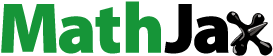
ABSTRACT
A sensitive and rapid magnetic-nanoparticles-based fluorescent immunoassay (MNPs-FIA) for individual and simultaneous determination of dichlorvos (DDVP) and paraoxon in untreated raw milk was developed. The conjugates DDVP–cBSA (cationized bovine serum albumin) and paraoxon–BSA (bovine serum albumin) were labeled by fluorescent dyes. The optimal amount of immobilized polyclonal anti-DDVP and anti-paraoxon antibodies on MNPs (0.375 mg Ab-MNPs) and optimal concentrations of DDVP–cBSA–FITC (18 µg/mL) and paraoxon–BSA–FITC (22 µg/mL) for MNPs-FIA were determined. The calibration curves of individual and simultaneous immunoassays of DDVP and paraoxon were investigated. The IC50 value of the individual paraoxon assay in raw cow’s milk was 60 ng/mL and linear range 2–300 ng/mL. The IC50 value of the individual DDVP assay was 70 ng/mL and linear range 5–300 ng/mL. The IC50 values of the paraoxon and DDVP (100 and 120 ng/mL respectively) in simultaneous assay were higher than those of the individual ones, and their linear ranges were 10–400 ng/mL.
Introduction
There is growing public concern over environmental pollution from pesticides. Human exposure to pesticides is multi-media and multiroute. This scenario allows for exposure to multiple pesticides, with resultant body burdens of these and their metabolites. That means, it is very important to carry out continuous pesticide control by multiplexed analysis.
Analytical methods to determine pesticides such as gas chromatography and liquid chromatography coupled with mass spectrometry or tandem mass spectrometry (MS/MS) have been developed (Darling, Laing, & Harkness, Citation1974; Díaz-Cruz, de Alda, López, & Barcelo, Citation2003). However, such techniques are limited by several drawbacks, including substantial equipment costs and/or extensive and time-consuming sample pretreatments, rendering progesterone routine determination and expensive analysis. Alternatively, ELISA is the most popular approach nowadays for the determination of pesticides (Chen et al., Citation2017; Gillis, Gosling, Sreenanc, & Kanea, Citation2002; Joel et al., Citation2017; Li et al., Citation2017; Watanabe, Miyake, & Yogo, Citation2013; Xu et al., Citation2010; Xu et al., Citation2012). But these analyses measured separated pesticides, independently, one at a time. To measure numerous analytes, sequential single measurements are needed. Immunofluorescence heterogeneous analyses permit to create multiplexing analysis by using microbead immunosorbents (Biagini et al., Citation2002; Biagini et al., Citation2003; Biagini et al., Citation2004; Fulton, McDade, Smith, Kienker, & Kettman, Citation1997; Vignali, Citation2000). The choice of solid phase is important to enhance the sensitivity in heterogeneous immunochemical assays. Magnetic nanoparticles (MNPs) as a solid phase were very suitable. It has been reported that the use of MNPs may assist in minimizing matrix interferences and reduce the immobilization time of antibody on MNPs to 2.5 h (Ivanova & Godjevargova, Citation2015; Yamaura et al., Citation2004). Also, a comfortable separation of bound and free fraction of the tracer can be performed only through a simple collection of the magnetic particles by a permanent magnet (Tudorache, Co, Lifgren, & Emneus, Citation2005; Tudorache, Zdrojewska, & Emneus, Citation2006).
Organophosphorus (OP) pesticides are one of the world’s most widely used pesticides, which are considered hazardous substances due to their high toxicity and their persistence in the environment (Roex, Keijzers, & van Gestel, Citation2003). Dichlorvos (DDVP) is widely used to control household, public health, and stored product insects (Celik, Yilmaz, & Turkoglu, Citation2009). In some countries, high levels of DDVP can be found in food and food products (Cengiz, Certel, & Göçmen, Citation2006). Paraoxon has never been registered for use as a pesticide. The sole source of methyl paraoxon in the environment is from the oxidation of methyl parathion, and for this reason few immunoassay analyses were developed (Li et al., Citation2009).
