ABSTRACT
Toll-like receptors (TLRs) play critical roles in the induction of immune and inflammatory responses by recognizing invading microbial pathogens. One of the most important proteins for inflammatory responses is inducible nitric oxide synthase (iNOS). The dysregulated iNOS activation play important roles in the development of certain inflammatory diseases. The present study investigated the effects of arachidic acid (ACA), which is a saturated fatty acid (SFA), and eicosapentanoic acid (EPA), which is polyunsaturated fatty acid (PUFA), on inflammation by modulating NF-κB activation and iNOS expression induced by TLRs agonists in murine macrophages. EPA suppressed NF-κB activation and iNOS expression induced by a lipopolysaccharide, macrophage-activating lipopeptide 2-kDa, and polyriboinosinic polyribocytidylic acid, but ACA did not. These results suggested that EPA can modulate TLR signaling pathways and subsequent chronic inflammatory responses, but ACA did not mediate these effects. All the results suggest that EPA is a promising novel agent for the treatment of inflammatory diseases.
GRAPHICAL ABSTRACT
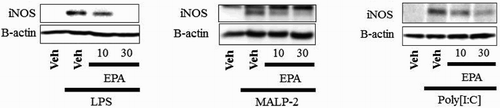
Introduction
Toll-like receptors (TLRs) play an important role in the induction of immune and inflammatory responses by recognizing invading microbial pathogens, including viruses, bacteria, protozoa, and fungi (Gu et al., Citation2013). The activation of TLRs by ligands can induce inflammatory responses for the development of many chronic inflammatory diseases (Medzhitov, Citation2001). Thirteen members of the TLR family have so far been identified in mammals. TLR4 recognizes lipopolysaccharide (LPS) of Gram-negative bacteria. TLR2 recognizes a variety of microbial components such as bacterial lipopeptides, peptidoglycan, and lipoteichoic acids. TLR5 recognizes bacterial flagellin. TLR3, TLR7, or TLR8 recognize viral double or single-stranded RNA. TLR9 recognizes bacterial and viral cytosine-phosphate-guanine dinucleotide DNA (CpG DNA). TLR11 and TLR12 recognize profilin protein in cases of Toxoplasma and TLR13 recognizes the bacterial ribosomal RNA sequence “CGGAAAGACC.” Broadly speaking, TLR agonists can activate two different downstream signaling pathways such as myeloid differentiation protein-88 (MyD88) or toll-interleukin-1 receptor domain-containing adapter inducing interferon-β (TRIF) (Ullah, Sweet, Mansell, Kellie, & Kobe, Citation2016). MyD88 and TRIF pathways activate transcription factors, such as nuclear factor-κB (NF-κB) and interferon regulatory factor 3/7 (IRF3/7), leading to the expression of inflammatory cytokine genes and subsequent immune responses (Shin & Youn, Citation2013).
Inflammation mediated by multiple molecular mechanisms refers to the pathological and physiological processes known to be involved in numerous diseases (Murakami & Ohigashi, Citation2007). One of the most important enzymes for inflammatory responses is inducible nitric oxide synthase (iNOS) that generates nitric oxide from the terminal guanidine nitrogen of L-arginine (Moncada, Citation1999). iNOS is a very important therapeutic target in the development of anti-inflammatory drugs due to dysregulated iNOS expression occurs in the development of certain inflammatory diseases (Kim et al., Citation2017; Vallance, Citation2003).
A fatty acid (FA) is a carboxylic acid with a long aliphatic hydrocarbon chain. Polyunsaturated fatty acids (PUFAs) contain more than one double bond in their structures. PUFAs are bioactive lipids that modulate inflammation and immunity. There are two main groups of PUFAs: n-6 PUFAs (linoleic acid and arachidonic acid) with their first double bond at C6, counting from the methyl C, and n-3 PUFA (alpha-linolenic acid, eicosapentanoic acid (EPA), and docosahexaenoic acid), with first unsaturated bond at C3. Accumulated evidence indicated that PUFAs have a variety of biological activities such as antioxidative, anti-inflammatory, and anticancer effects (Michalak, Mosinska, & Fichna, Citation2016). However, the underlying mechanisms remain to be elucidated. Therefore, we investigated whether arachidic acid (ACA) ((A)), which is a saturated fatty acid (SFA) and EPA ((B)), which is a PUFA with a 20-carbon chain, can modulate iNOS expression induced by several TLR agonists.
