ABSTRACT
In this study, a molecularly imprinted polymer that could selectively recognize trichlorfon was synthesized. Using the imprinted polymer as biomimetic antibody, a novel biomimetic immunoassay-capillary electrophoresis method with improved sensitivity was developed for the detection of trichlorfon. This method was based on the competitive reaction between quantum dot-labeled trichlorfon and free trichlorfon with the biomimetic antibody. Under optimal conditions, the limit of detection (IC15) and the sensitivity (IC50) of the method was 0.35 ± 0.021 μg/L and 0.81 ± 0.025 mg/L, respectively. To evaluate the accuracy of the method, trichlorfon spiked in the carrot and zucchini samples was determined, and the recovery was in the range of 80.1–95.3%. This method was also applied to the quantitative detection of trichlorfon residues in the edible rape and leek samples, and the results had a good correlation with those obtained using a gas chromatography method.
Introduction
Organophosphorus pesticides are widely used for prevention and control of plant diseases and insect pests, and they can easily enter the human body through skin and the respiratory tract. In recent years, organophosphorus pesticides have been paid much more attention globally because of their highly toxic and potentially carcinogenic properties (Zhao et al., Citation2014). To prevent these uncontrolled effects on human health, it is crucial to establish a rapid, accurate analysis method to detect organophosphorus pesticide residues.
There have been some reports regarding the detection methods for organophosphorus pesticide residues, such as gas chromatography (GC) coupled with tandem mass spectrometry (Chen, Chen, Feng, & Li, Citation2009; Pang et al., Citation2006), GC with flame photometric detection (Dugo, Bella, Torre, & Saitta, Citation2005; Liu et al., Citation2014), GC with nitrogen-phosphorus detection (Shen, Su, Zhu, & Gao, Citation2007; Tian, Liu, Fang, An, & Duan, Citation2014), high performance liquid chromatography (He & Lee, Citation2006; Seebunrueng, Santaladchaiyakit, & Srijaranai, Citation2014), liquid chromatography coupled with tandem mass spectrometry (Ingelse, van Dam, Vreeken, Mol, & Steijger, Citation2001; Li et al., Citation2013; Mol, van Dam, & Steijger, Citation2003) , chemiluminescence (Chen, Lin, Cai, Chen, & Wang, Citation2008; Li, He, & Xu, Citation2007; Li, Hu, Huo, & Xu, Citation2006; Wang, Zhang, Wang, Yang, & Zhang, Citation2001), capillary electrophoresis-quantum dot/laser induced detection (CE-QD/LIF) (Chen & Fung, Citation2010) and biosensor (Sun & Wang, Citation2010). However, these methods require expensive instruments and lengthy analytical times. The enzymatic inhibition method is also one of common methods to detect organophosphorus pesticide residue (Akkad & Schwack, Citation2010; Duford, Xi, & Salin, Citation2013; Salam et al., Citation2016), but the sensitivity of this method is not high, which needs the further qualitative and quantitative analysis by the GC, and the inhibiting degree of enzyme is affected by the amount of pesticides. As an alternative, immunoassay method has been developed and extensively used for rapid detection of organophosphates (Kim et al., Citation2011; Le, Zhu, & Yu, Citation2016; Liang, Liu, Liu, Yu, & Fan, Citation2008; Skerritt, Guihot, Asha, Bea, & Ngk, Citation2003; Wang, Tang, Fang, Pan, & Wang, Citation2011; Zhang, Wang, Yang, Du, & Lin, Citation2013).
Immunoassays, methods to determine trace levels in samples, have many advantages based on the specificity of the antigen–antibody reaction, such as high sensitivity, specificity, rapidity, low cost, and applicability to large numbers of samples (Li et al., Citation2014; Liu et al., Citation2012; Song et al., Citation2011). However, traditional immunoassay methods are difficult to perform because of the use of laboratory animals, the high cost and difficulties associated with antibody production, especially the sensitivity and instability of the biological antibody for the temperature and pH (Wang et al., Citation2009).
To overcome the difficulties of traditional immunoassay methods, many studies have been devoted to synthesizing antibody-like receptors. One of the most promising methods is molecular imprinting technology (Wang, Liu, Xu, Zhang, & Wang, Citation2007). The resulting molecularly imprinted polymer (MIP) has many advantages, such as good stability and high specific recognition ability. In recent years, certain biomimetic enzyme-linked immunoassay methods based on MIP have been studied (Fang et al., Citation2011; Lee et al., Citation2006; Meng, Qiao, Xu, Xin, & Wang, Citation2012; Sun, Xu, Ma, Qiao, & Xu, Citation2014; Tang et al., Citation2017; Tang, Fang, Wang, Sun, & Qian, Citation2013; Wang, Zhang, et al., Citation2011; Whitcombe, Kirsch, & Nicholls, Citation2014). However, natural enzymes are macromolecules, which have complicated structures and mutable characteristics. The selective recognition of MIP toward the enzyme-labeled antigen or antibody is very low, which weakens the competitive reaction and lowers the sensitivity of the method. Therefore, a marker that has a relatively small structure is necessary.