Milk is a very important food in the diet of infants and children. The presence of any pesticides in milk is a cause for concern and great strides are taken throughout the dairy industry to assure the purity of milk. The presence of multiple pesticides in virtually all samples of milk raises new questions about the possible toxicological impacts of pesticide mixtures on an infant’s developing nervous and immune systems, as well as on reproductive organs.
The main objective of our work was to develop a simple, rapid, and reliable MNP-based fluorescent immunoassay (MNPs-FIA) for the individual and simultaneous determination of paraoxon and DDVP in full milk samples. The preparation and characterization of fluorescent antigen–BSA (bovine serum albumin) conjugates were investigated. The influence of milk cow type and animal species on immunoassay was studied.
Materials and methods
Reagents
For the preparation of cationized bovine serum albumin (cBSA), BSA, 1-ethyl-3-[3-dimethylaminopropyl] carbodiimide hydrochloride, and ethylenediamine, purchased from Sigma-Aldrich (Taufkirchen, Germany), were used. All reagents used for obtaining conjugates DDVP (2,2-dichlorovinyl dimethyl phosphate), paraoxon-ethyl (diethyl 4-nitrophenyl phosphate), formaldehyde, diethyl ether, hydrochloric acid, acetic acid, sodium chloride, ethyl acetate, glutaraldehyde, zinc powder, anhydrous sodium sulfate, dimethyl formamide, ATTO 620 NHS, Bradford reagent, and ethanol were purchased from Sigma-Aldrich (Taufkirchen, Germany). Commercial Abraxis OP/Carbamate Assay was purchased from Abraxis LLC (Warminster, PA, USA). Dimethylformamide, fluorescein 5(6)–isothiocyanate (FITC), isomer I, 98% were used to make the fluorescent conjugates and were purchased from Sigma-Aldrich (Taufkirchen, Germany). For the purification of conjugates, Sephadex G-25 from GE Healthcare (Sweden) was used. Buffers were prepared according to standard laboratory procedure. All solutions were prepared using deionized water (ELGA, Buckinghamshire, UK).
Instrumentation
The ultraviolet–visible spectra of hapten conjugates were recorded by Spectrophotometer 6900 (Jenway, England). Fourier transforms infrared spectra of hapten conjugates were measured by Fourier transform infrared spectrophotometer Tenzor 27-Bruker (Bruker Optik GmbH, Germany). The fluorescence intensity in immunoassay was detected by a Perkin Elmer LS 45 fluorescence spectrophotometer (USA).
Preparation of functionalized MNPs, polyclonal anti-paraoxon antibody and anti- DDVP antibody
The preparation and functionalization of MNPs with APTES ((3-aminopropyl)triethoxysilane) were carried out by methods described by Ivanova and Godjevargova (Citation2015). Immunization and purification of polyclonal antibodies (anti-paraoxon antibody and anti- DDVP antibody) were performed by methods described in our previous paper (Yaneva, Ivanov, & Godjevargova, Citation2017).
Immobilization of anti-paraoxon antibody and anti- DDVP antibody onto the MNPs
The immobilization of anti-paraoxon antibody and anti- DDVP antibody was carried out by covalent method. 5 mg of the MNPs functionalized with APTES were activated with glutardialdehyde. The nanoparticles were collected with a magnet, the solution was taken out, and the nanoparticles were resuspended in 1 mL glutardialdehyde solution (5% w/v) in 50 mM PBS pH 8 and incubated for 2 h at room temperature with orbital agitation. Then the nanoparticles were washed once with 50 mM PBS pH 8 and five more times with 10 mM PBS pH 7.4. The MNPs were resuspended in 1 mL 10 mM PBS pH 7.4 containing 1 mg/mL anti-paraoxon and anti- DDVP polyclonal antibodies, respectively, and incubated for 2 h at 37°C. After that the nanoparticles were washed three times with 10 mM PBS pH 7.4 and the free active NH2 groups were blocked by adding 10 mM PBS pH 7.4 containing 1% BSA and 0.05% Tween 20. Each complex of antibody nanoparticles were stirred for 1 h at room temperature, washed three times, and resuspended in the same buffer to a final concentration of 5 mg/mL.