Figure 1. The structure of EPA and ACA. (A) EPA structure. (B) ACA structure. (C) RAW264.7 cells were treated with EPA (10, 30, 50 μM) or ACA (10, 30, 50 μM) for 4 h. Twenty microliters of the CellTiter 96® AQueous One Solution Reagent was added directly to culture wells. The plate was incubated at 37°C for 4 h in a humidified, 5% CO2 atmosphere. The absorbance was recorded at 490 nm with a 96-well plate reader. Veh, vehicle; EPA, eicosapentanoic acid; ACA, arachidic acid.
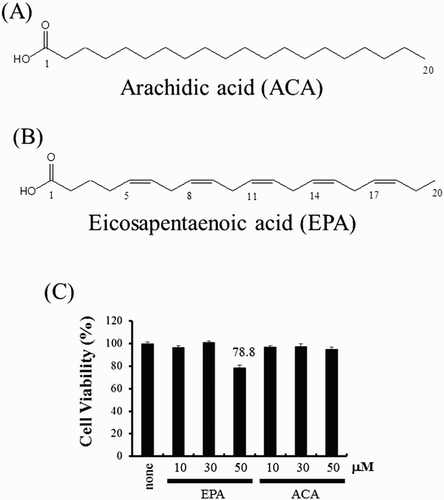
Materials and methods
Reagents
EPA and ACA were purchased from Sigma-Aldrich (St. Louis, MO, USA) and diluted in dimethyl sulfoxide (DMSO). LPS was obtained from List Biological Laboratories (San Jose, CA, USA) and macrophage-activating lipopeptide 2-kDa (MALP-2) was purchased from Alexis Biochemical (San Diego, CA, USA). Polyriboinosinic polyribocytidylic acid (poly[I:C]) was purchased from Amersham Biosciences (Piscataway, NJ, USA). All other reagents were purchased from Sigma-Aldrich (St. Louis, MO, USA) unless otherwise described.
Cell culture
RAW264.7 murine monocytic cells (TIB-71; American Type Culture Collection, Manassas, VA, USA) were cultured in Dulbecco’s modified Eagle’s medium containing 10% (v/v) fetal bovine serum, 100 units/mL penicillin, and 100 μg/mL streptomycin. Cells were maintained at 37°C in a 5% CO2 atmosphere unless otherwise stated.
Cell viability test
Cell viability was assessed by using a 3-(4,5-dimethylthiazol-2-yl)-5(3-carboxymethoxyphenyl)-2-(4-sulfophenyl)-2H-tetrazolium (MTS)-based colorimetric assay. Viability tests were performed using the CellTiter 96® AQueous One Solution Reagent assay kit (Promega, Madison, WI, USA) according to the manufacturer’s instructions.
Plasmid
NF-κB (2X)-luciferase reporter construct was from Frank Mercurio (Signal Pharmaceuticals, San Diego, CA) and iNOS-luciferase reporter construct was from Dr Daniel Hwang (University of California, Davis, CA). Heat-shock protein 70 (HSP70)-b-galactosidase reporter plasmid was from Robert Modulin (University of California, Los Angeles, CA). All DNA constructs were prepared in large scale for transfection using an EndoFree Plasmid Maxi kit (Qiagen, Valencia, CA).
Transfection and reporter gene luciferase assay
The assays were performed as previously described (Ahn, Kim, Hong, Gu, Shin, Jeong et al. Citation2016). Cells were co-transfected with a luciferase plasmid and a plasmid containing heat-shock protein (HSP)70-β-galactosidase as an internal control using the SuperFect transfection reagent (Qiagen, Valencia, CA, USA) according to the manufacturer’s instructions. Cell lysates were prepared, and luciferase enzyme activities were determined using a commercial Luciferase Assay System (Promega) according to the manufacturer’s instructions. Luciferase activity was normalized by β-galactosidase activity.