QDs, one of the nanometer-scale particles that consist of a semiconductor core, have garnered great scientific attention because of their narrow, symmetric, bright, and photostable fluorescent emission. Therefore, it is a better choice to make QDs as markers instead of enzymes for immunoassays. Furthermore, CE has proven to be an attractive analysis technique for various applications because of its major advantages including high efficiency and resolution in addition to low consumption of reagents and solvents (Gallartayala, Núñez, Moyano, & Galceran, Citation2010; Takeda et al., Citation2003; Zhao et al., Citation2014). Therefore, coupling CE with the biomimetic immunoassay technique can take advantage of the high separation efficiency of CE with the specificity of biomimetic immunoassay, and promote the sensitivity and accuracy of the biomimetic immunoassay (Li, Lu, Qiao, & Xu, Citation2016).
As one of the important organophosphorus pesticides and potent inhibitor of choline esterases, trichlorfon can over stimulate the nervous system and cause serious conditions including nausea, dizziness, and confusion, and respiratory paralysis and death at very high exposures (Sun et al., Citation2008). The target of this study was to present a new biomimetic immunoassay-capillary electrophoresis (BI-CE) method for determination of trichlorfon using MIP as the biomimetic antibody and QDs as the marker. The factors affecting the performance of this method were studied in detail, and the applicability and advantages of this developed method were evaluated.
Material and methods
Chemical and reagents
Fused-silica capillaries with 100 μm i.d. × 375 μm o.d. were purchased from the Yongnian Optic Fiber Plant (Hebei, China). The carrot and zucchini samples were purchased from the Aijia supermarket (Tai’an, China), and the edible rape and leek samples were purchased randomly from a vegetable market of Tai’an (Shandong, China), in January 2017.
Ethyleneglycol dimethacrylate (EGDMA) was supplied by Sigma-Aldrich (St. Louis, MO, USA). Methacrylic acid (MAA) and 2,2-azobisisobutyronitrile (AIBN) were purchased from Tianjin Chemical Reagent Factory (Tianjin, China), and MAA was vacuum distilled and AIBN was recrystallized before use. QDs were purchased from Jiayuan Quantum Dots Co., Ltd. (Wuhan, China). The analytical standard trichlorfon (99%) was obtained from the Institute for the Control of Agrochemicals of Ministry of Agriculture (Beijing, China). Double distilled water (DDW, 18.2 ΜΩ/cm) was obtained from an Aike ultrapure water instrument (Tangshi Kangning Technology Company, Chengdu, China) and used throughout the experiments. All other reagents and solvents used in this study were of the highest available purity and of analytical grade.
Solutions
The buffer solutions involving phosphate buffer saline (PBS), phosphate buffered saline with Tween-20 (PBS/T), and borate buffer saline (BBS) were prepared in DDW, and they were filtered through a 0.22-μm filter before use.
Apparatus
The CE experiments were performed on a P/ACE MDQ CE system (Beckman-Coulter, Fullerton, CA, USA) equipped with a photo-diode array detector. Data processing and acquisition were performed by Beckman 32 K software. The detection was operated at 195 nm, and the separation voltage was 25 kV. Before use, the capillary column was rinsed with 0.1 mol/L NaOH for 5 min and DDW for 5 min, and then with the running buffer solution, sodium borate solution for 20 min. Sample injection was conducted at 0.5 psi for 5 s, unless stated otherwise.
FT-IR spectra (4000–500 cm−1) in KBr were recorded using a Vector 22 spectrometer (Bruker, Karlsruhe, Germany). A DTG-60AH thermogravimetric analyzer (Shimadzu, Kyoto, Japan) was also used in this study.
A Shimadzu 2010 GC equipped with a flame photometric detector (Shimadzu, Kyoto, Japan) was used for the separation and determination of trichlorfon residue in vegetables. The separation was conducted on a RTX-1701 capillary column (30 m × 250 μm i.d. × 0.1-μm film thickness). Nitrogen was used as the carrier gas at a constant flow rate of 1.0 mL/min, and the injection volume was 1.0 μL. The injection port temperature was set at 180°C at the split mode with a split ratio of 2:1. The detector temperature was held constant at 250°C. The makeup flow rate was 30.0 mL/min.
Synthesis of trichlorfon hapten
Trichlorfon hapten was prepared following our previous study (Meng et al., Citation2012). Firstly, 5.664 g of trichlorfon (22 mmol) and 2.002 g of succinic anhydride (20 mmol) were dissolved with 5 mL of anhydrous pyridine, and the yellowish-brown dope formed after magnetic stirring for 10 min and placed in a dark room for 18 h. After purging pyridine with nitrogen, 50 mL of DDW was added, and the pH was adjusted to 8.0–9.0 with a NaHCO3 saturated solution. After extraction with ethyl acetate (3 × 30 mL), the organic fractions were evaporated to dryness under reduced pressure. The obtained crude product was dissolved in 50 mL of DDW.
