ABSTRACT
Quinoxaline antibiotics are widely employed in the animal feeding and breeding industry and their metabolites transfer to the environment via the ecological cycle. In this study, fluorescence microspheres-based immunochromatography has been established for the simultaneous detection of five classical quinoxalines in environmental water and animal foodstuffs. Under the optimal conditions, it achieved qualitative and quantitative detection of five quinoxalines within 15 min. The visual limit of detection (LOD) for five quinoxalines in environmental water and animal foodstuffs was 5–15 µg/L and 100–250 µg/kg, respectively. Besides, assisted by a strip reader, the LOD for the quantitative detection of five quinoxalines was 0.09–2.04 µg/kg. The average recoveries for spiked samples ranged from 74.54% to 126.33%, with the coefficients of variation less than 28.21%. Thirty-four samples collected from different areas were detected with the developed immunochromatography and commercial enzyme-linked immunosorbent kits; the results of the two methods showed a good correlation.
Introduction
The animal feeding and breeding industry cannot develop without the application of veterinary drugs. Quinoxalines, heterocyclic compounds containing a benzene ring and a pyrazine ring, can damage and inhibit DNA synthesis of bacteria and exhibit a broad range of biological activities (Cheng et al., Citation2015; English & Dunegan, Citation1970; Liu et al., Citation2016; Vicente et al., Citation2009; Wang, Zhang, et al., Citation2015). They have been known as potent antibacterial agents since the 1940s and widely used to promote growth and improve feed conversion efficiency in animal feeding since the 1970s (Carta, Corona, & Loriga, Citation2005; Cheng et al., Citation2016). Carbadox (CBX), mequindox (MEQ), olaquindox (OLA), quinocetone (QCT), and cyadox (CYA) are the five classical quinoxalines that have been commonly used as medicinal feed additives in animal production (Liu & Sun, Citation2013). However, the abuse of quinoxalines has caused drug residue and drug resistance. Therefore, OLA and CBX, with potential carcinogenic, teratogenic, and mutagenic effects, have been forbidden by the European Union since 1998 (Wang et al., Citation2010). MEQ and QCT, which are the alternatives of OLA and CBX, also have been found to exhibit genotoxicity (Ihsan, Wang, Tu, et al., Citation2013; Ihsan, Wang, Zhang, et al., Citation2013). CYA has been demonstrated to be safe (Hao et al., Citation2013), but the study of Yuan et al. suggested that CYA might present mild toxicity in non-rodents (Wang, Zhou, et al., Citation2015). Thus, it is significant to monitor and control the abuse of quinoxalines, especially the five classical ones.
Many analytical technologies have been developed for detecting quinoxalines and their metabolites, such as chromatography-tandem mass spectrometry (LC-MS/MS) (Sniegocki, Gbylik-Sikorska, Posyniak, & Zmudzki, Citation2014), high-performance liquid chromatography-tandem mass spectrometry (Souza Dibai et al., Citation2015), enzyme-linked immunosorbent assay (ELISA) (Cheng, Shen, Wang, Jiang, & Zhang, Citation2013; Jiang, Beier, Wang, Wu, & Shen, Citation2013; Le et al., Citation2013; Peng et al., Citation2015; Peng et al., Citation2015), fluorescence-linked immunosorbent assay (Le, Zhu, & Yu, Citation2016), time-resolved fluoroimmunoassay (Le, Wei, Niu, & Liu, Citation2014; Le, Yu, & Niu, Citation2015), and lateral flow immunoassay (LFIA) (Le et al., Citation2012; Le, Zhu, Shu, & Zhang, Citation2016; Pei et al., Citation2016; Song et al., Citation2011). Among these technologies, LFIA, which has been widely employed in point-of-care testing, allows rapid, sensitive, stable, convenient, inexpensive, and user-friendly determination of analytes (Tao et al., Citation2014).