Western blot analysis
Western blotting was performed as previously described (Gu et al., Citation2015). Equal amounts of cell extracts were subjected to 8% sodium dodecyl sulfate-polyacrylamide gel electrophoresis, and the separated proteins were electrotransferred to a polyvinylidene difluoride membrane. The membrane was blocked to prevent nonspecific binding of antibody in phosphate-buffered saline containing 0.1% Tween-20 and 3% nonfat dry milk. Immunoblotting was performed with the indicated antibodies and secondary antibodies conjugated to horseradish peroxidase (Amersham Biosciences, Arlington Heights, IL, USA). The reactive bands were visualized with enhanced chemiluminescence Western blot detection reagents (Intron, Seongnam, Gyeonggi-do, South Korea).
Nitrite assay
Nitric oxide (NO) released from the RAW264.7 cells was assessed by determining the nitrite concentration in the culture supernatant. Samples (100 mL) of the culture medium were incubated with 150 mL of Griess reagent (1% sulfanilamide and 0.1% naphthylethylene diamine in a 2.5% phosphoric acid solution) at room temperature for 10 min in a 96-well microplate. The absorbance at 540 nm was read by using a plate reader. The concentration of NO was then determined by the preparation of a standard calibration curve, using sodium nitrite as the standard.
Data analysis
Data were obtained from triplicate experiments. Values were expressed as the mean ± standard error of the mean (SEM). Differences in the data were evaluated using Student’s t-test. A p-value less than .05 were taken as a statistically significant difference.
Results and discussion
EPA, but not ACA, suppresses NF-κB activation induced by TLR agonists
The cytotoxic nature of EPA or ACA was determined using the MTS-based viability assay in RAW264.7 cells. Cytotoxicity was assessed using a well-established viability assay based on the conversion of 3-(4,5-dimethylthiazol-2-yl)-5(3-carboxymethoxyphenyl)-2-(4-sulfophenyl)-2H-tetrazolium (MTS) to formazan. Treatment with 50 μM EPA reduced population viability by 78.8% ((C)). However, ACA was not cytotoxic at the concentration tested (10–50 μM). Toxicity of EPA would not be a cause of any observed changes in experiments under a concentration of 50 μM, therefore, all subsequent experiments utilized 30 μM EPA.
TLR signaling pathways can trigger the activation of NF-κB, which induces the activation of target genes involved in inflammation (Ahn, Kim, Hong, Gu, Shin, Paek et al. Citation2016; Hacker & Karin, Citation2006). Therefore, NF-κB activation induced by several TLR agonists was used as the readout for the activation of TLRs. The effects of EPA or ACA on NF-κB activation was determined by a NF-κB-luciferase reporter assay. EPA was observed to inhibit the activation of NF-κB induced by LPS (a TLR4 agonist), MALP-2 (a TLR2 and TLR6 agonist), or Poly[I:C] (TLR3 agonist) ((A–C)). However, ACA did not inhibit the activation of NF-κB induced by LPS (TLR4 agonist), MALP-2 (TLR2 and TLR6 agonist), or Poly[I:C] (TLR3 agonist) ((A–C)).
Figure 2. EPA, but not ACA, suppresses the activation of NF-κB induced by TLR agonists. (A–C) RAW 264.7 cells were transfected with a NF-κB-luciferase reporter plasmid and pretreated with EPA (10, 30 μM) or ACA (10, 30 μM) for 1 h and then treated with LPS (10 ng/mL) (A), MALP-2 (10 ng/mL) (B), or Poly[I:C] (10 μg/mL) (C) for an additional 8 h. Cell lysates were prepared, and luciferase enzyme activities were determined. Values represent the mean ± SEM (n = 3). *, Significantly different from LPS alone, p < .01 (**) (A). #, Significantly different from MALP-2 alone, p < .05 (#) (B). +, Significantly different from Poly[I:C] alone, p < .05 ( + ) (C). Veh, vehicle; EPA, eicosapentanoic acid; ACA, arachidic acid.