The conjugate of trichlorfon hapten and QDs
The conjugate was prepared as follows: 72 μL of BBS (10 mM, pH 7.4) and 40 μL of trichlorfon hapten were added to the reaction container. The mixture was stirred for 5 min at room temperature. Then, 32 μL of 1-ethyl-3-(3-dimethylaminopropyl)carbodiimide (10 mM, dissolved in BBS) was added, and the mixture was stirred for 15 min. When 10 μL of NH2-QDs were added, the mixed solution reacted for 2 h at room temperature. Finally, the solution was ultrafiltered through a 0.22-μm filter for 5 times, and the end product was preserved at 2–8°C.
Synthesis of MIP
The MIP was prepared as follows: 0.257 g of trichlorfon (1.0 mmol) and 0.172 g of MAA (2.0 mmol) were dissolved in 3.0 mL of chloroform. After stirring for 30 min at room temperature, 0.754 mL of EGDMA (4 mmol) and 20.0 mg of AIBN were added, and the mixed solution was ultrasonicated and purged with nitrogen for 15 min, respectively. Then, the solution was incubated in a water bath at 58°C for 18 h. When the polymerization process ended, the rigid polymer was crushed and sieved with a 200-mesh sieve. The MIP particles were extracted with methanol/acetic acid (120/40, v/v) for 24 h, followed by 160 mL of methanol for 12 h. Finally, the polymer was dried in a vacuum oven at 60°C for 12 h.
For comparison, a non-imprinted polymer (NIP) was synthesized in the same way except for the addition of trichlorfon.
Procedure of QD-BI-CE
In this study, trichlorfon (Ag) competed with trichlorfon hapten labeled with QDs (Ag*) to bind to a limited amount of antibody, MIP (Ab). The reaction process was as follows: 5 mL of Ag* (diluted to 1:300 with BBS) was mixed with 5 mL of trichlorfon standard solution or sample extract. When 5.0 mg of MIP was added, the mixture solution was shaken for 80 min at room temperature. After centrifugation (5000 r/min) for 20 min, the supernatant was filtered with a 0.22-μm filter membrane and injected into CE for analysis.
For a comparison, a NIP was used in place of the MIP for the above procedure.
Sample preparation
To check the accuracy of the QD-BI-CE method, the carrot and zucchini samples spiked with trichlorfon were detected by this method. The specific steps were as follows: the carrot and zucchini were cut into slices, and 1.0 g of each was separately weighed into 100-mL beakers, and then spiked with 1.0 mL of trichlorfon standard solution (0.5 mg/L, 1.0 mg/L, and 5.0 mg/L). After incubation for 1 h at room temperature, the spiked samples were ultrasonicated with 3 × 10 mL DDW for 30 min. After filtration with a 0.22-μm filter membrane, the extractions were transferred to a 50-mL volumetric flask, and then diluted by DDW to 50 mL. Finally, the resulting extractions were used for the QD-BI-CE procedure, and the recoveries were calculated.
To detect the concentrations of trichlorfon in edible rape and leek samples, 1.0 g of samples were separately weighted into 100-mL beakers and prepared according to the above process except for the addition of trichlorfon. Finally, the trichlorfon level was calculated.
GC analysis
To identify the accuracy of the QD-BI-CE method, the edible rape and leek samples were determined by GC according to the method described in GB/T 5009.20–2003 (China). Briefly, 50.0 g of the edible rape and leek samples were separately weighed into a 300-mL beaker and mashed. Then, 50 mL of DDW and 100 mL of acetone were added. The mixture was ultrasonicated for 20 min. After filtration, 100 mL of the filtrate was transferred to a 500-mL separatory funnel. After 10 g of sodium chloride was added, the separatory funnel was shaken vigorously for 3 min. The aqueous phase was separated again by adding 2 × 50 mL of dichloromethane. All of the organic phases were collected. After being condensed by a rotary evaporator, the resulting extraction was diluted to 10 mL using chromatography grade dichloromethane. Finally, the extractions were filtered using a 0.22-μm filter membrane, and 1.0 μL of the filtrate was injected into the GC for analysis.
Statistical analysis
The ANOVA (SAS Institute, Cary, NC, USA) test was performed to evaluate the difference between the results obtained by the QD-BI-CE and GC methods.
Results and discussion
Characterization of MIP
FT-IR spectra analysis
The FT-IR spectra of trichlorfon (a), the NIP (b), the imprinted polymer without extraction (c), and the imprinted polymer after extraction (d) are compared in . For the imprinted polymer without extraction and imprinted polymer after extraction, the observed features around 2957 and 2954 cm−1 indicated a C–H stretch, and the features around 1723 and 1725 cm−1 indicated a C=O stretch, which resulted from the COOH of MAA. For the FT-IR spectra of trichlorfon and the polymer without extraction, the features around 1030 and 1040 cm−1 indicated the presence of a P=O bond of trichlorfon (Meng et al., Citation2011), and the stretch shift in the position of the spectrum can be attributed to the hydrophobic interaction between the −OH group of MAA and the P=O group of trichlorfon (Xu, Fang, & Wang, Citation2010). These results showed that trichlorfon reacted with MAA, and the MIP was synthesized.
