate had 5.2–29.5% activity increases but GC-I hydrolysate showed 12.3–25.3% activity decreases.
Using an incubation time of 24 h, the three hydrolysates led to respective LZM yields of 15.6–28.6, 12.1–23.2, and 22.6–32.8 ng/mL. Using another incubation time of 48 h, LZM yields were increased to 22.1–31.1, 18.5–25.9, and 26.9–36.2 ng/mL, respectively. Regarding the measured activity of caseinate hydrolysate, GC-II hydrolysate showed 14.7–44.9% activity increases but GC-I hydrolysate displayed 16.3–22.4% activity decreases.
Table 4. Effects of the tryptic hydrolysates of caseinate, GC-I, and GC-II on the secretion of five cytokines in RAW 264.7 murine macrophages.
All these results () showed a consistent conclusion; that is, lactose glycation and oligochitosan glycation of caseinate conferred the respective tryptic caseinate hydrolysates with weaker and stronger macrophage activation. GC-I hydrolysate totally showed 4.2–25.3% weaker activation but GC-II hydrolysate displayed 5.7–44.9% stronger activation on macrophages, in comparison with caseinate hydrolysate.
4. Discussion
Immuno-mediatory function of proteins and peptides is an interesting topic in the field of food science. Some proteins and especially their hydrolysates have been assessed for their immune properties, as protein hydrolysis can manifest various bioactivities, including immune activities (Gauthier et al., Citation2006). In general, immuno-modulatory peptides may exert their activities on the immune system of the body in three different ways: regulation of cytokine expression, production of antibody, and ROS-induced immune functions (Hartmann & Meisel, Citation2007). The peptides isolated from chicken bursa can promote the development of B cells and the production of specific antibody and cytokines (Liu et al., Citation2013). Shark protein hydrolysate shows immune effect via up-regulating the IgA-producing cells and the secretion of IL-6 and TNF-α (Mallet et al., Citation2014). Pine nut meal protein peptides with molecular weights of 3–10 kDa have immuno-competence in the mice by enhancing macrophage phagocytosis and phagocytosis index, and by increasing the production of four cytokines significantly (Lin, Liu, Liu, & Yu, Citation2017). Regarding milk proteins, both casein and whey proteins have been assessed for their immuno-modulatory abilities, as proteolysis of milk proteins can lead to the formation of immuno-modulatory peptides (Gill, Doull, Rutherfurd, & Cross, Citation2000). Whey protein and its enzymatic hydrolysate can stimulate splenocyte proliferation (Mercier et al., Citation2004). Two casein-derived peptides Gln-Glu-Pro-Val-Leu and Gln-Glu-Pro-Val are capable of enhancing lymphocyte proliferation and inhibiting LPS-induced inflammation through regulating NO and cytokine production (Zhou et al., Citation2014). Tryptic hydrolysate of bovine α-s1 casein can enhance antibody formation and macrophage phagocytosis (Jollès, Fiat, Migliore-Samour, Drouet, & Caen, Citation1992; Schlimme & Meisel, Citation1995). In general, milk proteins and their hydrolysates are able to modulate a variety of immune activities, including lymphocyte activation, cytokine secretion, antibody production, phagocytic activity, and granulocyte and NK cell activity (Gauthier et al., Citation2006).
Protein hydrolysates (or peptides) usually contain 3–20 amino acid residues, and their bioactivities depend on both amino acid compositions and sequences (Kim, Je, & Kim, Citation2007). Hydrophobic and hydrophilic amino acids such as Pro, Lys, and Arg are important to several bioactivities (Santiago-López, Hernández-Mendoza, Vallejo-Cordoba, Mata-Haro, & González-Córdova, Citation2016). Immune activities of protein hydrolysates are thus governed by both molecular weights and amino acid compositions (Hou et al., Citation2012; Santiago-López et al., Citation2016). This means that any loss of these amino acids in GC-I hydrolysate or GC-II hydrolysates might lead to changed immune activities. The Maillard reaction is known to reduce nutritional properties of proteins (Martins, Jongen, & van Boekel, Citation2000), by reducing bioavailability of several amino acids (especially Lys) (Varela, Arce, Marcos, & Castrillón, Citation1997). The present study also agreed that GC-I preparation led to Lys loss in GC-I hydrolysate (). It is speculated briefly that Lys damage during GC-I preparation resulted in GC-I hydrolysate with weaker immuno-modulatory ability, in comparison with caseinate hydrolysate. In a recent study, a galactomannan-glycated LZM has been assessed for its immune activities; however, raw LZM is not assessed (Ha et al., Citation2013). Potential adverse (or beneficial) effect of galactomannan glycation on immune activities of LZM therefore cannot be drew form those measured index values. In the present study, both caseinate hydrolysate and GC-I hydrolysate were assessed and compared for their immune activities, which enabled us to verify potential impact of prior lactose glycation on immune activities of tryptic caseinate hydrolysate. However, in conflict with the results of the present study, a glycated whey protein via Maillard reaction is found to have immune activities higher than raw whey protein (Chun et al., Citation2016).