![Figure 2. EPA, but not ACA, suppresses the activation of NF-κB induced by TLR agonists. (A–C) RAW 264.7 cells were transfected with a NF-κB-luciferase reporter plasmid and pretreated with EPA (10, 30 μM) or ACA (10, 30 μM) for 1 h and then treated with LPS (10 ng/mL) (A), MALP-2 (10 ng/mL) (B), or Poly[I:C] (10 μg/mL) (C) for an additional 8 h. Cell lysates were prepared, and luciferase enzyme activities were determined. Values represent the mean ± SEM (n = 3). *, Significantly different from LPS alone, p < .01 (**) (A). #, Significantly different from MALP-2 alone, p < .05 (#) (B). +, Significantly different from Poly[I:C] alone, p < .05 ( + ) (C). Veh, vehicle; EPA, eicosapentanoic acid; ACA, arachidic acid.](/cms/asset/598b9946-a8a5-4380-8c60-eb05e077525f/cfai_a_1326468_f0002_b.gif)
EPA, but not ACA, suppresses iNOS expression induced by TLR agonists
It was next investigated whether EPA and ACA could regulate iNOS expression. Results from the iNOS-luciferase reporter assay in RAW264.7 cells was shown in . Cells were stimulated with LPS, MALP-2, or poly[I:C] and were incubated with EPA or ACA. EPA showed a dose-dependent inhibition of iNOS, but ACA did not inhibit iNOS expression ((A–C)). Cells were stimulated with LPS, MALP-2, or poly[I:C], and were then incubated with EPA or ACA. EPA also suppressed the iNOS protein that was induced by LPS, MALP-2, or poly[I:C], as determined by an iNOS immunoblotting assay ((A–C)). However, ACA did not inhibit iNOS protein expression ((A–C)). The nitrite assay in RAW264.7 cells was shown in . Cells were stimulated with LPS, MALP-2, or poly[I:C] and were then incubated with EPA or ACA. Incubation with increasing amounts of EPA resulted in a dose-dependent decrease of nitric oxide (NO) production, but that of ACA does not decrease of nitric oxide (NO) production ((A–C)). All the results suggested that EPA inhibited iNOS expression induced by several TLR agonists, but ACA does not inhibit iNOS expression.
Figure 3. EPA, but not ACA, suppresses iNOS expression induced by TLR agonists. (A–C) RAW 264.7 cells were transfected with iNOS-luciferase reporter plasmid and pretreated with EPA (10, 30 μM) or ACA (10, 30 μM) for 1 h and then treated with LPS (10 ng/mL) (A), MALP-2 (10 ng/mL) (B), or Poly[I:C] (10 μg/mL) (C) for an additional 8 h. Cell lysates were prepared, and luciferase enzyme activities were determined. Values represent the mean ± SEM (n = 3). *, Significantly different from LPS alone, p < .05 (*), p < .01 (**) (A). #, Significantly different from MALP-2 alone, p < .01 (##) (B). +, Significantly different from Poly[I:C] alone, p < .01 (++) (C). Veh, vehicle; EPA, eicosapentanoic acid; ACA, arachidic acid.
![Figure 3. EPA, but not ACA, suppresses iNOS expression induced by TLR agonists. (A–C) RAW 264.7 cells were transfected with iNOS-luciferase reporter plasmid and pretreated with EPA (10, 30 μM) or ACA (10, 30 μM) for 1 h and then treated with LPS (10 ng/mL) (A), MALP-2 (10 ng/mL) (B), or Poly[I:C] (10 μg/mL) (C) for an additional 8 h. Cell lysates were prepared, and luciferase enzyme activities were determined. Values represent the mean ± SEM (n = 3). *, Significantly different from LPS alone, p < .05 (*), p < .01 (**) (A). #, Significantly different from MALP-2 alone, p < .01 (##) (B). +, Significantly different from Poly[I:C] alone, p < .01 (++) (C). Veh, vehicle; EPA, eicosapentanoic acid; ACA, arachidic acid.](/cms/asset/d1ef462d-f0c4-4372-8c7b-4ff8d54c228b/cfai_a_1326468_f0003_b.gif)
Figure 4. EPA inhibits iNOS protein induced by TLRs agonists, but ACA does not. (A–C) RAW264.7 cells were pretreated with EPA (10, 30 μM) or ACA (10, 30 μM) for 1 h and then further stimulated with LPS (10 ng/mL) (A), MALP-2 (10 ng/mL) (B), or Poly[I:C] (10 μg/mL) (C) for an additional 8 h. Cell lysates were analyzed for iNOS and β-actin protein by immunoblots. Veh, vehicle; EPA, eicosapentanoic acid; ACA, arachidic acid.