Determination of the amount of immobilized antibody on MNPs
The efficiency of the coupling strategies was evaluated by analyzing the antibody concentration in the supernatant before and after the coupling by the Bradford method (Bradford, Citation1976). The amount of antibody bound to the MNPs (mg Ab) was calculated according to the following equation:
where mg Ab' is the amount of antibody (in mg) in the starting solution and mg Ab'' is the amount of the unbound antibody (in mg) in the supernatant.
Preparation of FITC-labeled DDVP–cBSA conjugate
This conjugate was prepared by three consequentive steps (see supporting material). The first and second steps were preparation of cBSA and connection of cBSA with DDVP by condensation with formaldehyde. The method of their preparation was described in our early paper (Yaneva et al., Citation2017). The third step was preparation of DDVP–cBSA–FITC conjugate.
Fluorescein 5(6)-isothiocyanate (FITC), as a fluorescent marker, was used. DDVP–cBSA conjugate (15 mg) was dissolved in deionized water (1.5 mL). Then 500 µL FITC (2 mg/mL in dimethylformamide) was added slowly and reaction mixture was incubated overnight at 4°C. The labeling was carried out in brown vial to prevent photo-degradation of the fluorescent compound. The obtained conjugate (DDVP–cBSA–FITC) was purified by gel filtration through Sephadex G-25 column (1.5 × 30 cm). The column was equilibrated with PBS (10 mM pH 7.4). The column flow rate was 1 mL/min. The fractions of 2.0 mL were collected. The fraction absorbance was measured using a Jenway 6900 UV/Vis spectrophotometer. Spectrophotometric and IR analyses were used to evaluate the composition of the conjugate. The resulting product was lyophilized and stored at −20°C. Conjugate-containing fractions were analyzed with the following equations:
Preparation of FITC-labeled paraoxon–BSA conjugate
This conjugate was prepared also by three consequently steps (see supporting material). The first and second steps were reduction of nitro groups of paraoxon to amino groups and connection of modified paraoxon with BSA by glutaraldehyde. The method of their preparation was described in our early paper (Yaneva et al., Citation2017). The third step was preparation of paraoxon–cBSA–FITC conjugate. The connection between paraoxon–cBSA and FITC was made following the same condition as DDVP–cBSA–FITC.
Preparation of ATTO 620 NHS-labeled paraoxon–BSA conjugate
Paraoxon–BSA conjugate (10 mg) was dissolved in 250 µL 0.1 M sodium bicarbonate buffer pH 8.3. 0.5 mg ATTO 620 NHS was dissolved in 50 µL dimethylformamide. ATTO 620 NHS was added to conjugate dripwise while stirring constantly and carefully. Then the solution was left 1 h on shaker at room temperature and then overnight at 4°C. After expiring of the incubation time the conjugate was purified from unreacted low molecular compounds by means of gel permeation chromatography on the Sephadex G-25 carrier in 10 mM phosphate-buffered saline, pH 7.4. The resulting product was lyophilized and stored at −20°C. The optimal F/P values are 3–10 for any particular IgG.
Determination of optimal concentration of immunoassay components
Four different concentrations of the DDVP–cBSA–FITC and paraoxon–BSA–FITC conjugates (1.5, 3, 7.5, and 12 µg/mL) were used. The concentration of each conjugate concentration was combined with four different concentrations of the antibody (anti-dichlorovos, anti-paraoxon, and the combination of them) – 0.125, 0.25, 0.375, and 0.5 mg of antibody–MNPs provides 0.0055, 0.011, 0.0165, and 0.022 mg of immobilized antibody, respectively. Each sample of antibody–MNPs, described earlier, was put into a microcentrifuge tube. Supernatant was discarded by magnetic separator and MNPs complex remained on the microcentrifuge tube sidewall. Then, 67 µL of the antigen (50 µg/mL) was added to the microcentrifuge tube containing MNPs complex and 135 μL of each conjugate concentrations, described earlier, were added to the samples. An incubation step was performed for 15 min at 37°C. The particles were collected with a magnet, the supernatant was taken out and the residual fluorescent intensity was measured by a Perkin Elmer LS 45 fluorescence spectrophotometer. The fluorescence signal of the supernatant was measured at 518 nm. Experimental signals were calculated as the difference between initial and residual fluorescence of conjugate in percentages. The optimal concentrations of DDVP–BSA–FITC, paraoxon–BSA–ATТO 620 conjugates and corresponding immobilized antibodies for simultaneous immunoassay were determined by the same way as at individual immunoassay.