Thermogravimetric analysis
Thermogravimetric analysis of the MIP is shown in . When the temperature was increased from 0°C to 338°C, the weight of the MIP did not have an obvious decrease. However, the weight of the MIP did decrease with increasing temperature when the heating temperature was higher than 338°C. These results demonstrated that the synthesized MIP had good thermal stability at room temperature.
Evaluation of adsorption ability of MIP
To evaluate the adsorption ability of the MIP, the adsorption capacity (Q) of the MIP and NIP was calculated according to the following equation (Gong, Yu, Meng, Hu, & He, Citation2004):
where C0 and C1 are the concentrations of trichlorfon in solution before and after adsorption, respectively, V is the volume of the solution, and M is the mass of the MIP or NIP. The adsorption isotherms of the MIP and NIP are displayed in . As seen in , the adsorption capacities of MIP and NIP template molecules increased with increasing concentration of trichlorfon, respectively. The MIP exhibited a higher adsorption capacity towards trichlorfon, which was approximately 1.79 times more than that of NIP at 600 mg/L. These results showed that the MIP had specific adsorption ability for trichlorfon.
Optimization of the QD-BI-CE method
To improve the precision and sensitivity of the QD-BI-CE method, the experimental conditions, such as the diluted concentration of Ag*, competitive reaction time, and buffer solution composition, were optimized.
The absorption of MIP for Ag* was affected by the diluted concentration of Ag*. In this study, 5.0 mL of different concentrations of Ag* (diluted to 1/100, 1/200, 1/300, 1/400, and 1/500) were mixed with 5 mL of trichlorfon standard solution (0.1 mg/L) to carry out the competitive reaction. As shown in (a), the peak area of Ag* decreased with decreasing concentration of Ag*. A relatively moderate concentration (dilution ratio at 1/300) was chosen as the experimental work concentration.
Figure 4. (a) The peak area of Ag* at different Ag* diluted concentration ratios, (b) the influence of the competitive reaction time on trichlorfon inhibition, (c) the QD-BI-CE standard curves of trichlorfon using the MIP as the antibody at concentrations of 0.1–10,000 μg/L in PBS, PBS/T, BBS, and DDW solutions.
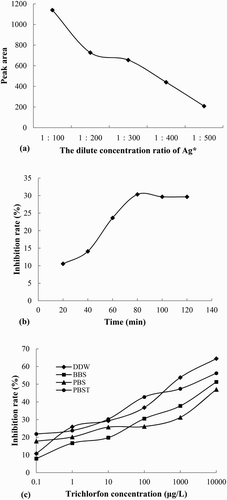
The competitive reaction time can affect the combination of Ag and Ab. The effect of different competitive reaction times (20, 40, 60, 80, 100 and 120 min) on the QD-BI-CE method was investigated. The inhibition ratio became larger as the reaction time increased until the reaction time was 80 min ((b)), which indicated that the adsorption had reached equilibrium at 80 min. Therefore, 80 min was selected as the reaction time.
The buffer solution used for the preparation of the standard solution and samples affected the recognition ability and adsorption capacity of the MIP toward the target molecules, which directly affected the sensitivity of the method. In this study, different buffer solutions involving PBS, phosphate buffered saline with Tween-20 (PBS/T), BBS and DDW were investigated ((c)). Experimental results indicated that in the DDW system, we obtained a better inhibition ratio and higher sensitivity. Therefore, DDW was chosen as the buffer solution.
Selectivity of the QD-BI-CE method
With the MIP and NIP respectively as the antibody, the QD-BI-CE standard curves for different trichlorfon concentrations from 0.1 to 10,000 μg/L were established under optimal experimental conditions (). The results showed that the MIP had a higher inhibition rate compared to the NIP at the same concentrations of trichlorfon. With the MIP as Ab, the inhibition rate reached 64.45% when the concentration of trichlorfon was 10,000 μg/L, while the inhibition rate was only 34.37% when the NIP was used as Ab.
Figure 5. The QD-BI-CE standard curves of trichlorfon using the MIP and NIP as the antibody at concentrations of 0.1–10,000 μg/L in a DDW solution.
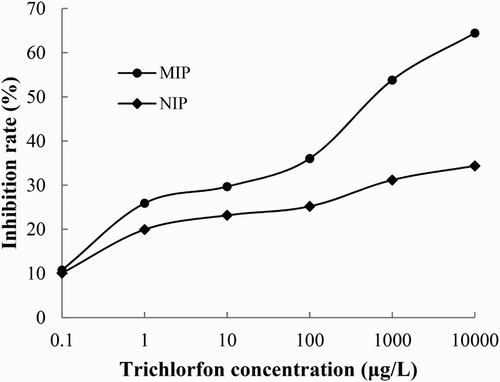
Cross-reactivity experiments can evaluate the specificity of the QD-BI-CE method. Monocrotophos and acephate, two structurally analogous compounds of trichlorfon, were chosen for the cross-reactivity experiments. The results in Figure S1 and indicated that MIP had a higher selectivity for trichlorfon than the other structural analogous compounds, monocrotophos and acephate, and lower cross-reactivities were obtained, which were 14.2% and 13.7% for monocrotophos and acephate, respectively.