![Figure 4. EPA inhibits iNOS protein induced by TLRs agonists, but ACA does not. (A–C) RAW264.7 cells were pretreated with EPA (10, 30 μM) or ACA (10, 30 μM) for 1 h and then further stimulated with LPS (10 ng/mL) (A), MALP-2 (10 ng/mL) (B), or Poly[I:C] (10 μg/mL) (C) for an additional 8 h. Cell lysates were analyzed for iNOS and β-actin protein by immunoblots. Veh, vehicle; EPA, eicosapentanoic acid; ACA, arachidic acid.](/cms/asset/b412f40c-f027-4df9-9332-04bdb7edfbbf/cfai_a_1326468_f0004_b.gif)
Figure 5. EPA, but not ACA, inhibits nitrite production induced by TLRs agonists. (A–C) RAW 264.7 cells were pretreated with EPA (10, 30 μM) or ACA (10, 30 μM) for 1 h and then treated with LPS (10 ng/mL) (A), MALP-2 (10 ng/mL) (B), or Poly[I:C] (10 μg/mL) (C) for an additional 20 h. The amounts of nitrite in the supernatant were measured using Griess reagent. Values represent the mean ± SEM (n = 3).*, Significantly different from LPS alone, p < .01 (**) (A). #, Significantly different from MALP-2 alone, p < .05 (#), p < .01 (##) (B). +, Significantly different from Poly[I:C] alone, p < .01 (++) (C). Veh, vehicle; EPA, eicosapentanoic acid; ACA, arachidic acid.
![Figure 5. EPA, but not ACA, inhibits nitrite production induced by TLRs agonists. (A–C) RAW 264.7 cells were pretreated with EPA (10, 30 μM) or ACA (10, 30 μM) for 1 h and then treated with LPS (10 ng/mL) (A), MALP-2 (10 ng/mL) (B), or Poly[I:C] (10 μg/mL) (C) for an additional 20 h. The amounts of nitrite in the supernatant were measured using Griess reagent. Values represent the mean ± SEM (n = 3).*, Significantly different from LPS alone, p < .01 (**) (A). #, Significantly different from MALP-2 alone, p < .05 (#), p < .01 (##) (B). +, Significantly different from Poly[I:C] alone, p < .01 (++) (C). Veh, vehicle; EPA, eicosapentanoic acid; ACA, arachidic acid.](/cms/asset/e409ca39-666b-464f-a1fc-903fdfbf6a3c/cfai_a_1326468_f0005_b.gif)
Broadly speaking, TLRs trigger two downstream signaling pathways, MyD88- and TRIF-dependent pathways (Takeda & Akira, Citation2005). All mammalian TLRs, except for TLR3, recruit MyD88, which activates the canonical I kappa B kinase (IKK) complex, leading to the activation of NF-κB. The activated NF-κB up-regulates the expression of inflammatory gene products including cytokines and chemokines (Takeda & Akira, Citation2005). In addition to the proinflammatory signals, TLR3 and TLR4 recruit TRIF, which activates two downstream kinases, a TNF receptor associated factor (TRAF) family member-associated NF-κB activator-binding kinase1 (TBK1) and an inducible IKK (IKKi)/IKKepsilon (IKKε), leading to the activation of interferon regulatory factor 3 (IRF3) (Fitzgerald et al., Citation2003). The representative target genes are regulated through the TRIF-dependent signaling pathway of TLRs, including IFN-β and IFN inducible genes, such as interferon-inducible protein-10 (IP-10) (Kawai et al., Citation2001).
