ABSTRACT
A signal-enhanced lateral flow strip biosensor has been constructed for ultrasensitive and on-site visual detection of bisphenol A (BPA). The signal amplification principle is based on the capacity of binding a large number of antibody-assembled gold nanoparticle (AuNP–antibody) conjugates of the poly amidoamine (PAMAM), which bears a multitude of amino groups. This signal-enhanced method could achieve BPA detection at 10 ppb based on naked eye visual observation, which represents an at least 50-fold improvement of the sensitivity of a traditional method without PAMAM. Moreover, good linearity was observed in the range from 2 to 100 ppb after quantitative analysis using the ImageJ software. This signal amplification method could be adopted as a potential generous technique in different fields.
1. Introduction
Bisphenol A (BPA) is extensively used in the production of polycarbonate and epoxy resin products. Considering its ubiquity, it is not uncommon for it to leach from food and beverage containers, as well as some dental sealants and composites, under normal conditions of use (Geens et al., Citation2012; Sun, Kang, et al., Citation2016; Vandenberg, Maffini, Sonnenschein, Rubin, & Soto, Citation2009). It has been shown that BPA can mimic the effects of endogenous hormones, namely estrogens and androgens, by binding to estrogen receptor sites (Vandenberg et al., Citation2009). Recently, several review articles have focused on the links between BPA exposure and human health (Chen, Shen, & Chen, Citation2016), and there is an increasing body of evidence that environmental BPA exposure can adversely affect humans, especially through elevating the risks of obesity, diabetes, and coronary heart disease (Cabaton et al., Citation2010; Rezg, El-Fazaa, Gharbi, & Mornagui, Citation2014; Rubin, Citation2011). In view of the potential harm of BPA, a great deal of effort has been directed towards establishing effective, ultrasensitive, and rapid methods for its detection in food samples and food containers, which is of great importance for assuring human health. In particular, for drinking water, BPA released from the plastic packages or cups should be accurately monitored on-site (Mielke & Gundertremy, Citation2012).
The conventional methods for low-level BPA detection are instrument-based, such as high-performance liquid chromatography (Zhou, Gao, & Xie, Citation2011), liquid chromatography/mass spectrometry (Halle, Claparols, Garrigues, Franceschi-Messant, & Perez, Citation2015; Yazdinezhad, Ballesteros-Gómez, Lunar, & Rubio, Citation2013), gas chromatography/mass spectrometry (Ros et al., Citation2015), and so on. However, the high cost of various instruments, and the need for time-consuming sample pre-treatments and expert personnel to carry out operations, greatly limits the wide application of these methods. Considering the urgent need to monitor the presence of BPA in daily water in relation to BPA-containing food contact materials, developing an easy and sensitive method for on-site detection of BPA is of critical significance for food safety and public health (Mei, Qu, et al., Citation2013). Meanwhile, various antibody-based rapid detection approaches have been established, which are characterized by high sensitivity and low cost. Enzyme-linked immunosorbent assay was the initial method of choice (Lei et al., Citation2013; Maiolini et al., Citation2014), and with the advent of electrochemical biosensors, an electrochemical immunosensor for BPA with “signal-on” detection based on square-wave voltammetry was fabricated (Wang et al., Citation2013). Besides, aptamer have received considerable attention as recognition probes in bioassays, and methods for the trace detection of BPA based on fluorescence resonance energy transfer (Zhu et al., Citation2015) and a label-free aptasensor based on colorimetric detection (Mei, Chu, et al., Citation2013) have been developed. In addition, an electrochemical aptasensor for screening drinking water for the presence of BPA has also been reported (Xue et al., Citation2013). However, the stabilities and reproducibilities of these methods were not wholly satisfactory.