Glycoproteins and glycolipids can influence antigenic specificity and immune induction of the host (Dell, Haslam, Morris, & Khoo, Citation1999; Die & Cummings, Citation2010). Ovomucoid glycopeptides from chicken egg white have immune activities (Matsuda et al., Citation1985). A glycoprotein from rice bran can modulate immune responses in the RAW 264.7 macrophages via influencing the production of NO, TNF-α, IL-1β, IL-6, and IL-10 (Park et al., Citation2013). In the present study, GC-II was prepared using lower temperature (37°C) than GC-I (100°C), but the same reaction time (3 h) was used. In theory, GC-II thus prepared had less amino acid loss than GC-I (), which ensured GC-II hydrolysate with immuno-modulatory activities similar to caseinate hydrolysate. In general, trypsin is special to hydrolyze these peptide bonds formed by Lys and Arg residues (Shu et al., Citation2015). That is, the oligochitosan connected in Gln residues of GC-II was not released by trypsin during GC-II hydrolysis. GC-II hydrolysate therefore contained some peptides glycated by oligochitosan, reflected by its HPLC analysis result (). These glycated peptides in GC-II hydrolysate had stronger immune activities than the counterpart peptides in caseinate hydrolysate. GC-II hydrolysate in all cases was detected with higher immune activities than caseinate hydrolysate (–). GC-I hydrolysate also contained some peptides glycated by lactose. However, the effect of amino acid (especially Lys) loss in GC-I predominated over that of these glycated peptides. GC-I hydrolysate in all cases was thus detected with weaker immune activities than caseinate hydrolysate (–).
The results of this study demonstrated that prior lactose glycation of caseinate via the Maillard reaction will weaken immuno-modulatory ability of caseinate hydrolysate, which is not reported in the past studies. This finding might have practical implications, as the Maillard reaction is often used in protein modification and widely occurs in many processed foods such as dairy foods. However, prior oligochitosan glycation of caseinate via the TGase approach in somewhat extent increased immuno-modulatory ability of caseinate hydrolysate. This study might for the first time reveal an adverse effect of the Maillard reaction as well as a beneficial effect of TGase approach on immuno-modulation of the glycated caseinates. During thermal treatment of food materials, avoiding the Maillard reaction is surely not practical; however, controlling the Maillard reaction at less reaction extent is therefore very necessary, to decrease these negative nutritional and immunological effects of the Maillard reaction on food proteins. Based on the present results, possible adverse impacts of the Maillard reaction on proteins should be reconsidered and revised. However, to seek for further supporting evidence, a study using animal model is necessary. The glycated protein yielded from other proteins and reducing saccharides should also be studied in future, to clarify potential effect of the Maillard reaction on the immune activities of the result protein hydrolysates.
5. Conclusions
Using the mentioned two protein glycation approaches and trypsin hydrolysis, it was verified that GC-I hydrolysate and GC-II hydrolysate had respective immuno-modulatory abilities lower and higher than caseinate hydrolysate, although they all were capable of activating three immune cells, enhancing the secretion of seven immune molecules, and inhibiting IL-4 secretion in splenocytes and macrophages. Lactose glycation of caseinate via the Maillard reaction conferred tryptic caseinate hydrolysate decreased immuno-modulatory ability, whereas oligochitosan glycation of caseinate via TGase approach led to an opposite result. Amino acid damage during GC-I preparation might partially make contribution to the decreased immuno-modulatory activities of GC-I hydrolysate, while oligochitosan glycation in somewhat extent conferred GC-II hydrolysate with enhanced immuno-modulatory activities. It is also highlighted that controlling the Maillard reaction extents of proteins during thermal processing of foods is necessary, if aiming to remain immuno-modulatory activities of proteins or protein hydrolysates at the maximum.
Acknowledgements
The authors thank the anonymous reviewers and editors for their valuable advice.
