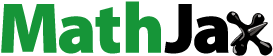
ABSTRACT
The herbicide propisochlor can be introduced into the water cycle via agricultural activities, thus possibly leading to harmful ecological effects. It is significant for the development of a rapid method for propisochlor analysis in water. In this work, a rapid homogeneous immunoassay based on fluorescence polarization immunoassay (FPIA) was firstly developed for the determination of propisochlor in pond water. The developed FPIA assay can be completed within only 14 min; the 50% inhibition concentration (IC50) was 125.1 ng/mL and the limit of detection was 8.4 ng/mL. The recoveries of propisochlor in pond water ranged from 80.0% to 86.6%. A high performance liquid chromatography confirmation showed a good correlation with the developed FPIA (R2 = 0.9748). The developed FPIA exhibited satisfactory rapidity, high sensitivity and excellent specificity, meeting the standards of the screening assay of propisochlor in pond water on site. The molecular modeling showed that the alkoxyalkyl of chloroacetamide herbicides exerted important effects on the herbicide–antibody binding.
Highlights
A homogeneous FPIA was developed for the determination of propisochlor for the first time.
The developed FPIA exhibited satisfactory rapidity, high sensitivity and high sample throughput.
The molecular structure of chloroacetamide herbicides was analyzed by molecular modeling.
The recognition mechanism between the herbicides and antibody was also studied.
Introduction
Herbicides have been widely used in agricultural production activities all over the world (Brender & Weyer, Citation2016; Choi, Kim, Choi, Eremin, & Chun, Citation2011). However, the intensive use of herbicides has resulted in serious pollution of agricultural products, soil resources, especially water resources, which pollute the whole ecological system, and possibly leads to a serious threat to the safety of the water resources (Brender & Weyer, Citation2016; Cruzeiro, Rocha, Pardal, & Rocha, Citation2016; Lei et al., Citation2011). In recent years, more and more attention has been paid to herbicide residue in water samples worldwide (Feng, Yu, Liu, Liu, & Sun, Citation2015; Lu, Peterson, Gooding, & Lee, Citation2012; Oberleitner et al., Citation2014; Wu et al., Citation2013; Zhang et al., Citation2011). These residual herbicides in water samples also presented a high risk to human health (Barbosa, Solano Mde, & Umbuzeiro Gde, Citation2015; Benito-Peña, Moreno-Bondi, Orellana, Maquieira, & van Amerongen, Citation2005; Kolosova et al., Citation2017; Sekhotha, Monyeki, & Sibuyi, Citation2016; Zhang et al., Citation2011). Therefore, it is necessary to strictly monitor agrochemical residues in water samples.
Propisochlor (2-chloro-N-(isopropyl-ethyl)-N-(2-ethylmethyl-6-methyl) phenyl acetamide) is a chloroacetamide herbicide widely used to extirpate broadleaf weeds. The previous report showed that chloroacetamide herbicides can induce hemolysis, lipid peroxidation and catalase activity (Meire et al., Citation2016). The European Union set the relevant laws and regulations to fix the maximum residue limit (MRL) of propisochlor pesticide in soybean (MRL, 0.1 mg/kg), and single pesticide in drinking water (MRL, 0.1 mg/kg) (Bach et al., Citation2016). The previous research showed that propisochlor could flow into water systems and become highly toxic to some aquatic organisms (Bernasinska, Duchnowicz, Koter-Michalak, & Koceva-Chyla, Citation2013; Rauch & Földényi, Citation2012; Sturve, Scarlet, Halling, Kreuger, & Macia, Citation2016; Tufi et al., Citation2016).
The detection methods of propisochlor were mainly based on chromatography, such as gas chromatography (Mekonen et al., Citation2016), high performance liquid chromatography (HPLC) coupled with mass spectrometry (Baris et al., Citation2016), ultra-performance liquid chromatography with tandem mass spectrometry (UPLC–MS/MS) was also developed for the determination of propisochlor (Wang, Zhang, Nesterenko, Eremin, & Shen, Citation2007). Although instrumental analytic methods could be used for the determination of propisochlor with appropriate limit of detection (LOD) and sensitivity, these instrumental methods are complex in sample preparation and expensive, which restricted their wide application. Thus, it is necessary to establish a simplicity, inexpensive and high sample throughput analysis for propisochlor.