To overcome the aforementioned drawbacks, in recent years, strip biosensors have received considerable attention due to their portability, rapid assay times, cost-effectiveness, and ease of use, and especially for their excellent repeatability (Kong, Xie, Liu, Song, & Kuang, Citation2017; Sun, Liu, Song, Kuang, & Xu, Citation2016; Yu, Liu, Song, Kuang, & Xu, Citation2016). In order to detect BPA, an immunochromatographic lateral flow strip was developed (Mei, Deng, et al., Citation2013), which was directly applied in the analysis of real spiked water samples. Furthermore, in order to enhance the detection sensitivity, a signal-amplified method was further established on the basis of the aforementioned report (Mei, Chu, et al., Citation2013). It involved two conjugation pads bearing two respective kinds of gold nanoparticles (AuNPs) on the lateral flow strip, one of which was the same as the conventional pad for antibody labeling, whereas the second was for signal amplification. Assembly of the signal-amplified pad required significant amounts of AuNPs and antibody. However, if the poly amidoamine (PAMAM) could be applied, the cost would be greatly reduced.
PAMAM is a polyamide-amine-type dendrimer. It is widely used in the biomedical field as carrier systems for drugs, genes, and vaccines, in drug release and targeted drug delivery (Alibolandi et al., Citation2017; Sharma et al., Citation2017). Rely on their large numbers of terminal functional groups, PAMAM can greatly increase the quantity of attached various biomolecules such as antibody, enzyme, or aptamer, and consequently improve sensitivity of detection (Akter, Jeong, Lee, Choi, & Rahman, Citation2017; Malvano, Albanese, Pilloton, & Matteo, Citation2017; Miodek, Omrani, Khoder, & Youssoufi, Citation2016). Meanwhile, it was also extensively applied in electrochemical sensor in order to additionally amplify the sensitivity and reach a low detection limit through association with others conductive nanomaterials such as AuNPs, Ag nanoparticles, grapheme, and so on (Araque et al., Citation2014; Karadag, Geyik, Demirkol, Ertas, & Timur, Citation2013; Ning, Zhang, & Zheng, Citation2014). However, to the best of our knowledge, it has rarely been used as a sensitization medium in lateral flow strip for the signal-enhancement process (Shen, Xu, Gurung, Yang, & Liu, Citation2013).
Herein, we describe the development of a disposable strip biosensor for the amplified detection of BPA by binding a multitude of AuNP–antibody conjugates using the PAMAM (). Even with a single anchoring of BPA–bovine serum albumin (BSA) against antibody–PAMAM, numerous AuNPs would be captured on a test line (T line), leading to an obvious change of the intensity color. Accordingly, if a tiny amount of BPA was present in the sample to be tested, a large number of AuNPs would be displaced, resulting in elimination of the distinct color of the T line. In this way, sensitization was achieved. Moreover, by using PAMAM, the amount of antibody needed was greatly reduced.
2. Materials and methods
2.1. Materials
Tween-20, BSA, and trisodium citrate were purchased from Sangon Biotech Co., Ltd. (Shanghai, China). Antibody against BPA and BPA–BSA conjugates were purchased from Wuxi Determine Bio-Tech Co., Ltd (Wuxi, China). PAMAM (G4.0) was purchased from Sigma-Aldrich. The nitrocellulose (NC) membrane was ordered from Whatman GmbH (Dassel, Germany), and the glass fiber membranes were bought from Shanghai Kinbio Tech Co., Ltd (Shanghai, China). The absorbent pad was purchased from Millipore Crop. (USA). Semi-rigid polyethylene sheets and adhesive tape were also purchased from Shanghai Joey Biotechnology Company (Shanghai, China). 1-ethyl-3-(3-dimethyl aminopropyl) carbodiimide (EDC), N-hydroxysuccinimide (NHS), HAuCl4, and BPA standard sample were purchased from the J&K Scientific Ltd (http://www.jkchemical.com/). All standard solutions for BPA and the analogues were prepared by dilution of stock solutions of these compounds (1 mg/mL in methanol).