Disclosure statement
No potential conflict of interest was reported by the authors.
Notes on contributors
Jia Shi is a PhD candidate in Food Chemistry and Nutrition. Her research interests are protein modification and food immunology. Presently, she is studying in Northeast Agricultural University, Harbin, China.
Xin-Huai Zhao is a professor in Food Science. His research interests are food proteins, phytochemicals, applied enzymology, and molecular nutrition. He is working in Northeast Agricultural University, Harbin, China.
ORCID
Xin-Huai Zhao http://orcid.org/0000-0001-9682-5426
Additional information
Funding
References
- Ahmad, W., Jantan, I., Kumolosasi, E., & Bukhari, S. N. A. (2015). Immunostimulatory effects of the standardized extract of Tinospora crispa on innate immune responses in Wistar Kyoto rats. Drug Design Development and Therapy, 9, 2961–2973.
- Ajandouz, E. H., Desseaux, V., Tazi, S., & Puigserver, A. (2008). Effects of temperature and pH on the kinetics of caramelisation, protein cross-linking and Maillard reactions in aqueous model systems. Food Chemistry, 107, 1244–1252. doi: 10.1016/j.foodchem.2007.09.062
- Anumula, K. R. (1994). Quantitative determination of monosaccharides in glycoproteins by high-performance liquid chromatography with highly sensitive fluorescence detection. Analytical Biochemistry, 220, 275–283. doi: 10.1006/abio.1994.1338
- AOAC. (2005). Official methods of analysis (6th ed.). Washington, DC: Author.
- Arena, S., Salzano, A. M., Renzone, G., D’Ambrosio, C., & Scaloni, A. (2014). Non-enzymatic glycation and glycoxidation protein products in foods and diseases: An interconnected, complex scenario fully open to innovative proteomic studies. Mass Spectrometry Reviews, 33, 49–77. doi: 10.1002/mas.21378
- Balkwill, F. R. (1992). Tumour necrosis factor and cancer. Progress in Growth Factor Research, 4, 121–137. doi: 10.1016/0955-2235(92)90027-F
- Brands, C. M., Alink, G. M., van Boekel, M. A., & Jongen, W. M. (2000). Mutagenicity of heated sugar-casein systems: Effect of the Maillard reaction. Journal of Agricultural and Food Chemistry, 48, 2271–2275. doi: 10.1021/jf9907586
- Cabodevila, O., Hill, S. E., Armstrong, H. J., De Sousa, I., & Mitchell, J. R. (1994). Gelation enhancement of soy protein isolate using the Maillard reaction and high temperatures. Journal of Food Science, 59, 872–875. doi: 10.1111/j.1365-2621.1994.tb08147.x
- Calder, P. C., Krauss-Etschmann, S., de Jong, E. C., Dupont, C., Frick, J. S., Frokiaer, H., … Lacker, G. (2006). Early nutrition and immunity – progress and perspectives. British Journal of Nutrition, 96, 774–790. doi: 10.1079/BJN20061881
- Carini, M., Aldini, G., Orioli, G., Piccoli, A., Rossoni, G., & Facino, R. M. (2004). Nitric oxide release and distribution following oral and intraperitoneal administration of nitroaspirin (NCX 4016) in the rat. Life Sciences, 74, 3291–3305. doi: 10.1016/j.lfs.2003.11.018
- Chun, S. H., Lee, H. A., Lee, K. B., Kim, S. H., Park, K. Y., & Lee, K. W. (2016). Effects of glycated whey protein concentrate on pro-inflammatory cytokine expression and phagocytic activity in RAW 264.7 macrophages. Biological & Pharmaceutical Bulletin, 39, 199–206. doi: 10.1248/bpb.b15-00596
- Dell, A., Haslam, S. M., Morris, H. R., & Khoo, K. H. (1999). Immunogenic glycoconjugates implicated in parasitic nematode diseases. Biochimica et Biophysica Acta-Molecular Basis of Disease, 1455, 353–362. doi: 10.1016/S0925-4439(99)00064-2
- Die, I. V., & Cummings, R. D. (2010). Glycomics in unraveling glycan-driven immune responses by parasitic helminthes. In R. D. Cummings, & J. M. Pierce (Eds.), Handbook of Glycomics (pp. 367–397). Burlington: Academic Press.