Immunoassay for individual determination of DDVP and paraoxon
An estimated 75 µL of 5 mg/mL immobilized antibody (0.375 mg antibody–MNPs) on MNPs against DDVP or paraxon was transferred to tubes and collected by a magnet. The supernatant was pipetted, and 67 µL of corresponding antigen with different concentrations (0–500 ng/mL in cow’s, goat’s, sheep’s, pasteurized milk and buffer solution) were incubated for 15 min at 37°C. Then 135 μL of each conjugate concentration, paraoxon–BSA–FITC (22 µg/mL) and DDVP–cBSA–FITC (18 µg/mL) was added to corresponding MNPs–Ab and incubated for 15 min at 37°C. Both antigens and fluorescent conjugate compete for the corresponding immobilized antibody. After the incubation, the MNPs–Ab–pesticides were separated from the supernatant on the side wall of the microcentrifuge tube by a magnet. The clear supernatant containing unbound tracer was separated. The fluorescence signal of this supernatant was measured at 518 nm by a Perkin Elmer LS 45 fluorescence spectrophotometer. Experimental signals were normalized using the following expression:
where B is the signal (intensity of fluorescence) measured in the presence of the increasing analyte concentrations; Bx is the signal in the absence of pesticides; and B0 is the signal of the initial conjugate solution. Competition curves were obtained by plotting normalized signal, % against the logarithm of analyte concentration. Sigmoidal curves were fitted to a four-parameter logistic equation, from which IC50 values (half maximal inhibitory concentration of the antibody–antigen binding) were determined. Each point represents the mean of five experiments, CV = 4–8%.
Immunoassay for simultaneous determination of DDVP and paraoxon
For simultaneous determination of DDVP and paraoxon in the same sample, the conjugate between paraoxon–BSA and fluorescein dye ATTO 620 NHS (620/643 nm) with carboxylic groups was prepared by carbodiimide method. 75 µL of 5 mg/mL of immobilized antibody on MNPs against DDVP (0.375 mg antibody–MNPs) and 75 µL of 5 mg/mL of immobilized antibody on MNPs against paroxon (0.375 mg antibody–MNPs) were transferred to tubes and collected by a magnet. The supernatant was pipetted, and 67 µL of paraoxon and 67 µL of DDVP solutions with different concentrations (0–500 ng/mL in cow’s, goat’s, sheep’s, pasteurized milk and buffer solution) were incubated for 15 min at 37°C. Then a 135 μL of two conjugates, paraoxon–BSA–ATTO 620 (22 µg/mL) and DDVP–cBSA–FITC (18 µg/mL), were added to the mixture and incubated for 15 min at 37°C. Both antigens and fluorescent conjugate competed for the corresponding immobilized antibody. After the incubation, the MNPs–Ab–pesticides were separated from the supernatant on the side wall of the microcentrifuge tube by a magnet. The clear supernatant containing unbound tracer was separated. The fluorescence signal of this supernatant was measured at 518 nm and 643 nm by a Perkin Elmer LS 45 fluorescence spectrophotometer. Experimental signals were normalized using the expression like individual analysis. Each point represents the mean of five experiments, CV = 4–8%.
Preparation of buffer and milk standards
The working standards containing 1.0, 2.0, 5.0, 10.0, 25.0, 50.0, 100.0, 150.0, 200.0, 250.0, 300.0, 400.0, and 500.0 ng/mL pesticides (DDVP and paraoxon) in cow’s milk, buffer (10 mM PBS, pH 7.4), pasteurized, goat’s, and sheep’s milk were prepared from a 1 mg/mL stock solution. Fresh full-cream cow’s, sheep’s, and goat’s milk (pesticide-free milk, measured by commercial Abraxis OP/Carbamate Assay (Abraxis LLC, Warminster, PA, USA) were used for the preparation of standard milk solutions and concentrations of pesticides were the same as in the buffer standard solutions. Three types of raw milk and pasteurized cow milk (1% fat) were employed for immunoassay validation and to obtain the pesticide calibration curves. It should be noted that it was not necessary to perform any sample pretreatment to the milk samples.