Table 1. The pesticide structure, IC50 and cross-reactivity (CR) of the trichlorfon and structural analogous compounds of monocrotophos and acephate.
Analytical parameters of the QD-BI-CE method
The analytical parameters of the developed method for the determination of trichlorfon were evaluated under optimal conditions. The limit of detection (LOD, IC15) and sensitivity (IC50) of the method were 0.35 ± 0.021 μg/L and 0.81 ± 0.025 mg/L, respectively. According to the “Regulation (EC) No 396/2005 of the European Parliament and of the Council”, the maximum residue level of trichlorfon in primary products is 50 μg/L. Therefore, this method is sensitive enough to be used for the determination of trichlorfon.
Accuracy and applicability evaluation of the QD-BI-CE method
To evaluate the accuracy of the proposed method, the carrot and zucchini samples spiked with trichlorfon at 0.5, 1.0, and 5.0 mg/L levels were extracted and analyzed by this method. For each concentration, measurements were performed in triplicate. The analytical data are shown in . Good recovery was achieved, which was from 80.1% to 95.3%.
Table 2. The recoveries of QDs-BI-CE method for the determination of spiked trichlorfon in the carrot and zucchini samples (n = 3).
To evaluate the practical application of the QD-BI-CE method, trichlorfon residues in edible rape and leek samples were detected (Figure S2). Trichlorfon was quantitatively detected to be 0.027 ± 0.001 mg/kg and 0.507 ± 0.025 mg/kg in the edible rape and leek samples, respectively. In addition, a comparative analysis of the edible rape and leek samples was performed by GC (Figure S3), which detected 0.023 ± 0.001 mg/kg and 0.541 ± 0.022 mg/kg of trichlorfon, respectively. There was no significant difference between the results obtained by the above two methods (P > 0.05), which proved that the QD-BI-CE method had good accuracy and application for the determination of trichlorfon in agricultural products.
Advantages of the proposed method
Compared with the reported methods, the results obtained in this study suggested that the QD-BI-CE method has many advantages. First, the structure of QDs is small, so using QDs as a marker can enhance the competitive reaction, subsequently improving the sensitivity of the proposed method, which was much higher than that of the biomimetic enzyme-linked immunossay studied by Meng et al. (6.8 mg/L). Second, the LOD of this method is comparable to that of GC (Shen et al., Citation2007) and is lower than that of the reported CE-QD/LIF method (Chen & Fung, Citation2010). Therefore, our method has potential to be used as a tool to detect trace levels of trichlorfon in food samples. Third, a cycle of this method lasts 110 min, while a complete procedure of the traditional chromatography methods is usually 180 min, so our procedure is more efficient. Finally, MIP used as the antibody is stable and can be reused for eight times without a loss in sensitivity, which also reduces the costs of analysis.
Conclusion
In this study, we developed a BI-CE method based on QD labels, and this method was successfully applied to the determination of trichlorfon in vegetables. With good accuracy and improved sensitivity, our study has provided a new analytical method for the quick determination of organophosphorous pesticide residues in food.
Disclosure statement
No potential conflict of interest was reported by the authors.
Notes on contributors
Mingdi Jiang obtained her bachelor’s degree from Shandong Agricultural University, Tai’an, China in 2016. Her research interests are food immunoassay detection methods.
Shang Wu will get his bachelor in Shandong Agricultural University (2018), Tai’an, China. His research interests are immunoassay.
Longhua Xu got her PhD in Food science in 2016 from Tianjin University of Science and Technology, Tianjin, China and then became a faculty in College of Food Science and Engineering, Shandong Agricultural University. Her research interests are molecularly imprinted polymers design and application.
Xuguang Qiao was born in 1965, is now a Professor in College of Food Science and Engineering, Shandong Agricultural University. His research interests focus on f vegetable processing technology, and food quality control.
Zhixiang Xu was born in 1973, is now a Professor in College of Food Science and Engineering, Shandong Agricultural University. His research interests focus on tailored (bio) molecular recognition interfaces, molecularly imprinted materials, and biomimetic immunosorbent assay.