2.2. Preparation of AuNPs
AuNPs with average diameters of 15, 25, and 40 nm were prepared by the classic trisodium citrate reduction method according to previous reports with a slight modification (Deng et al., Citation2015; Mei, Chu, et al., Citation2013), varying the dosage of trisodium citrate used as the reducing reagent. Before the preparation, all glassware were cleaned in a solution composed of three parts 12 M HCl and 1 part 8 M HNO3 by volume, rinsed with NANO pure H2O, and then oven-dried prior to use. Briefly, 1 wt% trisodium citrate was added to a boiling solution of HAuCl4 (50 mL, 1 mM). After a color change from pale-yellow to wine-red, the solution was maintained under reflux for a further 10 min, then removed from the heat and stirred for a further 5 min. It was allowed to cool to room temperature and stored at 4°C prior to use.
2.3. Preparation of antibody–PAMAM
Since PAMAM bears a multitude of amino groups, we firstly activated carboxy groups of the antibody with 10 mg/mL EDC and NHS for 30 min at room temperature. Thereafter, 2 μL of 0.2 mg/mL PAMAM was added and allowed to react with the activated antibody for 30 min to obtain the PAMAM–antibody conjugate.
2.4. Preparation of AuNP–antibody–PAMAM conjugation
AuNP–antibody conjugates were prepared as reported previously (Mei, Qu, et al., Citation2013). Firstly, the colloidal gold solution was adjusted to pH 8.0 with 0.1 M K2CO3, and then the solution was mixed with the prepared PAMAM–antibody and incubated for 1 h under agitation. Thereafter, 30 μL of blocking agent was added and agitation was continued for a further 30 min. The mixture was centrifuged at 9200g for 10 min, and the pellet obtained was resuspended in 0.5 mL of buffer (10% BSA, 2% sucrose) to yield the AuNP–antibody–PAMAM conjugate solution. The conjugate solution was stored at 4°C before use. The preparation steps used to obtain traditional AuNP–antibody conjugates were similar to those outlined above, but with the omission of PAMAM.
2.5. Preparation of the strip biosensor
The strip consists of four components: sample pad, conjugate pad, NC membrane, and absorption pad. All the components were mounted on a common backing layer. The sample pad (17 mm × 30 cm) was made from glass fiber and saturated with a buffer (pH 8.0) containing 0.25% Triton X-100, 20 mM Tris-HCl, and 150 mm NaCl. It was then dried at 37°C for 2 h and stored in a desiccator at room temperature. Meanwhile, the aforementioned AuNP–antibody conjugate was sprayed on the glass fiber membrane (5 mm × 30 cm) as the conjugated pad. The BPA–BSA conjugate solution and goat–anti-mouse antibody solution were sprayed on the NC membrane (25 mm × 30 cm) as the test line (T line) and control line (C line), respectively, and then dried at 37°C for 2 h. Finally, the sample pad, conjugate pad, NC membrane, and absorption pad were assembled on a plastic adhesive backing (60 mm × 30 cm), with each part overlapped by 2 mm to ensure migration of the solution through the strip during the assay. Strips of width 4 mm were cut using a programmable strip cutter.
2.6. Analytical procedure
Sample solutions containing different concentrations of BPA were prepared by spiking BPA in running buffer. In a typical test, 70 μL of sample solution was dropped onto the sample pad, and after 10 min, visual detection of BPA was easily realized by observing the color of the test zone of the strip. Quantitative analysis could be further carried out by determining the intensities of the test and control lines using ImageJ.
3. Results and discussion
3.1. Verification of the preparation of AuNP–antibody–PAMAM conjugation
As PAMAM is used in the signal enhancement of the lateral flow strip, and the preparation of the AuNP–antibody–PAMAM is a crucial step in the whole process, the successful preparation of AuNP–antibody is significant. Therefore, we recorded UV/Vis spectra of the AuNPs, AuNP–antibody, and AuNP–antibody–PAMAM. From the results shown in (a), it can be seen that, as expected, the characteristic absorption of the AuNPs at 518 nm was red-shifted to 524 nm after conjugation with the antibodies and further to about 536 nm when associated with PAMAM, indicating successful conjugation. Furthermore, we estimated the hydraulic diameters of the AuNPs and the respective complexes through dynamic light scattering (DLS). The results demonstrated that the original average diameter of the AuNPs was 25.66 nm ((b)), and the value increased after conjugated with the antibodies and PAMAM ((c,d)). There results further demonstrate successful preparation of the conjugates.