Recovery from milk by immunofluorescence analysis
The recovery of pesticides in cow’s milk was evaluated. Fresh full-cream cow’s milk (pesticide-free), measured by commercial Abraxis OP/Carbamate Assay (Abraxis LLC, Warminster, PA, USA), was used for the preparation of standard milk solutions. Samples free from pesticides were fortified with mixture of DDVP and paraoxon in a ratio of 1:1 with concentration 50.0, 100.0, and 200.0 ng/mL. This recovery was used for the estimation of concentration of the pesticides in samples by indirect competitive immunofluorescence analysis.
Results and discussion
Preparation of FITC-labeled DDVP–cBSA conjugate
DDVP–cBSA–FITC conjugate was prepared in three steps, as described in Materials and Methods section. DDVP does not have a functional group to react with proteins by the usual coupling methods. It is necessary to synthesize cationized protein for DDVP–protein coupling. Cationized protein was prepared according to the method of Muckerheide, Apple, Pesce, and Michael (Citation1987). BSA was treated with 1-ethyl-3-[3-dimethylaminopropyl] carbodiimide hydrochloride and ethylenediamine, consequently. The DDVP–cBSA conjugate was prepared by condensing with formaldehyde, using a modified method (Feng et al., Citation2009). In the third step (i.e. preparation of DDVP–cBSA–FITC conjugate), DDVP–cBSA was labeled with fluorescent dye (FITC). The isothiocyanate groups of FITC were bounded with amino groups of the DDVP–cBSA conjugate. Purification of the DDVP–cBSA–FITC conjugate was carried out by gel filtration chromatography. UV–Vis spectra of the DDVP–cBSA–FITC conjugate was measured and compared to UV–Vis spectra of the DDVP–cBSA and FITC, for conjugation proving. The results are shown in .
It is obvious that DDVP–cBSA absorbs at a maximum wavelength of 270 nm, while FITC absorbs at 490 nm, according to the obtained results. The maximum absorbing wavelength in DDVP–cBSA –FITC spectrum () is slightly blue-shifted from 490 to 487 nm compared with the unconjugated FITC, due to the interaction of DDVP–cBSA and FITC. Preparation of DDVP–cBSA–FITC conjugate was proved also by IR analysis (see supporting material). The optimal ratio of fluorescent dye to protein (F/P values) was 5.5 for investigated conjugate.
Preparation of FITC-labeled paraoxon–BSA conjugate
The conjugate was prepared also by three consequentive steps. The first and second steps were paraoxon nitro group reduction to amino groups and modified paraoxon–BSA connection by glutaraldehyde. The third step was preparation of paraoxon–BSA–FITC conjugate. UV–visible spectrophotometric method was used to confirm the paraoxon–BSA–FITC conjugate formation. In , ultraviolet spectra of paraoxon–BSA conjugate, FITC, and paraoxon–BSA–FITC conjugate are presented. It is obvious that the paraoxon–BSA conjugate has two absorption peaks. The first peak is at 275 nm and the second peak at 385 nm. FITC absorbed at a maximum wavelength of 490 nm. The obtained paraoxon–BSA–FITC conjugate has two absorption peaks, which corresponds to the peaks of the paraoxon–BSA and FITC, but they were offset. The first peak is shifted from 275 to 271 nm due to the reaction of paraoxon–BSA with FITC. The second peak is shifted from 490 to 489 nm, again due to the same interaction. Preparation of paraoxon–BSA–FITC conjugate was proved also by IR analysis (see supporting material). The optimal F/P value was 5.2 for investigated conjugate.