Immunoassays, based on interaction between antigen and antibody, are widely used to monitor pollutant in environment and food, because they are relatively inexpensive, highly rapid and highly sensitive. Fluorescence polarization immunoassay (FPIA) is one of the promising homogeneous immunoassay techniques. The labeled analyte was not necessary to be separated after the antibody–analyte binging reaction. FPIA was applied to determine pesticides (Stevanovic et al., Citation2017) and mycotoxins (Zhang et al., Citation2017). It meets the requirement of simple, cost-effective, fast and high sample throughput. In the present study, the aim was focused on the development and optimization of FPIA for the determination of propisochlor in pond water samples with high sensitivity, high accuracy and high sample throughput. The developed FPIA was confirmed by HPLC, and the results showed that the developed FPIA could be used as a screening analysis for propisochlor in pond water.
Materials and methods
Reagents and instrumentation
Propisochlor, fluorescein isothiocyanate isomer І, N-hydroxysuccinimide (NHS), ethylenediamine (EDF), hexamethylenediamine (HDF), 3-mercaptopropionic acid (3-MPA), Bull serum albumin (BSA) and ovalbumin (OVA) were purchased from Sigma (St Louis, MO, USA). S-(-)-metolachlor (96%), napropamide (97%), metsulfuron-methyl (95%), metalaxyl (96%), propanil (96%), pretilachlor (98%), mefenacet (96%), diflufenican (96%), benzoylprop ethyl (98%), carbetamide (96%), diphenamid (96%), butachlor (96%), acetochlor (94%), atrazine (96%), 2-chloro-2,6-diethyl acetanilide (96%), 2-ethyl-6-toluidine (96%), alachlor (98%) were purchased from J&K SCIENTIFIC LTD (Beijing, China). Other chemicals and organic solvents used in this experiment were of analytical grade or better and were obtained from a local chemical supplier (Yunhui Trade Co., Ltd., Guangzhou, China). The antibody was previously prepared in our laboratory, immunized by propisochlor–MPA–BSA. Pond water samples were collected from the campus of South China Agriculture University (Guangzhou, China). Stock solutions (1 mg/mL) of propisochlor and other structural analogues were prepared with phosphate buffer saline-Tween (PBST buffer, contained 10% methanol), and then stored at 4°C.
FPIA was performed employing an FP-Fluorescin 480/535 (Wallac VICTOR3™ 1420 multilable counter, PerkinElmer, Waltham, MA, USA) in default mode. The HPLC confirmation was performed by a Shimadzu LC-20A series HPLC system equipped with an LC-20AT intelligent gradient pump, a SPD-20A ultraviolet–visible (UV−VIS) light absorbance detector, a CTO-10AS vp temperature-controlled column compartment, an SIL-20A automatic sampler and a CMB-20A data collector (Shimadzu, Kyoto, Japan).
Synthesis of hapten
Propisochlor-3-mercaptopropionic acid (PMPA) was synthesized from propisochlor and 3-MPA in a similar way as described in the previous studies (Bach et al., Citation2016; Konda & Pásztor, Citation2001). Firstly, 100 μL 3-MPA and 0.2 g NaOH were dissolved in 15 mL ethanol. Then, the mixture was added to a flask with 150 μL propisochlor drop by drop, and refluxed for 12 h in an 80° oil bath pan. The reaction mixture was filtered from KCl and evaporated under vacuum. After dissolving in 10 mL NaHCO3 (5%, w/v) and acidifying to pH 3.0 with 6 M HCl, the crude product was extracted three times by 5 mL of ethylacetate. Then, the organic phase was washed with water and dried with Na2SO4. The BMPA was purified by preparative thin layer chromatography (TLC) using a mixture of benzene:1,4-dioxane:acetic acid (26:2:1, v/v/v) as an eluent. And the pure product (Rf = 0.5) was extracted by the mix eluent and evaporated. The resultant light yellow oily product was PMPA hapten.
Synthesis of antigen and production of antibody
The PMPA hapten was conjugated with BSA and OVA using EDC. Firstly, 2 mg of PMPA hapten was dissolved in 200 μL N,N-dimethylformamide (DMF), and 10 mg BSA or OVA was dissolved in 2.8 mL 9% NaCl. Then, the DMF solution was gradually added into the 9% NaCl solution, and 2 mg EDC was added. The reaction was allowed to take place until muddy under stirring at room temperature (RT). The mixture was transferred into a dialysis tube and extensively dialyzed against 9% NaCl for 72 h at 4°. The dialysis solution was changed very 8 h. The obtained PMPA hapten conjugation to BSA or OVA was determined by UV spectrophotometry. Finally, working aliquots of the conjugations (1 mg/mL in 9% NaCl) were stored at −20°C. PMPA–BSA was used as antigen and PMPA–OVA was used as coating antigen for detecting the titer of the antiserum.