Colloidal gold (CG) nanoparticles have been the most commonly used label in LFIA, but recently many novel nanomaterials have been employed as labels to improve the sensitivity of LFIA. Fluorescence microspheres (FMs), which are modified with functional groups, showed stable configuration and high quantum yield (Wang et al., Citation2014; Zhang et al., Citation2016) and have been widely applied in the area of food safety (Chen et al., Citation2013; Liu et al., Citation2015; Zhou et al., Citation2014). Xie et al. (Citation2014) developed and evaluated CG- and FMs-based LFIA for the detection of Escherichia coli O157:H7; the results proved that the sensitivity, consumption of antibodies, and coefficient of variation (CV) of FMs-based LFIA were better than those of CG-based LFIA. CG-based LFIAs have been developed for monitoring the quinoxaline antibiotics, and it has been indicated that the LFIA is a useful screening method for the qualitative, semiquantitative, or quantitative detection of quinoxalines (Le et al., Citation2012; Le, Zhu, Shu, et al., Citation2016; Pei et al., Citation2016; Song et al., Citation2011). However, FMs-based immunochromatography (FMIC) for the determination of quinoxalines has not been reported. Based on our previous study (Pei et al., Citation2016), we adopted FMIC for the simultaneous detection of five classical quinoxalines in environmental water and animal foodstuffs in this study.
Materials and methods
Materials and instruments
FMs with different colors and diameters were purchased from Life Technologies Corporation (USA); OLA standard (>95%) was provided by Beijing WDWK Biotechnology Co. Ltd; monoclonal antibody (mAb) against the five quinoxalines was prepared and stored in our laboratory (Pei et al., Citation2016). Bovine serum albumin (BSA), Tween-20, goat anti-mouse IgG, MEQ, QCT, CBX, and CYA were obtained from Sigma Corporation (St. Louis, USA). Most reagents were stored at 4°C before use.
The Nano Drop ND-1000 spectrophotometer was supplied by Gene Co., Ltd. (Hong Kong, China). The strip reader was provided by QIAGEN (Dusseldorf, Germany). The XYZ-3000 dispensing platform, guillotine cutter (Model: CM 4000) for the preparation of FMIC strips was provided by Bio Dot Inc. (Irvine, CA, USA).
Preparation of FMs and antibody conjugation
The conjugation of FMs and antibody was prepared with the active ester method. Thirty microlitre of carboxyl-modified FMs was dissolved in PB buffer (pH 6.0). Afterwards, 10 µL of 1-(3-Dimethylaminopropyl)-3-ethylcarbodiimide hydrochloride (EDC, 1.0 mg/mL) and N-Hydroxysulfosuccinimide sodium salt (NHSS, 1.0 mg/mL) were added simultaneously, and the mixture was agitated for 15 min to activate the carboxyl groups on the surface of FMs. After the addition of 50 µL of the 100-fold diluted mAb dropwise, the solution was incubated at room temperature for 10 min. And then, 20 µL of BSA solution (20%, w/v) was added for blocking unreacted active sites for 10 min. After centrifugation at 10,000 rpm for 12 min at 4°C, the pellet was resuspended in 200 μL of borate buffer containing 2% Tween-20. The prepared conjugation was stored at 4°C until use.
Preparation of immunochromatographic test strips
The immunochromatographic test strips were assembled using the method described previously with modifications (Tao et al., Citation2014). Both the sample pad and the conjugation pad were made of glass fiber. The nitrocellulose (NC) membrane was prepared by using a BioJet Quanti3000k dispenser; the test line with the edge distance of 0.9 cm was dispensed with antigen. The control line was spotted with the anti-mouse IgG, the spotting density was 0.80 μL/cm, and the interval between test and control lines was 0.60 cm. The prepared NC membranes were dried at 37°C for at least 12 hours. The absorption pad was used without treatment. The sample pad, conjugation pad, NC membrane, and absorption pad were attached on a Poly Vinyl Chloride (PVC) backing card overlapping each other by 2 mm, and then cut into strips (0.39 cm × 6.0 cm) by the automatic programmable cutter. All strips were packaged with plastic bags containing desiccant gel and stored until use.