Preparation of ATTO 620-labeled paraoxon–BSA conjugate
The paraoxon–BSA–ATTO 620 conjugate was prepared for simultaneous determination of paraoxon and DDVP in milk. UV–visible spectrophotometric method was used to confirm the formation of the paraoxon–BSA–ATTO 620 conjugate. In , ultraviolet spectra of ATTO 620 and paraoxon–BSA–ATTO 620 conjugate are presented. ATTO 620 absorbs at 620 nm. The obtained conjugate paraoxon–BSA–ATTO 620 has two absorption peaks, which correspond to the peaks of the paraoxon–BSA () and ATTO 620. The first peak at 332 nm is large and includes the characteristic peaks of paraoxon–BSA (275 and 385 nm). The second peak is at 618 nm, corresponding to a characteristic peak of ATTO 620. The shift of that peak is due to the reaction of paraoxon–BSA with ATTO 620. The optimal F/P value was 4.6 for the investigated conjugate.
Determination of optimal conditions for pesticide MNPs-FIA
The obtained conjugates were used as tracer in MNPs-FIA for the determination of DDVP and paraoxon. The other important component for immunoassay is immobilized antibodies. Polyclonal antibodies against DDVP and paraoxon were covalently coupled on MNPs. The MNPs (Fe3O4) were synthesized by a wet chemical method through the co-precipitation of Fe2+ and Fe3+ aqueous salt solutions. They were modified by APTES as the coating material to introduce amino groups (Smith & Chen, Citation2008; Wu, He, Chen, Tang, & Nie, Citation2007). The sizes of initial and functionalized MNPs were determined by IZON qNano particle analyzer (England), respectively, 182 and 292.5 nm. The nanoparticles’ size with immobilized antibody allows the possibility of handling the particles in suspension. The simple collection of magnetic particles by a permanent magnet allows separating bound and free fraction of the tracer. Other advantages of the magnetic beads compared to the conventional solid supports used in immunoassays are easy manipulation, low pressure drop, high mass transfer rate, good fluid–solid contact, and perspectives for system automation and miniaturization (Rad, Yavuz, Kocakulak, & Denizli, Citation2003; Solé, Merkoçi, & Alegret, Citation2001; Zhao & Shippy, Citation2004). The choice of the separation procedure is important to enhance the sensitivity in the heterogeneous immunoassays. Thus the development of a new MNPs-FIA method for the determination of pesticides in milk is very interesting.
Polyclonal antibodies against DDVP and paraoxon were covalently coupled through their amino groups to the functionalized MNPs with amino groups by random immobilization with glutaraldehyde. The efficiency of the coupling was evaluated using the Bradford method (Bradford, Citation1976). It was found that the amount of immobilized polyclonal anti-DDVP and anti-paraoxon antibodies on MNPs was 0.226 mg Ab per mg MNPs.
The individual immunoassays for the determination of paraoxon and DDVP were developed. For this purpose, the optimal concentrations of DDVP–cBSA–FITC and paraoxon–BSA–FITC conjugates and immobilized antibodies were determined. The best results for DDVP immunoassay were obtained at 18 μg/mL DDVP–cBSA–FITC conjugate and 0.375 mg antibody–MNPs (0.0165 mg immobilized antibody). The optimal concentrations of paraoxon–BSA–FITC conjugate and immobilized antibody were obtained – 22 μg/mL paraoxon–BSA–FITC conjugate and 0.375 mg antibody–MNPs (0.0165 mg immobilized antibody).
The simultaneous immunoassays for the determination of paraoxon and DDVP were developed. For this purpose, the paraoxon–BSA–ATTO 620 conjugate was prepared. The optimal conditions for the simultaneous determination of DDVP and paraoxon were also determined. It was found that the optimal concentrations of conjugates (DDPV–BSA–FITC and paraoxon–BSA–ATTO 620) and immobilized antibodies were the same as at individual immunoassay.
Calibration curves of individual and simultaneous determination of pesticide in cow’s milk and buffer by MNPs-FIA
The antigens (DDVP and paraoxon) are small molecules that only have one site for binding to the antibodies; so the immunoassay design was restricted to a competitive format. Thus, free antigen (pesticide) in the sample and constant known amount of fluorescent-labeled antigen were incubated with a limited amount of immobilized antibody on MNPs. So, the competition for the antibody binding sites was established. The MNPs-FIA was performed in 10 mM PBS pH 7.4 and in milk sample. After magnetic separation of the MNPs on the tube side wall, fluorescent intensity of the free unbound tracer in supernatant was measured. The fluorescence signal was proportional to the concentration of the antigen in the sample. After that, the inhibition curve characteristic of the immunoassay was plotted.