Two female New Zealand white rabbits (weighting 2 kg) were immunized with PMPA–BSA. The priming injection was carried out intradermally with 0.5 mg of PMPA–BSA and emulsified with Freund’s complete adjuvant (1:1, v/v). Then, the rabbits received booster injection intradermally with 0.5 mg of PMPA–BSA and emulsified with Freund’s incomplete adjuvant (1:1, v/v) every 4 weeks. Animals were bled through the ear vein 1 week after the third injection, and the antiserum titers were detected by enzyme-linked immunosorbent assay (ELISA). After the fourth booster injection, the rabbits were bled through the ear vein. The blood was allowed to coagulate for 1 h at 37°C, centrifuged, purified by caprylic acid-saturated ammonium sulfate precipitation, then the purified antibody was divided into aliquots and stored at −20°C until use.
Synthesis of fluorescein-labeled tracers
The fluorescein was synthesized by isothiocyanate and EDF or HDF according to the previous study () (Bach et al., Citation2016). Briefly, 141.1 mg (40 μmol) propisochlor–MPA, 15.3 mg (80 μmol) EDC and 9.7 mg (80 μmol) NHS were dissolved in 500 μL DMF, and stirred at RT overnight. Then the reaction mixtures were centrifuged to remove the precipitation. The obtained intermediate products were stirred at RT overnight in the dark with 4.5 mg (10 μmol) EDF or 5.05 mg (10 μmol) HDF. The mixtures were separated by TLC, and trichloromethane:methanol (4:1, v/v) was used as the eluent. The major yellow bands were collected, eluted with 1 mL methanol and verified by electrospray ionization-mass spectrum (ESI–MS). After detecting the activities, the tracers were stored at −20°C in the dark.
The absorbance of antibody-EDF/HDF, was determined by Wallac VICTOR3 1420 Multi Lable counter, and then the concentrations were calculated employing the following equation (Echeverry, Zapata, Paez, Mendez, & Pena, Citation2015):
(1)
(1) where A, the absorbance of antibody-EDF/HDF, was detected under 492 nm. ε, molar extinction coefficient, 8.78 × 104 M−1 cm−1; L, the width of the colorimetric cup (cm); Ctracer, the different concentrations of tracers.
FPIA procedure
Ninety microliters of tracer and 20 μL different concentrations of propisochlor standard (diluted with BBT buffer) were added to the fluorescent micro-porous plate. After short slight vibration blending, 90 μL polyclonal antibodies were added to each microplate. Then the FP values were collected after incubation. A four-parameter logistic equation was used to fit the standard curves according to Equation (2) (Howe, Reichelt-Brushett, Clark, & Seery, Citation2017; Yakovleva, Zherdev, Popova, Eremin, & Dzantiev, Citation2003)
(2)
(2) where A and D were the maximal and minimal signals of the assay (Amax and Amin), respectively. B denotes the slope of the function. C is the concentration of propisochlor resulting in 50% inhibition of binding of the enzyme tracer to the antibody (IC50). y was the collected FP values and x was the logarithmic value of the different concentrations of propisochlor. The IC10 value was defined as the LOD, and the IC20–IC80 was set as the dynamic working range (Bach et al., Citation2016).
Optimization of FPIA
To improve performance of the FPIA, the physicochemical parameters were optimized, such as reaction kinetics, antibody dilution, tracers’ concentration, and working buffer (pH and ionic strength), methanol concentration.
The optimum working condition was selected according to three parameters (IC50, ΔmP(FPmax − FPmin), ΔmP/IC50) (Kissane & Shephard, Citation2017). The lower IC50 values and higher ΔmP and higher ratio of ΔmP/IC50 manifest a more sensitive assay.
Specificity
The specificity of the FPIA was evaluated by cross-reactivity (CR), which was determined using several kinds of structural analogues.