FMIC test procedure
As illustrated in , the detection principle is similar to that in our previous report (Pei et al., Citation2016). The prepared FMs-mAb probes were incubated with the sample in the microwell plate, and then the FMIC test strips were inserted vertically into the well. After 10 min, results were obtained by an ultraviolet lamp or a portable strip reader.
Optimization of the parameters for FMs-mAb probes preparation
FMs of three different colors (red, green, and blue) were first evaluated in the study. The buffer system is an important parameter for the preparation of FMs-mAb probes. PB buffer, Carbonate Buffer (CB), and Morpholine ethanesulfonic acid (MES) buffer were selected. Then, in order to evaluate the influence of BSA in the process of labeling, ultrapure water with different concentrations of BSA (0, 1%, 5%, and 10%, w/v) was used to dilute the antibody 100 times. In addition, the amount of mAb used in the labeling process (10, 30, 50, and 80 µL of 100-fold diluted antibody) was optimized to obtain a high coupling rate of FMs-mAb probes. Each experiment was performed with three replications.
Optimization of the reaction conditions for quinoxalines detection
The FMIC was developed by optimizing the following parameters. Three kinds of sample pads (filter blood membrane (FBM), RB65 glass fiber, and SB08 glass fiber), incubation time of sample and FMs-mAb probes (1, 2, 3, 4, and 5 min), volume of FMs-mAb probes (1, 3, 5, and 7 µL/well), and detection time were optimized. Each experiment was performed with three replications.
Sample pretreatment
The samples (environmental water and animal foodstuffs) were first ascertained to be free of quinoxalines by LC-MS/MS. In order to reduce the matrix effect, samples collected from different areas were used after pretreatment. The environmental water was centrifuged at 8000 r/min for 5 min, and the supernatant was used for detection. As for the animal foodstuffs, the extraction mode was optimized as reported in our previous publication (Pei et al., Citation2016). Briefly, the collected animal foodstuffs were pulverized; 1 g ± 0.1 g of the power feed was extracted with 3 mL of methanol–water (5:95, v/v). After standing for 10 min, 1 mL of the extraction collected from the supernatant was diluted with 2 mL of PBS buffer (containing 0.05% Tween-20 and 0.1% K2CO3) before assaying.
Results and discussions
Parameters for the preparation of FMs-mAb probes
In the white background of the NC membranes, the color of labels is an objective factor for qualitative detection by naked eyes. The mAbs against the five quinoxalines were first conjugated with FMs via the EDC/NHSS-mediated method, and then the conjugations were applied on the prepared FMIC test strips. As shown in (A), compared with the green and blue FMs, the red FMs exhibited apparent color on the NC membrane and the inhibition rate of OLA (5.0 ng/mL) was higher than that of the other FMs. Therefore, the red FMs were chosen to provide visual signals. The diameter of FMs is also important for the sensitivity of FMIC as it is easier to observe red FMs with large size on the NC membrane by naked eyes (as shown in (B)). It is possible that the large diameter of FMs exhibited stronger fluorescence intensity than that of small-sized FMs. Thus, 200 nm of the red FMs were employed in subsequent experiments of this study.
Figure 2. The influence of color and diameter of FMs in the development of FMIC. (A) Visual signals of red (580/605), green (505/515), and blue (365/415) FMs on the FMIC under the ultraviolet lamp. (B) Visual signals of red (580/605) FMs with different diameters on the FMIC under the ultraviolet lamp, and the inset photograph is the FMs with different diameters in visible light.