Additional information
Funding
References
- Akkad, R., & Schwack, W. (2010). Multi-enzyme inhibition assay for the detection of insecticidal organophosphates and carbamates by high-performance thin-layer chromatography applied to determine enzyme inhibition factors and residues in juice and water samples. Journal of Chromatography B – Analytical Technologies in the Biomedical and Life Sciences, 878(17–18), 1337–1345. doi: 10.1016/j.jchromb.2009.12.021
- Chen, H., Chen, R. W., Feng, R., & Li, S. Q. (2009). Simultaneous analysis of carbamate and organophosphorus pesticides in water by single-drop microextraction coupled with GC-MS. Chromatographia, 70(1), 165–172. doi: 10.1365/s10337-009-1154-4
- Chen, Q., & Fung, Y. (2010). Capillary electrophoresis with immobilized quantum dot fluorescence detection for rapid determination of organophosphorus pesticides in vegetables. Electrophoresis, 31(18), 3107–3114. doi: 10.1002/elps.201000260
- Chen, X. M., Lin, Z. J., Cai, Z. M., Chen, X., & Wang, X. R. (2008). Electrochemiluminescence detection of dichlorvos pesticide in luminol-CTAB medium. Talanta, 76, 1083–1087. doi: 10.1016/j.talanta.2008.05.007
- Duford, D. A., Xi, Y., & Salin, E. D. (2013). Enzyme inhibition-based determination of pesticide residues in vegetable and soil in centrifugal microfluidic devices. Analytical Chemistry, 85(16), 7834–7841. doi: 10.1021/ac401416w
- Dugo, G., Bella, G. D., Torre, L. L., & Saitta, M. (2005). Rapid GC-FPD determination of organophosphorus pesticide residues in Sicilian and Apulian olive oil. Food Control, 16(5), 435–438. doi: 10.1016/j.foodcont.2004.05.003
- Fang, G., Lu, J., Pan, M., Li, W., Ren, L., & Wang, S. (2011). Substitution of antibody with molecularly imprinted film in enzyme-linked immunosorbent assay for determination of trace ractopamine in urine and pork samples. Food Analytical Methods, 4(4), 590–597. doi: 10.1007/s12161-011-9206-4
- Gallartayala, H., Núñez, O., Moyano, E., & Galceran, M. T. (2010). Field-amplified sample injection-micellar electrokinetic capillary chromatography for the analysis of bisphenol A, bisphenol F, and their diglycidyl ethers and derivatives in canned soft drinks. Electrophoresis, 31(9), 1550–1559. doi: 10.1002/elps.200900606
- Gong, S., Yu, Z., Meng, L., Hu, L., & He, Y. (2004). Dye-molecular-imprinted polysiloxanes. II. Preparation, characterization, and recognition behavior. Journal of Applied Polymer Science, 93(2), 637–643. doi: 10.1002/app.20486
- He, Y., & Lee, H. K. (2006). Continuous flow microextraction combined with high-performance liquid chromatography for the analysis of pesticides in natural waters. Journal of Chromatography A, 1122(1–2), 7–12. doi: 10.1016/j.chroma.2006.04.078
- Ingelse, B. A., van Dam, R. C., Vreeken, R. J., Mol, H. G., & Steijger, O. M. (2001). Determination of polar organophosphorus pesticides in aqueous samples by direct injection using liquid chromatography-tandem mass spectrometry. Journal of Chromatography A, 918(1), 67–78. doi: 10.1016/S0021-9673(01)00660-4
- Kim, Y. A., Lee, E. H., Kim, K. O., Lee, Y. T., Hammock, B. D., & Lee, H. S. (2011). Competitive immunochromatographic assay for the detection of the organophosphorus pesticide chlorpyrifos. Analytica Chimica Acta, 693(1–2), 106–113. doi: 10.1016/j.aca.2011.03.011
- Le, T., Zhu, L., & Yu, H. (2016). Dual-label quantum dot-based immunoassay for simultaneous determination of carbadox and olaquindox metabolites in animal tissues. Food Chemistry, 199, 70–74. doi: 10.1016/j.foodchem.2015.11.116
- Lee, W. Y., Lee, E. K., Kim, Y. J., Park, W. C., Chung, T., & Yong, T. L. (2006). Monoclonal antibody-based enzyme-linked immunosorbent assays for the detection of the organophosphorus insecticide isofenphos. Analytica Chimica Acta, 557(1–2), 169–178. doi: 10.1016/j.aca.2005.10.007
- Li, B., He, Y., & Xu, C. (2007). Simultaneous determination of three organophosphorus pesticides residues in vegetables using continuous-flow chemiluminescence with artificial neural network calibration. Talanta, 72(1), 223–230. doi: 10.1016/j.talanta.2006.10.023
- Li, X., Hu, Y., Huo T., & Xu C. (2006). Comparison of the determination of chloramphenicol residues in aquaculture tissues by time-resolved fluoroimmunoassay and with liquid chromatography and tandem mass spectrometry. Food and Agricultural Immunology, 17(3–4), 191–199. doi: 10.1080/09540100601090349