Recent studies suggested that TLRs and its signaling components could be excellent therapeutic targets for chronic inflammatory diseases (Kawai & Akira, Citation2010). All TLRs, except for TLR3, induce iNOS expression mediated through the MyD88-dependent pathway, but TLR3 induces iNOS expression mediated through only the TRIF-dependent pathway. However, TLR4 can induce iNOS expression mediated through both the MyD88- and TRIF-dependent pathways. In this study, EPA inhibited iNOS expression induced by several TLR agonists, including a TLR3 agonist. This means that EPA can regulate both MyD88- and TRIF-dependent pathway of TLRs. Therefore, our results provide new insights into the mode of anti-inflammatory action of EPA.
Conclusions
Our study demonstrated that EPA suppressed the iNOS expression induced by TLR agonists, while ACA did not suppress iNOS expression. Recently, it was shown that PUFAs, unlike SFAs, have numerous benefits to human health (Deckelbaum & Torrejon, Citation2012; Lee et al., Citation2003). EPA, in particular, is a precursor of many substances such as DHA, prostaglandin-3, thromboxane-3, etc. In our experiments, EPA plays an important role in main inflammation downstream pathway for iNOS. These results suggest that PUFA is more effective in regulating of TLR downstream pathways than SFA.
Disclosure statement
No potential conflict of interest was reported by the authors.
Notes on contributors
Hyeon-Myeong Shin is a B.S. in Department of Biomedical Laboratory Science, College of Medical Sciences, SoonChunHyang University in Korea. Her main research project has been to identify the molecular targets of certain phytochemicals that are known to possess anti-inflammatory and chemopreventive effects.
Hyun-Jin Shim is a B.S. in Department of Biomedical Laboratory Science, College of Medical Sciences, SoonChunHyang University in Korea. Her main research project has been to identify the molecular targets of certain phytochemicals that are known to possess anti-inflammatory and chemopreventive effects.
Ah-Yeon Kim is a B.S. in Department of Biomedical Laboratory Science, College of Medical Sciences, SoonChunHyang University in Korea. Her main research project has been to identify the molecular targets of certain phytochemicals that are known to possess anti-inflammatory and chemopreventive effects.
Yoo Jung Lee is a B.S. in Department of Biomedical Laboratory Science, College of Medical Sciences, SoonChunHyang University in Korea. Her main research project has been to identify the molecular targets of certain phytochemicals that are known to possess anti-inflammatory and chemopreventive effects.
Hyung-Sun Youn is a professor of Soonchunhyang University in Korea. He got a Ph.D. from University of Alabama in 2012. He published more than 50 papers. His main research project has been to identify the molecular targets of certain phytochemicals that are known to possess anti-inflammatory and chemopreventive effects. He has been studying the regulation of downstream signaling pathways and target gene expression for Toll-like receptors (TLR). Identifying the direct targets of phytochemicals in TLR-mediated signaling pathways is the new direction of research in this area that would expand in the coming decade. Identifying natural products that can modulate signaling molecules in TLR signaling pathways can lead to discovery of chemopreventive and anti-diabetic agents.
Additional information
Funding
References
- Ahn, S. I., Kim, J. S., Hong, C. Y., Gu, G. J., Shin, H. M., Jeong, H. J., … Youn, H. S. (2016). 1-[4-Fluoro-2-(2-nitrovinyl)phenyl]pyrrolidine suppresses toll-like receptor 4 dimerization induced by lipopolysaccharide. Journal of Immunoassay and Immunochemistry, 37(3), 307–315. doi: 10.1080/15321819.2015.1135162
- Ahn, S. I., Kim, J. S., Hong, C. Y., Gu, G. J., Shin, H. M., Paek, J. H., … Youn, H. S. (2016). Eupatorium makinoi suppresses toll-like receptor signaling pathways. Food and Agricultural Imunology, 27(2), 242–250. doi: 10.1080/09540105.2015.1086315
- Deckelbaum, R. J., & Torrejon, C. (2012). The omega-3 fatty acid nutritional landscape: Health benefits and sources. Journal of Nutrition, 142(3), 587S–591S. doi: 10.3945/jn.111.148080
- Fitzgerald, K. A., Mcwhirter, S. M., Faia, K. L., Rowe, D. C., Latz, E., Golenbock, D. T., … Maniatis, T. (2003). IKKepsilon and TBK1 are essential components of the IRF3 signaling pathway. Nature Immunology, 4(5), 491–496. doi: 10.1038/ni921
- Gu, G. J., Ahn, S. I., Kim, J. S., Hong, C. Y., Lee, S. C., Chang, Y. T., … Youn, H. S. (2015). Suppression of the TRIF-dependent signaling pathway of toll-like receptor by CDr10b in RAW264.7 macrophages. International Immunopharmacology, 28(1), 29–33. doi: 10.1016/j.intimp.2015.05.017
- Gu, G. J., Eom, S. H., Suh, C. W., Koh, K. O., Kim, D. Y., & Youn, H. S. (2013). Suppression of TRIF-dependent signaling pathway of toll-like receptors by (E)-1-(2-(2-nitrovinyl)phenyl)pyrrolidine. European Journal of Pharmacolgy, 721(1–3), 109–115. doi: 10.1016/j.ejphar.2013.09.045
- Hacker, H., & Karin, M. (2006). Regulation and function of IKK and IKK-related kinases. Sci STKE, 357, re13.