- Diftis, N., & Kiosseoglou, V. (2006). Physicochemical properties of dry-heated soy protein isolate-dextran mixtures. Food Chemistry, 96, 228–233. doi: 10.1016/j.foodchem.2005.02.036
- FitzGerald, R. J., & Murray, B. A. (2006). Bioactive peptides and lactic fermentations. International Journal of Dairy Technology, 59, 118–125. doi: 10.1111/j.1471-0307.2006.00250.x
- Fu, Y., Young, J. F., Rasmussen, M. K., Dalsgaard, T. K., Lametsch, R., Aluko, R. E., & Therkildsen, M. (2016). Angiotensin I-converting enzyme-inhibitory peptides from bovine collagen: Insights into inhibitory mechanism and transepithelial transport. Food Research International, 89, 373–381. doi: 10.1016/j.foodres.2016.08.037
- Fu, M., & Zhao, X. H. (2016). Structure and property changes of transglutaminase-induced modification of sodium caseinate in the presence of oligochitosan of 5 kDa. International Journal of Food Properties, 19, 2596–2607. doi: 10.1080/10942912.2015.1137937
- Fu, M., & Zhao, X. H. (2017). Modified properties of a glycated and cross-linked soy protein isolate by transglutaminase and an oligochitosan of 5 kDa. Journal of the Science of Food and Agriculture, 97, 58–64. doi: 10.1002/jsfa.7682
- Gauthier, S. F., Pouliot, Y., & Saint-Sauveur, D. (2006). Immunomodulatory peptides obtained by the enzymatic hydrolysis of whey proteins. International Dairy Journal, 16, 1315–1323. doi: 10.1016/j.idairyj.2006.06.014
- Gill, H. S., Doull, F., Rutherfurd, K. J., & Cross, M. L. (2000). Immunoregulatory peptides in bovine milk. British Journal of Nutrition, 84, S111–S117.
- Goossens, V., Grooten, J., De Vos, K., & Fiers, W. (1995). Direct evidence for tumor necrosis factor-induced mitochondrial reactive oxygen intermediates and their involvement in cytotoxicity. Proceedings of the National Academy of Sciences of the United States America, 92, 8115–8119. doi: 10.1073/pnas.92.18.8115
- Ha, Y. M., Chun, S. H., Hong, S. T., Koo, Y. C., Choi, H. D., & Lee, K. W. (2013). Immune enhancing effect of a Maillard-type lysozyme-galactomannan conjugate via signaling pathways. International Journal of Biological Macromolecules, 60, 399–404. doi: 10.1016/j.ijbiomac.2013.06.007
- Hao, L. X., & Zhao, X. H. (2016). Immunomodulatory potentials of the water-soluble yam (Dioscorea opposita Thunb) polysaccharides for the normal and cyclophosphamide-suppressed mice. Food and Agricultural Immunology, 27, 667–677. doi: 10.1080/09540105.2016.1148666
- Hartmann, R., & Meisel, H. (2007). Food-derived peptides with biological activity: From research to food applications. Current Opinion in Biotechnology, 18, 163–169. doi: 10.1016/j.copbio.2007.01.013
- Hong, P. K., Gottardi, D., Ndagijimana, M., & Betti, M. (2014). Glycation and transglutaminase mediated glycosylation of fish gelatin peptides with glucosamine enhance bioactivity. Food Chemistry, 142, 285–293. doi: 10.1016/j.foodchem.2013.07.045
- Hong, P. K., Ndagijimana, M., & Betti, M. (2016). Glucosamine-induced glycation of hydrolysed meat proteins in the presence or absence of transglutaminase: Chemical modifications and taste-enhancing activity. Food Chemistry, 197, 1143–1152. doi: 10.1016/j.foodchem.2015.11.096
- Hou, H., Fan, Y., Li, B. F., Xue, C. H., & Yu, G. L. (2012). Preparation of immunomodulatory hydrolysates from Alaska pollock frame. Journal of the Science of Food and Agriculture, 92, 3029–3038. doi: 10.1002/jsfa.5719
- Jiang, S. J., & Zhao, X. H. (2010). Transglutaminase-induced cross-linking and glucosamine conjugation in soybean protein isolates and its impacts on some functional properties of the products. European Food Research and Technology, 231, 679–689. doi: 10.1007/s00217-010-1319-2
- Jiang, S. J., & Zhao, X. H. (2011). Transglutaminase-induced cross-linking and glucosamine conjugation of casein and some functional properties of the modified product. International Dairy Journal, 21, 198–205. doi: 10.1016/j.idairyj.2010.12.004
- Jollès, P., Fiat, A., Migliore-Samour, D., Drouet, L., & Caen, J. (1992). Peptides from milk proteins implicated in antithrombosis and immunomodulation. In B. Renner & G. Sawatzki (Eds.), New perspectives in infant nutrition (pp. 160–172). New York, NY: Thieme Medical Press.