- Li, M., Jin, Y., Li, H. F., Hashi, Y., Ma, Y., & Lin, J. M. (2013). Rapid determination of residual pesticides in tobacco by the quick, easy, cheap, effective, rugged, and safe sample pretreatment method coupled with LC-MS. Journal of Separation Science, 36(15), 2522–2529. doi: 10.1002/jssc.201201091
- Li, J., Lu, J., Qiao, X., & Xu, Z. (2016). A study on biomimetic immunoassay-capillary electrophoresis method based on molecularly imprinted polymer for determination of trace trichlorfon residue in vegetables. Food Chemistry, 221, 1285–1290. doi: 10.1016/j.foodchem.2016.11.028
- Li, X., Zhang, H., Ji, Y., Zheng, Z., Bian, Q., & Zhu, G. (2014). Immunochemical and molecular characteristics of monoclonal antibodies against organophosphorus pesticides and effect of hapten structures on immunoassay selectivity. Food and Agricultural Immunology, 26(1), 109–119. doi: 10.1080/09540105.2013.873390
- Liang, Y., Liu, X. J., Liu, Y., Yu, X. Y., & Fan, M. T. (2008). Synthesis of three haptens for the class-specific immunoassay of O,O-dimethyl organophosphorus pesticides and effect of hapten heterology on immunoassay sensitivity. Analytica Chimica Acta, 615(2), 174–183. doi: 10.1016/j.aca.2008.03.050
- Liu, B., Ge, Y., Zhang, Y., Song, Y., Lv, Y., & Wang, X. (2012). Production of the class-specific antibody and development of direct competitive elisa for multi-residue detection of organophosphorus pesticides. Food and Agricultural Immunology, 23(2), 157–168. doi: 10.1080/09540105.2011.608120
- Liu, H., Kong, W., Qi, Y., Gong, B., Miao, Q., Wei, J., & Yang, M. (2014). Streamlined pretreatment and gc-fpd analysis of multi-pesticide residues in perennial morinda roots: A tropical or subtropical plant. Chemosphere, 95(1), 33–40. doi: 10.1016/j.chemosphere.2013.07.085
- Meng, L., Qiao, X., Song, J., Xu, Z., Xin, J., & Zhang, Y. (2011). Study of an online molecularly imprinted solid phase extraction coupled to chemiluminescence sensor for the determination of trichlorfon in vegetables. Journal of Agricultural and Food Chemistry, 59(24), 12745–12751. doi: 10.1021/jf203801n
- Meng, L., Qiao, X., Xu, Z., Xin, J., & Wang, L. (2012). Development of a direct competitive biomimetic enzyme-linked immunosorbent assay based on a hydrophilic molecularly imprinted membrane for the determination of trichlorfon residues in vegetables. Food Analytical Methods, 5(5), 1229–1236. doi: 10.1007/s12161-012-9366-x
- Mol, H. G., van Dam, R. C., & Steijger, O. M. (2003). Determination of polar organophosphorus pesticides in vegetables and fruits using liquid chromatography with tandem mass spectrometry: Selection of extraction solvent. Journal of Chromatography A, 1015(1–2), 119–127. doi: 10.1016/S0021-9673(03)01209-3
- Pang, G. F., Liu, Y. M., Fan, C. L., Zhang, J. J., Cao, Y. Z., Li, X. M., … Guo, T. T. (2006). Simultaneous determination of 405 pesticide residues in grain by accelerated solvent extraction then gas chromatography-mass spectrometry or liquid chromatography-tandem mass spectrometry. Analytical and Bioanalytical Chemistry, 384(6), 1366–1408. doi: 10.1007/s00216-005-0237-9
- Salam, F., Puat, N. H. A., Rahman, G. A., Ramli, S. N. M., Mohamed, K. A., & Kadir, A. A. A. (2016). Comparative study of pesticides analysis using enzyme inhibition sensor and gas chromatography methods. Procedia Chemistry, 20, 33–39. doi: 10.1016/j.proche.2016.07.005
- Seebunrueng, K., Santaladchaiyakit, Y., & Srijaranai, S. (2014). Vortex-assisted low density solvent based demulsified dispersive liquid–liquid microextraction and high-performance liquid chromatography for the determination of organophosphorus pesticides in water samples. Chemosphere, 103(5), 51–58. doi: 10.1016/j.chemosphere.2013.11.024
- Shen, X., Su, Q., Zhu, X., & Gao, Y. (2007). Determination of pesticide residues in soil by modified matrix solid-phase dispersion and gas chromatography. Annali Di Chimica, 97(8), 647–653. doi: 10.1002/adic.200790049
- Skerritt, J. H., Guihot, S. L., Asha, M. B., Bea, R., & Ngk, K. (2003). Sensitive immunoassays for methyl-parathion and parathion and their application to residues in foodstuffs. Food and Agricultural Immunology, 15(15), 1–15. doi: 10.1080/0954010031000138069
- Song, C., Liu, Q., Zhi, A., Yang, J., Zhi, Y., Li, Q., Hu, X., … Zhang, G. (2011). Development of a lateral flow colloidal gold immunoassay strip for the rapid detection of olaquindox residues. Journal of Agricultural and Food Chemistry, 59(17), 9319–9326. doi: 10.1021/jf202213m