(3)
(3)
Molecular modeling
Minimum energy conformations of chloroacetamide herbicides were calculated using the Minimize module of Sybyl-X 2.1.1 (Tripos Inc., USA). The force field was Tripos with an 8 Å cutoff for non-bonded interactions, and the atomic point charges were calculated with Gasteiger–Huckel. Minimizations were achieved using the steepest descent method for the first 1000 steps, followed by the Powell calculation method until the root-mean-square of the gradient became less than 0.005 kcal/(mol Å). Molecular surfaces were calculated in the MOLCAD Surface module of Sybyl-X 2.1.1 with the default setting. Map type was based on “electrostatic potential”, and the color mode was texture.
Pretreatment and recovery of water sample
Water samples for FPIA analysis were only simply filtered (0.45 μm), following dilution with BBT, and then analyzed by the proposed FPIA. Spiked samples were fortified with propisochlor at three levels (50, 100, 150 ng/mL) before the filtration, and then were analyzed by FPIA for the recovery evaluation.
HPLC analysis
HPLC analysis was performed using a Waters E2695 HPLC instrument with a UV detector (model 2475). A C18 column (150 × 2.1 mm, 5 μm particle size, Agilent) was used as the stationary phase. The flow rate was 1.0 mL/min and the column temperature was 28°C. The measured results were compared with the results of FPIA. The linear correlation between the results of the two methods was verified by Student’s t-test.
Water samples for HPLC analysis were extracted in triplicate by ethyl acetate, and then the combined ethyl acetate was concentrated by rotate vacuum evaporation; the residue was re-dissolved in n-hexane for HPLC analysis.
Results and discussion
Synthesis of hapten, antigens and characterization of antibody
For obtaining a high affinity and specificity antibody against a small molecular weight pollutant, hapten design is a critical step. 3-MPA was previously used and added to acetochlor and butachlor as a spacer arm (Bach et al., Citation2016; Yakovleva et al, Citation2002). The resulting haptens have carboxyl for conjugating with carrier proteins, and the obtained antibody had high specificity. Therefore, in the present study, 3-MPA derivative of propisochlor (PMPA) was synthesized as hapten. The purified hapten was identified by ESI–MS. The mass peaks of 354 showed that PMPA was successfully synthesized (Figure S1).
PMPA was conjugated with BSA and OVA. The UV absorbance properties of PMPA, BSA and the conjugations were determined. The UV absorbance spectra are shown in Figure S2. The UV spectrometry data indicated that PMPA–BSA was successfully conjugated. Then, PMPA–BSA was used as antigen to immunize two rabbits. After the third injection, the obtained serum was tested by ELISA. As shown in Figure S3, the serum of rabbit 2 has higher titer than that of rabbit 1.
Synthesis of fluorescein-labeled tracers
The carboxyl group in propisochlor–MPA can directly react with the amino group in EDF or HDF. The tracers were isolated from the reaction mixtures by preparative TLC (chloroform:methanol = 4:1). The bands were yellow fluorescence and the Rf were 0.2 and 0.3, respectively. The target bands were collected and extracted with methanol (1 mL), then identified by ESI–MS. The mass peaks of m/z = 783.2 and 839.2 showed that propisochlor–MPA–EDF (tracer A) and propisochlor–MPA–HDF (tracer B) were successfully formed. Generally, the FP value of the free tracer was smaller, and it would rise when specifically combining with antibody. In this investigation, the antibody was employed to check the activities of the obtained tracers. The performance of immunoassay can be influenced by the tracer structure (Choi et al., Citation2011). In this study, the IC50 (178 ng/mL) of tracer A was lower than that (401 ng/mL) of tracer B. The values ΔmP and ΔmP/IC50 of tracer A were 122 and 0.68, respectively. The values ΔmP and ΔmP/IC50 of tracer B were 74 and 0.18, respectively. The results indicated a successful synthesis of tracer A and tracer B, and the sensitivity of tracer A was better than that of tracer B (). Therefore, tracer A was selected for the further investigation.
FPIA optimization
In this study, the reaction kinetics, antibody dilution, tracers’ concentration and methanol concentration was varied. IC50 ΔmP and ΔmP/IC50 were used as criteria to evaluate the optimal conditions. The observed IC50 ΔmP and ΔmP/IC50 are shown in and . The lower IC50, higher ΔmP and ΔmP/IC50 were obtained when the reaction kinetics, antibody dilution, tracers’ concentration and methanol concentration were 14 min, 1:1000, 3 nmol/L, 5%, and pH and ionic strength of working buffer were 9.0 and 200 mmol/L, respectively. These results indicated that the developed FPIA will possess higher sensitivity under these optimized conditions. The achieved competitive inhibition curve is shown in . The IC50 was 125.1 ng/mL, the LOD (IC10) was 8.4 ng/mL and the dynamic working range (IC20–IC80) was from 17.1 to 915.4 ng/mL. The duration of the reaction kinetics was 14 min, and the LOD also met the requirement of corresponding MRLs.