3.2. Choice of running buffer
It has been reported that a suitable running buffers crucial for the test sensitivity of the strip (Mei, Qu, et al., Citation2013), since this would control the migration speed and allow efficient reaction between the recognition conjugate and the target analyte or capture antibody on the strip. In this regard, we examined three kinds of running buffers for BPA detection (0, 20, 50, 100, 500 ppb). The results shown in indicate that the optimal running buffer for detection was C. Compared with the detection results using running buffers A and B, the line with buffer C was unambiguous, and the T line of the blank sample was also clearly discernible. Moreover, with increasing concentration of BPA, the intensity of the T line faded. Therefore, running buffer C was adopted for all further investigations.
3.3. Influence of the AuNPs diameter
In this method, the AuNPs also play a vital role in the sensitivity, because their diameter influences the migration speed. Besides, the stability of the particles is also related to their diameter. Hence, the diameter of the AuNPs was another factor taken into account for the parameter optimization. Three different diameters of AuNPs (15, 25, and 40 nm) were chosen and assessed for optimal labeling of the antibody. From (b), it can be seen that as the diameter of the AuNPs was increased, their color gradually darkened and a distinctred shift of their characteristic absorption was also observed. As shown in (a), use of the 25 nm AuNPs for labeling gave T and C lines with the optimum shape and color. The color of the 15 nm AuNPs was too light to generate distinct lines, whereas that of the 40 nm AuNPs was too dark. Moreover, the diameter of the 40 nm particles somewhat hindered migration of the complexes, leading to non-ideal sensitivity.
3.4. Detection of BPA with PAMAM-based signal-amplified lateral flow strip
The structure and the detection principle of an immunochromatographic lateral flow strip were shown in . In the traditional lateral flow strip, the conjugates of anti-BPA antibodies with AuNPs were used as recognition probes, and BPA–BSA conjugate and anti-mouse antibodies were coated on the test line and control line, respectively. Compared with the traditional lateral flow strip, we exploited the abundance of amino groups on the PAMAM molecule as a signal-enhancement component to capture and accumulate large numbers of AuNPs through the antibody. At the same time, it was shown that the application of PAMAM is also beneficial for conservation of the antibody. As shown in , both the process with and without PAMAM used the same tiny volume of antibody (0.4 μL, 1 mg/mL) in the preparation of AuNP–antibody–PAMAM and AuNP–antibody conjugates, but the intensities of the lines were distinctly different. Using PAMAM, both the T and C lines of the strips were intensified several-fold. This was because the amino groups of PAMAM allowed the concentration of a large amount of colloidal gold in the dendrimer through the antibody. The fixation efficiency of AuNPs on the test paper was greatly improved, so the color of the lines was greatly intensified. Furthermore, the color of the strips when using PAMAM was quite dark, as shown in (right), so the amount of antibody used could be further reduced.
3.5. Sensitivity of the signal-amplified lateral flow strip
Under the optimal conditions, the sensitivity of the assembled signal-enhanced lateral flow strip was examined by using standard BPA solutions at different concentrations, as shown on the right of (a). For comparison, a conventional lateral flow strip without PAMAM-based signal amplification for BPA detection was also used as a control (left). From the results shown in (a), it is evident that the intensities of the test lines on the two kinds of lateral flow strips decreased with increasing BPA concentration. Empirically, the limit of detection (LOD) observable by naked eye could be determined as the minimum target concentration causing the color on the test line to disappear. On this basis, the LOD of the traditional lateral flow strip could be estimated as 500 ppb, whereas that of the PAMAM-based enhanced method was 10 ppb, representing a 50-fold improvement in sensitivity.