- Sun, F., Betzendahl, I., Van, W. K., Cortvrindt, R., Smitz, J., Pacchierotti, F., & Eichenlaub-Ritter, U. (2008). Trichlorfon-induced polyploidy and nondisjunction in mouse oocytes from preantral follicle culture. Mutation Research/Genetic Toxicology & Environmental Mutagenesis, 651(102), 114–124. doi: 10.1016/j.mrgentox.2007.10.008
- Sun, X., & Wang, X. (2010). Acetylcholinesterase biosensor based on Prussian blue-modified electrode for detecting organophosphorous pesticides. Biosensors & Bioelectronics, 25(12), 2611–2614. doi: 10.1016/j.bios.2010.04.028
- Sun, Q., Xu, L., Ma, Y., Qiao, X., & Xu, Z. (2014). Study on a biomimetic enzyme-linked immunosorbent assay method for rapid determination of trace acrylamide in French fries and cracker samples. Journal of the Science of Food & Agriculture, 94(1), 102–108. doi: 10.1002/jsfa.6204
- Takeda, S., Omura, A., Chayama, K., Tsuji, H., Fukushi, K., Yamane, M., … Terabe, S. (2003). Separation and on-line concentration of bisphenol A and alkylphenols by micellar electrokinetic chromatography with anionic surfactant. Journal of Chromatography A, 1014(1–2), 103–107. doi: 10.1016/S0021-9673(03)01126-9
- Tang, Y., Fang, G., Wang, S., Sun, J., & Qian, K. (2013). Rapid determination of metolcarb residues in foods using a biomimetic enzyme-linked immunosorbent assay employing a novel molecularly imprinted polymer film as artificial antibody. Journal of Aoac International, 96(2), 453–458. doi: 10.5740/jaoacint.10-387
- Tang, Y., Gao, J., Liu, X., Gao, X., Ma, T., Lu, X., & Li, J. (2017). Ultrasensitive detection of clenbuterol by a covalent imprinted polymer as a biomimetic antibody. Food Chemistry, 228, 62–69. doi: 10.1016/j.foodchem.2017.01.102
- Tian, F., Liu, W., Fang, H., An, M., & Duan, S. (2014). Determination of six organophosphorus pesticides in water by single-drop microextraction coupled with GC-NPD. Chromatographia, 77(5), 487–492. doi: 10.1007/s10337-013-2609-1
- Wang, X. H., Liu, T., Xu, N., Zhang, Y., & Wang, S. (2007). Enzyme-linked immunosorbent assay and colloidal gold immunoassay for ochratoxin A: Investigation of analytical conditions and sample matrix on assay performance. Analytical and Bioanalytical Chemistry, 389(3), 903–911. doi: 10.1007/s00216-007-1506-6
- Wang, J., Tang, W., Fang, G., Pan, M., & Wang, S. (2011). Development of a biomimetic enzyme-linked immunosorbent assay method for the determination of methimazole in urine sample. Journal of the Chinese Chemical Society, 58(4), 463–469. doi: 10.1002/jccs.201190007
- Wang, S., Xu, Z., Fang, G., Zhang, Y., Liu, B., & Zhu, H. (2009). Development of a biomimetic enzyme-linked immunosorbent assay method for the determination of estrone in environmental water using novel molecularly imprinted films of controlled thickness as artificial antibodies. Journal of Agricultural & Food Chemistry, 57(11), 4528–4534. doi: 10.1021/jf900505k
- Wang, J., Zhang, C., Wang, H., Yang, F., & Zhang, X. (2001). Development of a luminol-based chemiluminescence flow-injection method for the determination of dichlorvos pesticide. Talanta, 54(6), 1185–1193. doi: 10.1016/S0039-9140(01)00388-5
- Wang, L., Zhang, L. J., Wei, L. V., Han, S. H., Zhang, F. K., & Pan, J. R. (2011). Determination of organophosphorus pesticides based on biotin-avidin enzyme-linked immunosorbent assay. Chinese Journal of Analytical Chemistry, 39(3), 346–350. doi: 10.1016/S1872-2040(10)60425-2
- Whitcombe, M. J., Kirsch, N., & Nicholls, I. A. (2014). Molecular imprinting science and technology: A survey of the literature for the years 2004–2011. Journal of Molecular Recognition, 27(6), 297–401. doi: 10.1002/jmr.2347
- Xu, Z., Fang, G., & Wang, S. (2010). Molecularly imprinted solid phase extraction coupled to high-performance liquid chromatography for determination of trace dichlorvos residues in vegetables. Food Chemistry, 119(2), 845–850. doi: 10.1016/j.foodchem.2009.08.047
- Zhang, X., Wang, H., Yang, C., Du, D., & Lin, Y. (2013). Preparation, characterization of Fe3O4 at TiO2 magnetic nanoparticles and their application for immunoassay of biomarker of exposure to organophosphorus pesticides. Biosensors & Bioelectronics, 41(6), 669–674. doi: 10.1016/j.bios.2012.09.047
- Zhao, T., Gao, H., Wang, X., Zhang, L., Qiao, X., & Xu, Z. (2014). Study on a molecularly imprinted solid-phase extraction coupled to capillary electrophoresis method for the determination of trace trichlorfon in vegetables. Food Analytical Methods, 7(6), 1159–1165. doi: 10.1007/s12161-013-9729-y