Figure 4. Effects of the physicochemical parameters on FPIA performance and three parameters IC50, LOD and ΔmP were calculated. The results showed that the higher sensitivity was obtained when the antibody dilution, tracers’ concentration, methanol concentration were 1:1000, 3 nmol/L, 5%, and pH and ionic strength of working buffer were 9.0 and 200 mmol/L, respectively. (A, B, C, D, E were the calibration curves of the polyclonal antiserum anti-propisochlor, different concentrations of tracer A, different pH values of buffer solution, ionic strength of working buffer and different concentrations of methanol concentration, respectively.)
Molecular modeling for specificity
The cross reaction (CR) for 14 structural analogues () was lower than 0.60%, besides pretilachlor, benzoylprop ethyl, and acetochlor, with CR was 2.5%, 1.3%, and 11.6%, respectively. The results showed that the developed FPIA possesses high specificity to propisochlor.
Table 1. CR% of 17 structural analogues and molecular modeling.
Chloroacetamide herbicides include six herbicides, propisochlor, acetochlor, pretilachlor, alachlor, metolachlor and butachlor (Bernasinska et al., Citation2013). The antibody exhibited high specificity to propisochlor, and the CRs to acetochlor, pretilachlor, alachlor, metolachlor and butachlor were 11.6%, 2.5%, 0.6%, 0.4% and less than 0.01%, respectively.
The previous study indicated that the bulky butoxy-group of butachlor molecules was an important group of epitopes (Bach et al., Citation2016). To better understand the specificity, minimum energy conformation and electronic properties were discussed. Firstly, the six 3D structures of chloroacetamide herbicides are shown in , which present the global minimum energy structure in the same orientation. For the six herbicides, the benzene ring lies in the same plane, and the orientations of all chlorine were difference. The difference of alkoxyalkyl substituent caused a significant decrease of IC50 (more than 80% decrease) compared to that of propisochlor, and the increased length of alkoxyalkyl in butachlor resulted in the lowest antibody affinity (CR less than 0.01%). Moreover, the introduction of methyl or ethyl between nitrogen and alkoxyalkyl in metolachlor and pretilachlor causes a steric hindrance, thus resulting in chlorine element shift. The changed structure accounts for the low binding affinity for metolachlor and pretilachlor; CRs were 0.43% and 2.46%, respectively. Therefore, it was speculated that the alkoxyalkyl substituent significantly contributed to the interaction between the antibody and antigen.
The effect of electronic properties was also studied. The electrostatic potential was displayed over the electron density surfaces of the global minimum energy conformations of the six-chloroacetamide herbicides. The charges of the isosurfaces are color coded as shown in the legend of . The red region, purple region and cyan region represent positive charge, negative charge and neutral charge, respectively. And the color changing from red to purple indicates that the charge changed from positive to negative. Propisochlor, acetochlor, alachlor and butachlor have identical surface charges, the positions of red regions (electropositive atom) are similar, expect the olivine regions. The charge of the olivine regions of propisochlor results in significant increase of affinity, compared to no olivine region of acetochlor, alachlor and butachlor. According to the 3D structures of the four herbicides, the olivine regions contain the alkoxyalkyl. In contrast, metolachlor and pretilachlor show greater difference in the surface charge; there are purple regions (electronegative atom) around the red regions. These differences also resulted in a huge decrease in IC50 value. It was speculated that the alkoxyalkyl was significant for the antibody binding.
Development of FPIA in pond water
To estimate the matrix effect, sample dilution is a widely used method. As shown in , the standard curves of pond water (diluted five times) can match well with the curve prepared with BBT. The matrix effect could be removed after five times of dilution.
The applicability of the FPIA developed for propisochlor analysis in pond water samples was also estimated. Three spiked samples were prepared at concentrations of 50, 100 and 150 ng/mL and analyzed by the developed method. As seen from , the average recoveries of propisochlor from pond water were 80.0–86.6% with acceptable coefficients of variable (CVs) (13.8–17.2%). These results fulfilled the recommendations of the US Environment Protection Agency for analysis of the environmental sample (the recovery should be within 70–120%) (El Alfy & Faraj, 2016).