- Kim, S. Y., Je, J. Y., & Kim, S. K. (2007). Purification and characterization of antioxidant peptide from hoki (Johnius belengerii) frame protein by gastrointestinal digestion. Journal of Nutritional Biochemistry, 18, 31–38. doi: 10.1016/j.jnutbio.2006.02.006
- Kim, T. H., Lee, S. J., Rim, H. K., Shin, J. S., Jung, J. Y., Heo, J. S., … Lee, K. T. (2013). In vitro and in vivo immunostimulatory effects of hot water extracts from the leaves of Artemisia princeps Pampanini cv. Sajabal. Journal of Ethnopharmacology, 149, 254–262. doi: 10.1016/j.jep.2013.06.030
- Kleekayai, T., Harnedy, P. A., O’Keeffe, M. B., Poyarkov, A. A., CunhaNeves, A., Suntornsuk, W., & FitzGerald, R. J. (2015). Extraction of antioxidant and ACE inhibitory peptides from Thai traditional fermented shrimp pastes. Food Chemistry, 176, 441–447. doi: 10.1016/j.foodchem.2014.12.026
- Lee, J., Lee, S. J., & Lim, K. T. (2014). ZPDC glycoprotein (24 kDa) induces apoptosis and enhances activity of NK cells in N-nitrosodiethylamine-injected BALB/c. Cellular Immunology, 289, 1–6. doi: 10.1016/j.cellimm.2014.03.002
- Lin, S. Y., Liu, X. T., Liu, B. L., & Yu, Y. L. (2017). Optimization of pine nut (Pinus koraiensis) meal protein peptides on immunocompetence in innate and adaptive immunity response aspects. Food and Agricultural Immunology, 28, 109–120. doi: 10.1080/09540105.2016.1228835
- Liu, X. D., Zhou, B., Cao, R. B., Feng, X. L., Li, X. F., & Chen, P. Y. (2013). Comparison of immunomodulatory functions of three peptides from the chicken bursa of Fabricius. Regulatory Peptides, 186, 57–61. doi: 10.1016/j.regpep.2013.07.007
- Luft, F. C. (2008). Curbing the appetites of the big eaters. Journal of Molecular Medicine, 86, 351–352. doi: 10.1007/s00109-008-0321-7
- Mallet, J. F., Duarte, J., Vinderola, G., Anguenot, R., Beaulieu, M., & Matar, C. (2014). The immunopotentiating effects of shark-derived protein hydrolysate. Nutrition, 30, 706–712. doi: 10.1016/j.nut.2013.10.025
- Martins, S. I., Jongen, W. M., & van Boekel, M. A. (2000). A review of Maillard reaction in food and implications to kinetic modelling. Trends in Food Science and Technology, 11, 364–373. doi: 10.1016/S0924-2244(01)00022-X
- Martins, S. I., & van Boekel, M. A. (2005). A kinetic model for the glucose/glycine Maillard reaction pathways. Food Chemistry, 90, 257–269. doi: 10.1016/j.foodchem.2004.04.006
- Matsuda, T., Gu, J. X., Tsuruta, K., & Nakamura, R. (1985). Immunoreactive glycopeptides separated from peptic hydrolysate of chicken egg white ovomucoid. Journal of Food Science, 50, 592–594. doi: 10.1111/j.1365-2621.1985.tb13751.x
- Mercier, A., Gauthier, S. F., & Fliss, I. (2004). Immunomodulating effects of whey proteins and their enzymatic digests. International Dairy Journal, 14, 175–183. doi: 10.1016/j.idairyj.2003.08.003
- Mu, L. X., Zhao, H. F., Zhao, M. M., Cui, C., & Liu, L. Y. (2011). Physicochemical properties of soy protein isolates-acacia gum conjugates. Czech Journal of Food Science, 29, 129–136.