Figure 5. Photo images of detection results of the BPA using traditional method (a-left) and PAMAM-based method (a-right). ImageJ scanning result and the corresponding calibration curve for BPA detection by the PAMAM-based method are shown in (b) and (c), respectively.
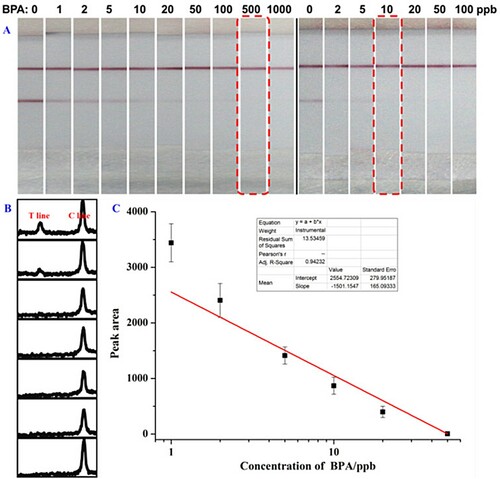
Quantitative detection using the PAMAM-based strip was further investigated using ImageJ software, as shown in (b). Two peaks are seen in the graph. The left peak corresponds to the intensity of the T line on the strip, while the right peak corresponds to the intensity of the C line. The BPA concentrations were 0, 2, 5, 10, 20, 50, and 100 ppb from top to bottom in (b). With the increase of BPA concentration in the solution, the peak intensity of the C line showed negligible changed, while the peak intensity of the T line decreased. This could be rationalized in terms of the adopted direct competitive immunoreaction model. Besides, the average values of three parallel samples at the same concentration were utilized for calibration curve construction ((c)), and good linearity was observed between 2 and 100 ppb.
4. Conclusion
In summary, we have successfully developed a signal-enhanced lateral flow strip biosensor for the visual detection of BPA based on PAMAM binding with antibody. The generated signals (the T line on the strip) can be unambiguously read by the naked eye. The LOD was 10 ppb, which represents a 50-fold enhancement compared with sensor without signal amplification. Good linearity was also achieved by ImageJ software analysis. Importantly, the signal-enhanced strip biosensor is simple to operate and shows a rapid response. By virtue of these excellent features, it should offer a promising sensing platform for routine on-site monitoring of BPA in complex samples. Besides, it is also a material saving and economical method. By using PAMAM, a 10-fold reduction in the amount of antibody required for AuNPs assembly can be achieved. Thus, we believe that this signal amplification technique has outstanding potential in the application of immunochromatographic test strips.
Disclosure statement
No potential conflict of interest was reported by the authors.
Additional information
Funding
Notes on contributors
Xiayu Peng
Xiayu Peng received his Ph.D. in cell engineering from Shihezi University in 2010. His research interests include the synthesis and application of biodegradable polymers in the differentiation modulation of reproductive cells and animal rapid detection technology of zoonoses.
Lichao Kang
Lichao Kang obtained his MS degree in preventive veterinary medicine from Shihezi University in 2007. His current research interests focus on prevention of animal epidemic diseases and detection of food microorganisms.
Fangqin Pang
Fangqin Pang got her bachelor’s degree in food science and technology from Xinjiang University. Her current research interests include immunoassay applications in food.
Hongmin Li
Hongmin Li received her bachelor’s degree in Agricultural Resources and Environment from Tarim University in 2005. Her current research interest is immunoassay applications in food.
Ruifeng Luo
Ruifeng Luo is a senior experimenter at Xinjiang Academy of Agricultural and Reclamation Science. He got his bachelor’s degree in chemistry in 2003 from Shihezi University. His research work is the food safety analysis.
Xiaoling Luo
Xiaoling Luo received her bachelor’s and master’s degrees in Environmental Engineering at the Shihezi University and Northwest Agricultural & Forest University, respectively. Her current research interests include risk assessment and food safety.