Table 2. Recovery of propisochlor from environmental water samples detected by the developed FPIA (n = 5).a
HPLC confirmation
The developed FPIA was also confirmed by HPLC. After obtaining data with the developed FPIA and HPLC methods, they were analyzed by Student’s t-test and the analytical result showed good correlation with R2 value of 0.9748. The HPLC confirmation results indicate that the developed FPIA could be used as a simplicity in sample preparation, high accuracy and high sample throughput analysis for determination of propisochlor in pond water samples.
Conclusions
In this study, two different tracers were synthesized to optimize FPIA performance. CR studies prove that the alkoxyalkyl of chloroacetamide herbicides possibly played a significant role in antibody binding according to the molecular modeling analysis. The duration of the FPIA was within 14 min, the 50% inhibition concentration (IC50) was 125.1 ng/mL, the LOD was 8.4 ng/mL without any CR with analogs. The recoveries of propisochlor in pond water ranged from 80.0% to 86.6%, which meet the recommendations of the US Environment Protection Agency. In conclusion, the firstly proposed FPIA could be used as a simplicity in sample preparation, high accuracy, and high sample throughput analysis for the determination of propisochlor in pond water samples.
Supplemental Material
Download MS Word (130.8 KB)Disclosure statement
No potential conflict of interest was reported by the authors.
Additional information
Funding
Notes on contributors
Yao Liu
Yao Liu, A Ph.D. student of South China Agricultural University.
Lan-Teng Wang
Lan-Teng Wang, A Ph.D. student of South China Agricultural University.
Kai Zhou
Kai Zhou, A Ph.D. student of South China Agricultural University.
Sergei A. Eremin
Sergei A. Eremin, A professor of MV Lomonosov Moscow State University.
Xin-An Huang
Xin-An Huang, A professor of Guangzhou University of Chinese Medicine.
Yuan-Ming Sun
Yuan-Ming Sun, A professor of South China Agricultural University.
Zhen-Lin Xu
Zhen-Lin Xu, A professor of South China Agricultural University.
Hong-Tao Lei
Hong-Tao Lei, A professor of South China Agricultural University.
References
- Bach, M., Diesner, M., Grossmann, D., Guerniche, D., Hommen, U., Klein, M., … Trapp, M. (2016). Pesticide exposure assessment for surface waters in the EU. Part 1: Some comments on the current procedure. Pest Management Science, 72, 1279–1284. doi: https://doi.org/10.1002/ps.4281
- Barbosa, A. M., Solano Mde, L., & Umbuzeiro Gde, A. (2015). Pesticides in drinking water – The Brazilian monitoring program. Frontiers in Public Health, 3, 246–255. doi: https://doi.org/10.3389/fpubh.2015.00246
- Baris, D., Waddell, R., Beane Freeman, L. E., Schwenn, M., Colt, J. S., Ayotte, J. D., … Silverman, D. T. (2016). Elevated bladder cancer in northern New England: The role of drinking water and arsenic. Journal of the National Cancer Institute, 108, djw099–djw108. doi: https://doi.org/10.1093/jnci/djw099
- Benito-Peña, E., Moreno-Bondi, M. C., Orellana, G., Maquieira, Á., & van Amerongen, A. (2005). Development of a novel and automated fluorescent immunoassay for the analysis of β-lactam antibiotics. Journal of Agricultural and Food Chemistry, 53, 6635–6642. doi: https://doi.org/10.1021/jf0511502
- Bernasinska, J., Duchnowicz, P., Koter-Michalak, M., & Koceva-Chyla, A. (2013). Effect of safeners on damage of human erythrocytes treated with chloroacetamide herbicides. Environmental Toxicology and Pharmacology, 36, 368–377. doi: https://doi.org/10.1016/j.etap.2013.04.010
- Brender, J. D., & Weyer, P. J. (2016). Agricultural compounds in water and birth defects. Current Environmental Health Reports, 3, 144–152. doi: https://doi.org/10.1007/s40572-016-0085-0
- Choi, E. H., Kim, D. M., Choi, S. W., Eremin, S. A., & Chun, H. S. (2011). Optimisation and validation of a fluorescence polarisation immunoassay for rapid detection of zearalenone in corn. International Journal of Food Science & Technology, 46, 2173–2181. doi: https://doi.org/10.1111/j.1365-2621.2011.02733.x
- Cruzeiro, C., Rocha, E., Pardal, M. A., & Rocha, M. J. (2016). Environmental assessment of pesticides in the Mondego river estuary (Portugal). Marine Pollution Bulletin, 103, 240–246. doi: https://doi.org/10.1016/j.marpolbul.2015.12.013
- Echeverry, G., Zapata, A. M., Paez, M. I., Mendez, F., & Pena, M. (2015). Evaluation of human health risk for a population from Cali, Colombia, by exposure to lead, cadmium, mercury, 2,4-dichloro-phenoxyacetic acid and diuron associated with water and food consumption. Biomedica: Revista del Instituto Nacional de Salud, 35(Spec), 110–119.