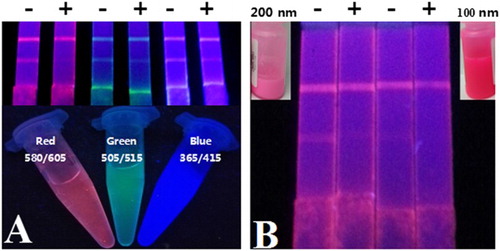
The usage of antibody has as a key role in the preparation of FMs-mAb probes, and it is also an important parameter for the sensitivity of the developed FMIC. The mAb was first diluted 100 times and then added into the buffer system at different volumes. As shown in (A), the fluorescence intensity of negative sample increased with the increase in the mAb amount. However, the excess mAb can not only bind with the targets, but can also be captured by the antigen immobilized on the test line, which causes the decrease in sensitivity. Therefore, 50 µL of 100-fold diluted antibody was chosen. MES buffer, CB buffer, and PB buffer were used as the buffer system for the preparation of FMs-mAb probes. And the FMs-mAb probes prepared in the PB buffer system performed the best; the fluorescence intensity of negative sample was the strongest; and the inhibition ratio of 5 ng/mL was the highest (see (B)). Because the volume of BSA in antibody dilution may reduce the nonspecific binding, resulting in the improvement of sensitivity, the concentration of BSA was adjusted. As shown in (C), the mAb diluted with 1% BSA (w/v) performed the best, as excess BSA may compete with the mAb for the binding site of FMs, which led to a significant decrease in fluorescence intensity.
Optimization of the FMIC for quinoxalines detection
The sample pad of the FMIC test strip may affect the release of FMs-mAb probes, which is important for the assay sensitivity, specificity, and consistency. Among the three types of sample pads (FBM, RB65 glass fiber, and SB08 glass fiber), FBM was the most suitable one (see (A)). The incubation time of FMs-mAb probes and sample is related to the sensitivity of FMIC. As shown in (B), the inhibition ratio of 5 ng/mL improved with the increase in incubation time. The target in the sample could react with the FMs-mAb probes more completely within the long incubation time, generating a high inhibition ratio. As for the analysis time, 3 min was the optimal. The amount of FMs-mAb probes was another parameter optimized. The fluorescence intensity of the negative sample might be very weak with the small volume of FMs-mAb probes, which might cause false-positive results. However, a significant background signal might be generated with the excess FMs-mAb probes, resulting in the decrease in FMIC sensitivity. The results shown in (C) indicated that 5 µL/well of FMs-mAb probes was optimal. The fluorescence intensity of the FMIC test strip increased with the increase in detection time, but after 10 min, the fluorescence intensity changed insignificantly. Therefore, with the addition of the 3-min incubation in the ELISA well, the total time of the LFIA system for quinoxalines detection was 13 min.
Sensitivity and specificity of FMIC
The samples spiked with serial concentrations of quinoxalines were pretreated as described above. The concentrations of OLA, CBX, MEQ, QCT, and CYA spiked into the environmental water were 0, 1, 2.5, 5, 10, 15, 20, and 25 µg/L, and the amounts of quinoxalines fortified in the animal foodstuffs were 0, 5, 10, 25, 50, 100, 150, 200, 250, 300, 350, 400 µg/kg. The test lines showed no fluorescence when the concentrations of OLA, CBX, MEQ, QCT, and CYA in the environmental water were 5, 15, 10, 5, and 10 µg/L, respectively (see Figure 1S). And when OLA, CBX, MEQ, QCT, and CYA in the animal foodstuffs were 100, 250, 250, 150, and 200 µg/kg, respectively, the test lines cannot be identified by naked eyes (see Figure 2S). This indicated that the developed FMIC exhibited high cross-reactivity (27.55–109.0%) against CBX, MEQ, QCT, and CYA. And this significantly suggested that the developed FMIC was able to simultaneously detect five quinoxaline residues in the samples. In addition, the fluorescence signals of the test lines were obtained by the strip reader. The analyte concentrations and the fluorescence intensity ratio (F/F0, where F and F0 are defined as the fluorescence intensity of positive and negative samples, respectively) were fitted to sigmoid curves for the determination of the five quinoxaline residues (see ), and the IC50 values and cross-reactivity were obtained from the standard curves (see Table 1S). The cross-reactivity was calculated by the IC50 ratio between the other four quinoxalines and OLA. The limits of detection for the determination of the five quinoxalines were 0.10, 0.27, 0.25, 0.09, and 0.10 µg/L in the environmental water and 0.90, 2.04, 1.12, 0.88, and 1.56 µg/kg in animal foodstuffs. This manifested that the developed FMIC can qualitatively and quantitatively detect the five quinoxalines in the environmental water and animal foodstuffs.