Fengxia Sun
Fengxia Sun received her Ph.D. degree in Food Science from Jiangnan University in 2011 and then began to work as an associate professor in Xinjiang Academy of Agriculture and Reclamation Science. Her research interest is food safety analysis.
References
- Akter, R., Jeong, B., Lee, Y. M., Choi, J. S., & Rahman, M. A. (2017). Femtomolar detection of cardiac troponin I using a novel label-free and reagent-free dendrimer enhanced impedimetric immunosensor. Biosensors and Bioelectronics, 91, 637–643. doi: https://doi.org/10.1016/j.bios.2017.01.021
- Alibolandi, M., Taghdisi, S. M., Ramezani, P., Shamili, F. H., Farzad, S. A., Abnous, K., & Ramezani, M. (2017). Smart AS1411-aptamer conjugated pegylated PAMAM dendrimer for the superior delivery of camptothecin to colon adenocarcinoma in vitro and in vivo. International Journal of Pharmaceutics, 519, 352–364. doi: https://doi.org/10.1016/j.ijpharm.2017.01.044
- Araque, E., Arenas, C. B., Gamella, M., Reviejo, J., Villalonga, R., & Pingarrón, J. M. (2014). Graphene–polyamidoamine dendrimer–Pt nanoparticles hybrid nanomaterial for the preparation of mediatorless enzyme biosensor. Journal of Electroanalytical Chemistry, 717-718, 96–102. doi: https://doi.org/10.1016/j.jelechem.2014.01.016
- Cabaton, N. J., Wadia, P. R., Rubin, B. S., Zalko, D., Schaeberle, C. M., Askenase, M. H., … Soto, A. M. (2010). Perinatal exposure to environmentally relevant levels of bisphenol A decreases fertility and fecundity in CD-1 mice. Environmental Health Perspectives, 119, 547–552. doi: https://doi.org/10.1289/ehp.1002559
- Chen, W. Y., Shen, Y. P., & Chen, S. C. (2016). Assessing bisphenol A (BPA) exposure risk from long-term dietary intakes in Taiwan. Science of the Total Environment, 543(Pt A), 140–146. doi: https://doi.org/10.1016/j.scitotenv.2015.11.029
- Deng, Y., Wang, X., Xue, F., Zheng, L., Liu, J., Yan, F., … Chen, W. (2015). Ultrasensitive and rapid screening of mercury(II) ions by dual labeling colorimetric method in aqueous samples and applications in mercury-poisoned animal tissues. Analytica Chimica Acta, 868, 45–52. doi: https://doi.org/10.1016/j.aca.2015.02.003
- Geens, T., Aerts, D., Berthot, C., Bourguignon, J. P., Goeyens, L., Lecomte, P., … Covaci, A. (2012). A review of dietary and non-dietary exposure to bisphenol-A. Food and Chemical Toxicology, 50, 3725–3740. doi: https://doi.org/10.1016/j.fct.2012.07.059
- Halle, A. T., Claparols, C., Garrigues, J. C., Franceschi-Messant, S., & Perez, E. (2015). Development of an extraction method based on new porous organogel materials coupled with liquid chromatography-mass spectrometry for the rapid quantification of bisphenol A in urine. Journal of Chromatography A, 1414, 1–9. doi: https://doi.org/10.1016/j.chroma.2015.07.046
- Karadag, M., Geyik, C., Demirkol, D. O., Ertas, F. N., & Timur, S. (2013). Modified gold surfaces by 6-(ferrocenyl)hexanethiol/dendrimer/gold nanoparticles as a platform for the mediated biosensing applications. Materials Science and Engineering: C, 33(2), 634–640. doi: https://doi.org/10.1016/j.msec.2012.10.008
- Kong, D. Z., Xie, Z. J., Liu, L. Q., Song, S. S., & Kuang, H. (2017). Development of ic-ELISA and lateral-flow immunochromatographic assay strip for the detection of citrinin in cereals. Food and Agricultural Immunology, 28, 754–766. doi: https://doi.org/10.1080/09540105.2017.1312293