- Oliver, C. M., Melton, L. D., & Stanley, R. A. (2006). Creating protein with novel functionality via the Maillard reaction: A review. Critical Reviews in Food Science and Nutrition, 46, 337–350. doi: 10.1080/10408690590957250
- Parkin, J., & Cohen, B. (2001). An overview of the immune system. The Lancet, 357, 1777–1789. doi: 10.1016/S0140-6736(00)04904-7
- Park, H. Y., Yu, A. R., Choi, I. W., Hong, H. D., Lee, K. W., & Choi, H. D. (2013). Immunostimulatory effects and characterization of a glycoprotein fraction from rice bran. International Immunopharmacology, 17, 191–197. doi: 10.1016/j.intimp.2013.06.013
- Račaitytė, K., Kiessig, S., & Kálmán, F. (2005). Application of capillary zone electrophoresis and reversed-phase high-performance liquid chromatography in the biopharmaceutical industry for the quantitative analysis of the monosaccharides released from a highly glycosylated therapeutic protein. Journal of Chromatography A, 1079, 354–365. doi: 10.1016/j.chroma.2005.03.080
- Ramezani, R., Esmailpour, M., & Aminlari, M. (2008). Effect of conjugation with glucosamine on the functional properties of lysozyme and casein. Journal of the Science of Food and Agriculture, 88, 2730–2737. doi: 10.1002/jsfa.3400
- Renn, P. T., & Sathe, S. K. (1997). Effect of pH, temperature, and reactant molar ratio on L-leucine and D-glucose Maillard browning reaction in an aqueous system. Journal of Agricultural and Food Chemistry, 45, 3782–3787. doi: 10.1021/jf9608231
- Santiago-López, L., Hernández-Mendoza, A., Vallejo-Cordoba, B., Mata-Haro, V., & González-Córdova, A. (2016). Food-derived immunomodulatory peptides. Journal of the Science of Food and Agriculture, 96, 3631–3641. doi: 10.1002/jsfa.7697
- Schlimme, E., & Meisel, H. (1995). Bioactive peptides derived from milk proteins. Structural, physiological and analytical aspects. Molecular Nutrition and Food Research, 39, 1–20.
- Shu, M., Shen, W., Wang, X. J., Wang, F., Ma, L. X., & Zhai, C. (2015). Expression, activation and characterization of porcine trypsin in Pichia pastoris GS115. Protein Expression and Purification, 114, 149–155. doi: 10.1016/j.pep.2015.06.014
- Song, C. L., & Zhao, X. H. (2013). Rheological, gelling, and emulsifying properties of a glycosylated and cross-linked caseinate generated by transglutaminase. International Journal of Food Science and Technology, 48, 2595–2602. doi: 10.1111/ijfs.12255
- Song, C. L., & Zhao, X. H. (2014). Structure and property modification of oligochitosan- glycosylated and crosslinked soybean protein generated by microbial transglutaminase. Food Chemistry, 163, 114–119. doi: 10.1016/j.foodchem.2014.04.089
- Varela, B. Y. P., Arce, M. D. M., Marcos, A., & Castrillón, A. M. (1997). Immunocompetence in relation to a heat-processed diet (Maillard reaction) in weanling rats. British Journal of Nutrition, 77, 947–956. doi: 10.1079/BJN19970092
- Vivier, E., Tomasello, E., Baratin, M., Walzer, T., & Ugolini, S. (2008). Functions of natural killer cells. Nature Immunology, 9, 503–510. doi: 10.1038/ni1582
- Wang, M. C., Jiang, C. X., Ma, L. P., Zhang, Z. J., Cao, L., Liu, J., & Zeng, X. X. (2013). Preparation, preliminary characterization and immunostimulatory activity of polysaccharide fractions from the peduncles of Hovenia dulcis. Food Chemistry, 138, 41–47. doi: 10.1016/j.foodchem.2012.09.098
- Wang, X. P., & Zhao, X. H. (2017). Using an enzymatic galactose assay to detect lactose glycation extents of caseinate and soybean protein isolate via the Maillard reaction. Journal of the Science of Food and Agriculture. doi: 10.1002/jsfa.8084
- Yuan, C. F., Wang, C. D., Bu, Y. Q., Xiang, T. X., Huang, X. N., Wang, Z. W., … Song, F. Z. (2010). Antioxidative and immunoprotective effects of Pyracantha fortuneana (Maxim.) Li polysaccharides in mice. Immunology Letters, 133, 14–18. doi: 10.1016/j.imlet.2010.04.004
- Zhou, J. H., Ma, L. L., Xu, H. H., Gao, Y., Jin, Y. K., Zhao, L., … Zhang, S. H. (2014). Immunomodulating effects of casein-derived peptides QEPVL and QEPV on lymphocytes in vitro and in vivo. Food and Function, 5, 2061–2069. doi: 10.1039/C4FO00384E