Analysis of samples
To evaluate the determination capability of the developed FMIC, different concentrations of the five quinoxalines were spiked in the environmental water and animal foodstuffs. The spiked samples were detected after pretreatment, and the results shown in indicated that the recoveries ranged from 83.49% to 108.27% with the CV less than 28.21%. According to the results, the recoveries of quinoxalines in the animal foodstuffs were lower than that in the water samples. It implied that as the animal foodstuffs were extracted, the amount of analytes in the extraction might be less than that in the water samples. And the influence of the matrix effect on the extraction of animal foodstuffs was more significant than that of the environment water. Thirty-four samples (17 environmental water samples and 17 animal foodstuff samples) were collected from different areas in Beijing. The real samples were simultaneously analyzed with the developed FMIC and commercial ELISA kits. The results obtained from the FMIC were quite consistent with those from the ELISA kits, with a coincidence rate of 92.31%. This implied that the developed FMIC can be applied to the rapid screening of the five quinoxaline residues in real samples.
Table 1. The recovery of the FMIC system for the detection of the quinoxalines in water and feeds samples (n = 3).
Comparison with colloidal gold-based immunochromatography technology (CGIC)
CGIC had been successfully developed for the simultaneous detection of five quinoxalines in animal feed as reported by Le et al. (Citation2015). According to the results (see Table 2S), the detection time of the FMIC is longer than that of CGIC, but the FMIC exhibited advantages over CGIC in terms of sensitivity. In addition, as affected by the factors of light and fluorescence lifetime, the FMIC showed larger CV value than GCIC.
Conclusion
A FMIC was established for the rapid and simultaneous detection of OLA, CBX, MEQ, QCT, and CYA in the environmental water and animal foodstuffs for the first time. The detection could be completed within 15 min, and it exhibited advantages over CGIC in terms of sensitivity. The results of 34 real samples indicated that the accuracy of the FMIC was acceptable. In conclusion, the developed FMIC provided a convenient and feasible approach for the rapid and simultaneous screening of five classical quinoxaline residues in real samples.
Acknowledgements
The authors appreciate the cooperation of other faculty members in the Department of Pharmacology and Toxicology of the College of Veterinary Medicine at China Agricultural University.
Disclosure statement
No potential conflict of interest was reported by the authors.
Notes on contributors
Tao Peng was born in Jiangxi Province and began to study as a Ph.D. student in the College of Veterinary Medicine of China Agricultural University in 2015. His research interests are immunoassay's development for veterinary drug residue.
Xingyao Pei was born in Xinjiang Province and obtained master degree in the College of Veterinary Medicine of China Agricultural University in 2016. Her research interests are immunoassay's development for veterinary drug residue.
Yongjun Zheng obtained his Ph.D. from China Agricultural University and began to work as a faculty in College of Engineering of China Agricultural University. He is currently a full professor in Mechanical and Electrical Engineering. His research interests are biosensor development.
Jianyi Wang was born in Jiangsu Province and began to study as a Ph.D. student in the College of Veterinary Medicine of China Agricultural University in 2015. His research interests are monoclonal antibody development and applications.
Qi Wang was born in Hebei Province and obtained master degree in the College of Veterinary Medicine of China Agricultural University in 2016. Her research interests are immunoassay's development for veterinary drug residue.
Jiancheng Li obtained his Ph.D. from China Agricultural University and began to work as a faculty in College of Veterinary Medicine of China Agricultural University. He is currently an assistant professor in basic veterinary science. His research interests are veterinary pharmacology and toxicology.