- Lei, Y. J., Fang, L. Z., Akash, M. S. H., Liu, Z. M., Shi, W. X., & Chen, S. Q. (2013). Development and comparison of two competitive ELISAs for the detection of bisphenol A in human urine. Analytical Methods, 5, 6106–6113. doi: https://doi.org/10.1039/c3ay41023d
- Maiolini, E., Ferri, E., Pitasi, A. L., Montoy, A., Giovanni, M. D., Errani, E., & Girotti, S. (2014). Bisphenol A determination in baby bottles by chemiluminescence enzyme-linked immunosorbent assay, lateral flow immunoassay and liquid chromatography tandem mass spectrometry. The Analyst, 139, 318–324. doi: https://doi.org/10.1039/C3AN00552F
- Malvano, F., Albanese, D., Pilloton, R., & Matteo, M. D. (2017). A new label-free impedimetric aptasensor for gluten detection. Food Control, 79, 200–206. doi: https://doi.org/10.1016/j.foodcont.2017.03.033
- Mei, Z. L., Chu, H. Q., Chen, W., Xue, F., Liu, J., Xu, H. N., … Zheng, L. (2013). Ultrasensitive one-step rapid visual detection of bisphenol A in water samples by label-free aptasensor. Biosensors and Bioelectronics, 39, 26–30. doi: https://doi.org/10.1016/j.bios.2012.06.027
- Mei, Z. L., Deng, Y., Chu, H. Q., Xue, F., Zhong, Y. H., Wu, J. J., … Chen, W. (2013). Immunochromatographic lateral flow strip for on-site detection of bisphenol A. Microchimica Acta, 180, 279–285. doi: https://doi.org/10.1007/s00604-012-0930-2
- Mei, Z. L., Qu, W., Deng, Y., Chu, H. Q., Cao, J. X., Xue, F., … Chen, W. (2013). One-step signal amplified lateral flow strip biosensor for ultrasensitive and on-site detection of bisphenol A (BPA) in aqueous samples. Biosensors and Bioelectronics, 49, 457–461. doi: https://doi.org/10.1016/j.bios.2013.06.006
- Mielke, H., & Gundertremy, U. (2012). Physiologically based toxicokinetic modelling as a tool to support risk assessment: Three case studies. Journal of Toxicology, 2012(4), Article ID 359471.
- Miodek, A., Omrani, N. M., Khoder, R., & Youssoufi, H. K. (2016). Electrochemical functionalization of polypyrrole through amine oxidation of poly(amidoamine) dendrimers: Application to DNA biosensor. Talanta, 154, 446–454. doi: https://doi.org/10.1016/j.talanta.2016.03.076
- Ning, D. L., Zhang, H. F., & Zheng, J. B. (2014). Electrochemical sensor for sensitive determination of nitrite based on the PAMAM dendrimer-stabilized silver nanoparticles. Journal of Electroanalytical Chemistry, 717-718, 29–33. doi: https://doi.org/10.1016/j.jelechem.2013.12.011
- Rezg, R., El-Fazaa, S., Gharbi, N., & Mornagui, B. (2014). Bisphenol A and human chronic diseases: Current evidences, possible mechanisms, and future perspectives. Environment International, 64(3), 83–90. doi: https://doi.org/10.1016/j.envint.2013.12.007
- Ros, O., Vallejo, A., Blanco-Zubiaguirre, L., Olivares, M., Delgado, A., Etxebarria, N., & Prieto, A. (2015). Microextraction with polyethersulfone for bisphenol-A, alkylphenols and hormones determination in water samples by means of gas chromatography-mass spectrometry and liquid chromatography-tandem mass spectrometry analysis. Talanta, 134, 247–255. doi: https://doi.org/10.1016/j.talanta.2014.11.015