- El Alfy, M., & Faraj, T. (2017). Spatial distribution and health risk assessment for groundwater contamination from intensive pesticide use in arid areas. Environmental Geochemistry and Health, 39, 231–253. doi: https://doi.org/10.1007/s10653-016-9825-1
- Feng, J. L., Yu, H., Liu, S. H., Liu, M. L., & Sun, J. H. (2015). Distribution characteristics and ecological risk assessment of HCHs and DDTs in surface water bodies in Xinxiang. Huan Jing Ke Xue, 36, 2849–2856.
- Howe, P. L., Reichelt-Brushett, A. J., Clark, M. W., & Seery, C. R. (2017). Toxicity estimates for diuron and atrazine for the tropical marine cnidarian Exaiptasia pallida and in-hospite Symbiodinium spp. using PAM chlorophyll-a fluorometry. Journal of Photochemistry and Photobiology B: Biology, 171, 125–132. doi: https://doi.org/10.1016/j.jphotobiol.2017.05.006
- Kissane, Z., & Shephard, J. M. (2017). The rise of glyphosate and new opportunities for biosentinel early-warning studies. Conservation Biology, doi:https://doi.org/10.1111/cobi.12955.
- Kolosova, A., Maximova, K., Eremin, S. A., Zherdev, A. V., Mercader, J. V., Abad-Fuentes, A., & Dzantiev, B. B. (2017). Fluorescence polarisation immunoassays for strobilurin fungicides kresoxim-methyl, trifloxystrobin and picoxystrobin. Talanta, 162, 495–504. doi: https://doi.org/10.1016/j.talanta.2016.10.063
- Konda, L. N., & Pásztor, Z. (2001). Environmental distribution of acetochlor, atrazine, chlorpyrifos, and propisochlor under field conditions. Journal of Agricultural and Food Chemistry, 49, 3859–3863. doi: https://doi.org/10.1021/jf010187t
- Krotzky, A. J., & Zeeh, B. (1995). Pesticides report 33. Immunoassays for residue analysis of agrochemicals: Proposed guidelines for precision, standardization and quality control (technical report). Pure and Applied Chemistry, 67, 2065–2088. doi: https://doi.org/10.1351/pac199567122065
- Lei, H., Xue, G., Yu, C., Haughey, S. A., Eremin, S. A., Sun, Y., … Shen, Y. (2011). Fluorescence polarization as a tool for the detection of a widely used herbicide, butachlor, in polluted waters. Analytical Methods, 3, 2334–2340. doi: https://doi.org/10.1039/c1ay05347g
- Lu, Y., Peterson, J. R., Gooding, J. J., & Lee, N. A. (2012). Development of sensitive direct and indirect enzyme-linked immunosorbent assays (ELISAs) for monitoring bisphenol-A in canned foods and beverages. Analytical and Bioanalytical Chemistry, 403, 1607–1618. doi: https://doi.org/10.1007/s00216-012-5969-8
- Meire, R. O., Khairy, M., Targino, A. C., Galvao, P. M., Torres, J. P., Malm, O., & Lohmann, R. (2016). Use of passive samplers to detect organochlorine pesticides in air and water at wetland mountain region sites (S-SE Brazil). Chemosphere, 144, 2175–2182. doi: https://doi.org/10.1016/j.chemosphere.2015.10.133
- Mekonen, S., Argaw, R., Simanesew, A., Houbraken, M., Senaeve, D., Ambelu, A., & Spanoghe, P. (2016). Pesticide residues in drinking water and associated risk to consumers in Ethiopia. Chemosphere, 162, 252–260. doi: https://doi.org/10.1016/j.chemosphere.2016.07.096
- Oberleitner, L., Grandke, J., Mallwitz, F., Resch-Genger, U., Garbe, L. A., & Schneider, R. J. (2014). Fluorescence polarization immunoassays for the quantification of caffeine in beverages. Journal of Agricultural and Food Chemistry, 62, 2337–2343. doi: https://doi.org/10.1021/jf4053226