Xi Xia obtained his Ph.D. from China Agricultural University and began to work as a faculty in College of Veterinary Medicine of China Agricultural University. He is currently an assistant professor in basic veterinary science.His research interests are veterinary residue analysis and microbial drug resistance.
Haiyang Jiang was born in Sichuan Province, he obtained Ph.D. from China Agricultural University and began to work as a faculty in College of Veterinary Medicine of China Agricultural University. He is currently an assistant professor in basic veterinary science. His research interests are food safety and veterinary pharmacology and toxicology.
Additional information
Funding
References
- Carta, A., Corona, P., & Loriga, M. (2005). Quinoxaline 1,4-dioxide: A versatile scaffold endowed with manifold activities. Current Medicinal Chemistry, 12(19), 2259–2272. doi: 10.2174/0929867054864831
- Cheng, G., Li, B., Wang, C., Zhang, H., Liang, G., Weng, Z., … Yuan, Z. (2015). Systematic and molecular basis of the antibacterial action of quinoxaline 1,4-Di-N-oxides against Escherichia coli. PLoS One, 10(8), e136450. doi: 10.1371/journal.pone.0136450
- Cheng, G., Sa, W., Cao, C., Guo, L., Hao, H., Liu, Z., & Yuan, Z. (2016). Quinoxaline 1,4-di-N-oxides: Biological activities and mechanisms of actions. Frontiers In Pharmacology, 7, 1–21. doi: 10.3389/fphar.2016.00064.
- Cheng, L., Shen, J., Wang, Z., Jiang, W., & Zhang, S. (2013). A sensitive and specific ELISA for determining a residue marker of three quinoxaline antibiotics in swine liver. Analytical and Bioanalytical Chemistry, 405(8), 2653–2659. doi: 10.1007/s00216-012-6696-x
- Chen, R., Li, H., Zhang, H., Zhang, S., Shi, W., Shen, J., & Wang, Z. (2013). Development of a lateral flow fluorescent microsphere immunoassay for the determination of sulfamethazine in milk. Analytical and Bioanalytical Chemistry, 405(21), 6783–6789. doi: 10.1007/s00216-013-7150-4
- English, A. R., & Dunegan, C. M. (1970). Quinoxaline-1, 4-di-N oxides I. Inhibition of deoxyribonucleic Acid synthesis in Escherichia coli by 2,3-dihydroxymethyl-quinoxaline-1, 4-di-N-oxide. Experimental Biology and Medicine, 133(2), 398–400. doi: 10.3181/00379727-133-34481
- Hao, H., Guo, W., Iqbal, Z., Cheng, G., Wang, X., Dai, M., … Yuan, Z. (2013). Impact of cyadox on human colonic microflora in chemostat models. Regulatory Toxicology and Pharmacology, 67(3), 335–343. doi: 10.1016/j.yrtph.2013.08.011
- Ihsan, A., Wang, X., Tu, H., Zhang, W., Dai, M., Peng, D., … Yuan, Z. (2013). Genotoxicity evaluation of Mequindox in different short-term tests. Food and Chemical Toxicology, 51, 330–336. doi: 10.1016/j.fct.2012.10.003
- Ihsan, A., Wang, X., Zhang, W., Tu, H., Wang, Y., Huang, L., … Yuan, Z. (2013). Genotoxicity of quinocetone, cyadox and olaquindox in vitro and in vivo. Food and Chemical Toxicology, 59, 207–214. doi: 10.1016/j.fct.2013.06.008
- Jiang, W., Beier, R. C., Wang, Z., Wu, Y., & Shen, J. (2013). Simultaneous screening analysis of 3-methyl-quinoxaline-2-carboxylic Acid and quinoxaline-2-carboxylic Acid residues in edible animal tissues by a competitive indirect immunoassay. Journal of Agricultural and Food Chemistry, 61(42), 10018–10025. doi: 10.1021/jf4037497