- Kawai, T., & Akira, S. (2010). The role of pattern-recognition receptors in innate immunity: Update on toll-like receptors. Nat Immunol, 11(5), 373–384. doi: 10.1038/ni.1863
- Kawai, T., Takeuchi, O., Fujita, T., Inoue, J., Muhlradt, P. F., Sato, S., … Akira, S. (2001). Lipopolysaccharide stimulates the MyD88-independent pathway and results in activation of IFN-regulatory factor 3 and the expression of a subset of lipopolysaccharide-inducible genes. Journal of Immunology, 167(10), 5887–5894. doi: 10.4049/jimmunol.167.10.5887
- Kim, J. S., Shin, H. M., Kim, A. Y., Ahn, S. I., Shim, H. J., Nam, K. W., … Youn, H. S. (2017). Aster yomena suppresses LPS-induced cyclooxygenase-2 and inducible nitric oxide synthase expression. Food and Agricultural Immunology, 28(2), 202–210. doi: 10.1080/09540105.2016.1251395
- Lee, J. Y., Plakidas, A., Lee, W. H., Heikkinen, A., Chanmugam, P., Bray, G., & Hwang, D. H. (2003). Differential modulation of toll-like receptors by fatty acids: Preferential inhibition by n-3 polyunsaturated fatty acids. Journal of Lipid Research, 44(3), 479–486. doi: 10.1194/jlr.M200361-JLR200
- Medzhitov, R. (2001). Toll-like receptors and innate immunity. Nature Review Immunology, 1(2), 135–145. doi: 10.1038/35100529
- Michalak, A., Mosinska, P., & Fichna, J. (2016). Polyunsaturated fatty acids and their derivatives: Therapeutic value for inflammatory, functional gastrointestinal disorders, and colorectal cancer. Front Pharmacology, 7, 459–474. doi: 10.3389/fphar.2016.00459
- Moncada, S. (1999). Nitric oxide: Discovery and impact on clinical medicine. Journal of the Royal Society of Medicine, 92(4), 164–169. doi: 10.1177/014107689909200402
- Murakami, A., & Ohigashi, H. (2007). Targeting NOX, INOS and COX-2 in inflammatory cells: Chemoprevention using food phytochemicals. Internal Journal of Cancer, 121(11), 2357–2363. doi: 10.1002/ijc.23161
- Shin, H. J., & Youn, H. S. (2013). TBK1-targeted suppression of TRIF-dependent signaling pathway of toll-like receptors by Helenalin. Life Science, 93(22), 847–854. doi: 10.1016/j.lfs.2013.09.004
- Takeda, K., & Akira, S. (2005). Toll-like receptors in innate immunity. Internaional Immunology, 17(1), 1–14.
- Ullah, M. O., Sweet, M. J., Mansell, A., Kellie, S., & Kobe, B. (2016). TRIF-dependent TLR signaling, its functions in host defense and inflammation, and its potential as a therapeutic target. Journal of Leukocyte Biology, 100(1), 27–45. doi: 10.1189/jlb.2RI1115-531R
- Vallance, P. (2003). Nitric oxide: Therapeutic opportunities. Fundamental & Clinical Pharmacology, 17(1), 1–10. doi: 10.1046/j.1472-8206.2003.00124.x