- Rauch, R., & Földényi, R. (2012). The effect of alginite on the decomposition of the herbicide propisochlor. Journal of Environmental Science and Health, Part B, 47, 670–676. doi: https://doi.org/10.1080/03601234.2012.669212
- Sekhotha, M. M., Monyeki, K. D., & Sibuyi, M. E. (2016). Exposure to agrochemicals and cardiovascular disease: A review. International Journal of Environmental Research and Public Health, 13, 299–241. doi: https://doi.org/10.3390/ijerph13020229
- Stevanovic, M., Gasic, S., Pipal, M., Blahova, L., Brkic, D., Neskovic, N., & Hilscherova, K. (2017). Toxicity of clomazone and its formulations to zebrafish embryos (Danio rerio). Aquatic Toxicology, 188, 54–63. doi: https://doi.org/10.1016/j.aquatox.2017.04.007
- Sturve, J., Scarlet, P., Halling, M., Kreuger, J., & Macia, A. (2016). Environmental monitoring of pesticide exposure and effects on mangrove aquatic organisms of Mozambique. Marine Environmental Research, 121, 9–19. doi: https://doi.org/10.1016/j.marenvres.2016.05.005
- Tufi, S., Wassenaar, P. N., Osorio, V., de Boer, J., Leonards, P. E., & Lamoree, M. H. (2016). Pesticide mixture toxicity in surface water extracts in snails (Lymnaea stagnalis) by an in vitro acetylcholinesterase inhibition assay and metabolomics. Environmental Science & Technology, 50, 3937–3944. doi: https://doi.org/10.1021/acs.est.5b04577
- Wang, Z., Zhang, S., Nesterenko, I. S., Eremin, S. A., & Shen, J. (2007). Monoclonal antibody-based fluorescence polarization immunoassay for sulfamethoxypyridazine and sulfachloropyridazine. Journal of Agricultural and Food Chemistry, 55, 6871–6878. doi: https://doi.org/10.1021/jf070948d
- Wu, X., Xu, J., Liu, X., Dong, F., Wu, Y., Zhang, Y., & Zheng, Y. (2013). Determination of herbicide propisochlor in soil, water and rice by quick, easy, cheap, effective, rugged and safe (QuEChERS) method using by UPLC-ESI-MS/MS. Bulletin of the Korean Chemical Society, 34, 917–921. doi: https://doi.org/10.5012/bkcs.2013.34.3.917
- Yakovleva, J. N., Lobanova, A. I., Panchenko, O. A., & Eremin, S. A. (2002). Production of antibodies and development of specific polarization fluoroimmunoassay for acetochlor. International Journal of Environmental Analytical Chemistry, 82, 851–863. doi: https://doi.org/10.1080/0306731021000102310
- Yakovleva, J., Zherdev, A. V., Popova, V. A., Eremin, S. A., & Dzantiev, B. B. (2003). Production of antibodies and development of enzyme-linked immunosorbent assays for the herbicide butachlor. Analytica Chimica Acta, 491, 1–13. doi: https://doi.org/10.1016/S0003-2670(03)00796-7
- Zhang, X., Eremin, S. A., Wen, K., Yu, X., Li, C., Ke, Y., … Wang, Z. (2017). Fluorescence polarization immunoassay based on a new monoclonal antibody for the detection of the zearalenone class of mycotoxins in maize. Journal of Agricultural and Food Chemistry, 65, 2240–2247. doi: https://doi.org/10.1021/acs.jafc.6b05614
- Zhang, J., Zheng, J. W., Liang, B., Wang, C. H., Cai, S., Ni, Y. Y., … Li, S. P. (2011). Biodegradation of chloroacetamide herbicides by Paracoccus sp. FLY-8 in vitro. Journal of Agricultural and Food Chemistry, 59, 4614–4621. doi: https://doi.org/10.1021/jf104695g