- Le, T., Wei, S., Niu, X., & Liu, J. (2014). Development of a time-resolved fluoroimmunoassay for the rapid detection of methyl-3-quinoxaline-2-carboxylic Acid in porcine tissues. Analytical Letters, 47(4), 606–615. doi: 10.1080/00032719.2013.845897
- Le, T., Xu, J., He, H., Niu, X., Chen, Y., & Jia, Y. (2013). Development and validation of an enzyme-linked immunosorbent assay for rapid detection of multi-residues of five quinoxaline-1,4-dioxides in animal feeds. Food and Agricultural Immunology, 24(4), 457–466. doi: 10.1080/09540105.2012.716024
- Le, T., Xu, J., Jia, Y. Y., He, H. Q., Niu, X. D., & Chen, Y. (2012). Development and validation of an immunochromatographic assay for the rapid detection of quinoxaline-2-carboxylic acid, the major metabolite of carbadox in the edible tissues of pigs. Food Addit Contam Part A Chem Anal Control Expo Risk Assess, 29(6), 925–934. doi: 10.1080/19440049.2012.662703
- Le, T., Yu, H., & Niu, X. (2015). Detecting quinoxaline-2-carboxylic acid in animal tissues by using sensitive rapid enzyme-linked immunosorbent assay and time-resolved fluoroimmunoassay. Food Chemistry, 175, 85–91. doi: 10.1016/j.foodchem.2014.11.135
- Le, T., Zhu, L., Shu, L., & Zhang, L. (2016). Simultaneous determination of five quinoxaline-1,4-dioxides in animal feeds using an immunochromatographic strip. Food Additives & Contaminants Part A, 33(2), 244–251.
- Le, T., Zhu, L., & Yu, H. (2016). Dual-label quantum dot-based immunoassay for simultaneous determination of carbadox and olaquindox metabolites in animal tissues. Food Chemistry, 199, 70–74. doi: 10.1016/j.foodchem.2015.11.116
- Liu, D., Huang, Y., Chen, M., Wang, S., Liu, K., & Lai, W. (2015). Rapid detection method for aflatoxin B1 in soybean sauce based on fluorescent microspheres probe. Food Control, 50, 659–662. doi: 10.1016/j.foodcont.2014.10.011
- Liu, Z., & Sun, Z. (2013). The metabolism of carbadox, olaquindox, mequindox, quinocetone and cyadox: An overview. Medicinal Chemistry, 9(8), 1017–1027. doi: 10.2174/1573406411309080002
- Liu, Q., Zhang, J., Luo, X., Ihsan, A., Liu, X., Dai, M., … Yuan, Z. (2016). Further investigations into the genotoxicity of quinoxaline-di-N-oxides and their primary metabolites. Food and Chemical Toxicology, 93, 145–157. doi: 10.1016/j.fct.2016.04.029
- Pei, X., Wang, Q., Li, X., Xie, J., Xie, S., Peng, T., … Jiang, H. (2016). Provision of ultrasensitive quantitative Gold immunochromatography for rapid monitoring of olaquindox in animal feed and Water samples. Food Analytical Methods, 9(7), 1919–1927. doi: 10.1007/s12161-015-0360-y
- Peng, J., Kong, D., Liu, L., Song, S., Kuang, H., & Xu, C. (2015). Determination of quinoxaline antibiotics in fish feed by enzyme-linked immunosorbent assay using a monoclonal antibody. Analytical Methods, 7(12), 5204–5209. doi: 10.1039/C5AY00953G
- Sniegocki, T., Gbylik-Sikorska, M., Posyniak, A., & Zmudzki, J. (2014). Determination of carbadox and olaquindox metabolites in swine muscle by liquid chromatography/mass spectrometry. Journal of Chromatography B, 944, 25–29. doi: 10.1016/j.jchromb.2013.09.039