- Rubin, B. S. (2011). Bisphenol A: An endocrine disruptor with widespread exposure and multiple effects. The Journal of Steroid Biochemistry and Molecular Biology, 127, 27–34. doi: https://doi.org/10.1016/j.jsbmb.2011.05.002
- Sharma, A. K., Gothwal, A., Kesharwani, P., Alsaab, H., Iyer, A. K., & Gupta, U. (2017). Dendrimer nanoarchitectures for cancer diagnosis and anticancer drug delivery. Drug Discovery Today, 22(2), 314–326. doi: https://doi.org/10.1016/j.drudis.2016.09.013
- Shen, G. Y., Xu, H., Gurung, A. S., Yang, Y. H., & Liu, G. D. (2013). Lateral flow immunoassay with the signal enhanced by gold nanoparticle aggregates based on polyamidoamine dendrimer. Analytical Sciences, 29(8), 799–804. doi: https://doi.org/10.2116/analsci.29.799
- Sun, F. X., Kang, L. C., Xiang, X. L., Li, H. M., Luo, X. L., Luo, R. F., … Peng, X. Y. (2016). Recent advances and progress in the detection of bisphenol A. Analytical & Bioanalytical Chemistry, 408(25), 1–15. doi: https://doi.org/10.1007/s00216-016-9791-6
- Sun, C., Liu, L. Q., Song, S. S., Kuang, H., & Xu, C. L. (2016). Development of a highly sensitive ELISA and immunochromatographic strip to detect pentachlorophenol. Food and Agricultural Immunology, 27(5), 689–699. doi: https://doi.org/10.1080/09540105.2016.1148668
- Vandenberg, L. N., Maffini, M. V., Sonnenschein, C., Rubin, B. S., & Soto, A. M. (2009). Bisphenol-A and the great divide: A review of controversies in the field of endocrine disruption. Endocrine Reviews, 30, 75–95. doi: https://doi.org/10.1210/er.2008-0021
- Wang, X., Reisberg, S., Serradji, N., Anquetin, G., Pham, M. C., Wu, W., … Piro, B. (2013). E-assay concept: Detection of bisphenol A with a label-free electrochemical competitive immunoassay. Biosensors & Bioelectronics, 53C, 214–219.
- Xue, F., Wu, J. J., Chu, H. Q., Mei, Z. L., Ye, Y. K., Liu, J., … Chen, W. (2013). Electrochemical aptasensor for the determination of bisphenol A in drinking water. Microchimica Acta, 180, 109–115. doi: https://doi.org/10.1007/s00604-012-0909-z
- Yazdinezhad, S. R., Ballesteros-Gómez, A., Lunar, L., & Rubio, S. (2013). Single-step extraction and cleanup of bisphenol A in soft drinks by hemimicellar magnetic solid phase extraction prior to liquid chromatography/tandem mass spectrometry. Analytica Chimica Acta, 778, 31–37. doi: https://doi.org/10.1016/j.aca.2013.03.025
- Yu, L., Liu, L. Q., Song, S. S., Kuang, H., & Xu, C. L. (2016). Development of an immunochromatographic test strip and ic-ELISA for tetrabromobisphenol: A detection in lake water and rice pudding samples. Food and Agricultural Immunology, 27(4), 460–470. doi: https://doi.org/10.1080/09540105.2015.1126234
- Zhou, Q. X., Gao, Y. Y., & Xie, G. H. (2011). Determination of bisphenol A, 4-n-nonylphenol, and 4-tert-octylphenol by temperature-controlled ionic liquid dispersive liquid-phase microextraction combined with high performance liquid chromatography-fluorescence detector. Talanta, 85, 1598–1602. doi: https://doi.org/10.1016/j.talanta.2011.06.050
- Zhu, Y. Y., Cai, Y. L., Xu, L. G., Zheng, L. X., Wang, L. M., Qi, B., & Xu, C. L. (2015). Building an aptamer/graphene oxide FRET biosensor for one-step detection of bisphenol A. ACS Applied Materials & Interfaces, 7, 7492–7496. doi: https://doi.org/10.1021/acsami.5b00199