- Song, C., Liu, Q., Zhi, A., Yang, J., Zhi, Y., Li, Q., … Zhang, G. (2011). Development of a lateral flow colloidal gold immunoassay strip for the rapid detection of olaquindox residues. Journal of Agricultural and Food Chemistry, 59(17), 9319–9326. doi: 10.1021/jf202213m
- Souza Dibai, W. L., de Alkimin Filho, J. F., Da Silva Oliveira, F. A., Sampaio De Assis, D. C., Camargos Lara, L. J., de Figueiredo, T. C., & de Vasconcelos Cançado, S. (2015). HPLC-MS/MS method validation for the detection of carbadox and olaquindox in poultry and swine feedingstuffs. Talanta, 144, 740–744. doi: 10.1016/j.talanta.2015.07.029
- Tao, P., Zhang, F. S., Yang, W. C., Li, D. X., Chen, Y., Xiong, Y. H., … Lai, W. H. (2014). Lateral-flow assay for rapid quantitative detection of clorprenaline residue in swine urine. Journal of Food Protection, 77(10), 1824–1829. doi: 10.4315/0362-028X.JFP-14-103
- Vicente, E., Pérez-Silanes, S., Lima, L. M., Ancizu, S., Burguete, A., Solano, B., … Monge, A. (2009). Selective activity against mycobacterium tuberculosis of new quinoxaline 1,4-di-N-oxides. Bioorganic & Medicinal Chemistry, 17(1), 385–389. doi: 10.1016/j.bmc.2008.10.086
- Wang, Z., Li, H., Li, C., Yu, Q., Shen, J., & De Saeger, S. (2014). Development and application of a quantitative fluorescence-based immunochromatographic assay for fumonisin B1 in maize. Journal of Agricultural and Food Chemistry, 62(27), 6294–6298. doi: 10.1021/jf5017219
- Wang, X., Zhang, H., Huang, L., Pan, Y., Li, J., Chen, D., … Yuan, Z. (2015). Deoxidation rates play a critical role in DNA damage mediated by important synthetic drugs, quinoxaline 1,4-dioxides. Chemical Research in Toxicology, 28(3), 470–481. doi: 10.1021/tx5004326
- Wang, X., Zhang, W., Wang, Y., Peng, D., Ihsan, A., Huang, X., … Yuan, Z. (2010). Acute and sub-chronic oral toxicological evaluations of quinocetone in wistar rats. Regulatory Toxicology and Pharmacology, 58(3), 421–427. doi: 10.1016/j.yrtph.2010.08.008
- Wang, X., Zhou, W., Ihsan, A., Chen, D., Cheng, G., Hao, H., … Yuan, Z. (2015). Assessment of thirteen-week subchronic oral toxicity of cyadox in beagle dogs. Regulatory Toxicology and Pharmacology, 73(2), 652–659. doi: 10.1016/j.yrtph.2015.09.023
- Xie, Q., Wu, Y., Xiong, Q., Xu, H., Xiong, Y., Liu, K., … Lai, W. (2014). Advantages of fluorescent microspheres compared with colloidal gold as a label in immunochromatographic lateral flow assays. Biosensors and Bioelectronics, 54, 262–265. doi: 10.1016/j.bios.2013.11.002
- Zhang, X., Wen, K., Wang, Z., Jiang, H., Beier, R. C., & Shen, J. (2016). An ultra-sensitive monoclonal antibody-based fluorescent microsphere immunochromatographic test strip assay for detecting aflatoxin M 1 in milk. Food Control, 60, 588–595. doi: 10.1016/j.foodcont.2015.08.040
- Zhou, J., Zhu, K., Xu, F., Wang, W., Jiang, H., Wang, Z., & Ding, S. (2014). Development of a microsphere-based fluorescence immunochromatographic assay for monitoring lincomycin in milk, honey, beef, and swine urine. Journal of Agricultural and Food Chemistry, 62(49), 12061–12066. doi: 10.1021/jf5029416