The calibration curves of individual immunoassay of DDVP, individual immunoassay of paraoxon, and simultaneous immunoassay of DDVP and paraoxon in raw cow’s milk were investigated by MNPs-FIA at determined optimal conjugate and antibody concentrations (). The assay sensitivity is usually expressed in terms of its lower detection limit (LOD). The LOD is the lowest concentration of analyte giving a response statistically different from that observed in the absence of analyte. It is calculated as 95% from Bx – the fluorescence intensity of bound conjugate to the immunosorbent in the absence of pesticides. shows that in raw cow’s milk the IC50 value of the individual paraoxon assay was 60 ng/mL, and detection limit was 1.9 ng/mL (5% inhibition). The linear working range of the assay was between 2 and 300 ng/mL (). The results for individual DDVP determination are similar to the first one. The IC50 value of the individual DDVP assay was 70 ng/mL and detection limit was 4.75 ng/mL. The linear working range of this assay was between 5 and 300 ng/mL. The linear working ranges of simultaneous assay of paraoxon and DDVP were with higher pesticide concentrations of 10–400 ng/mL. The IC50 values of the paraoxon and DDVP in simultaneous assay were higher than individual ones, 100 and 120 ng/mL, respectively. The detection limit of simultaneous paraoxon assay was 7.5 ng/mL and DDVP assays – 8.0 ng/mL. It was proved that the change was not due to the presence of the both pesticides in the sample. It was confirmed by investigation of the specificity of the antibodies by indirect competitive ELISA. It was found that the cross-reactivity of anti-DDVP antibody with DDVP was 100% and had no cross-reactivity with paraoxon-ethyl. The anti-paraoxon antibody provided cross-reactivity of 100% with paraoxon-ethyl, and no cross-reactivity with DDVP. The calibration relationships are presented in . High correlation coefficients were achieved in cow’s samples using the MNPs-FIA (0.96–0.98).
Figure 4. MNPs-FIA curves for inhibition paraoxon (Ab1, ♦); DDVP (Ab2, ▪); paraoxon (Ab1+Ab2, ▴), DDVP (Ab1+Ab2, x) in cow’s milk by indirect competitive immunoassay.
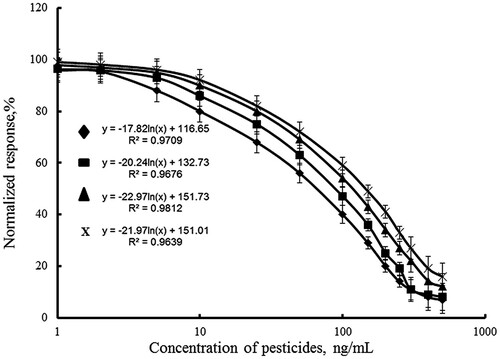
Table 1. Basic analytical characteristics of MNPs-FIA of paraoxon (Ab1), DDVP (Ab2), paraoxon (Ab1+Ab2), DDVP (Ab1+Ab2) in cow, pasteurized milk, and in buffer solution.
The MNPs-FIA was applied to evaluate pesticides in buffer solution and in pasteurized cow’s milk with low fat concentration – 1% ( and ). The obtained results were compared with the results in fresh raw milk. Series of standard solutions with different pesticide concentrations were prepared in buffer solutions and pasteurized milk for the analytical performance of the proposed fluorescent immunoassay assessment. It was found that all curves in pasteurized milk with low fat concentration and in buffer have the shorter linear range and LOD compared to the same characteristics in cow’s milk. Obviously the milk matrix (fat, proteins, salts and est.) was affected on the sensitivity of pesticide analysis. The linear range of all curves in buffer solutions was the shortest and the sensitivity was the highest (). High correlation coefficients were achieved in buffer samples and in pasteurized cow’s milk samples using the MNPs-FIA (0.94–0.99).
Figure 5. MNPs-FIA curves for inhibition paraoxon (Ab1, ♦); DDVP (Ab2, ▪); paraoxon (Ab1+Ab2, ▴), DDVP (Ab1+Ab2, x) in buffer solution by indirect competitive immunoassay.
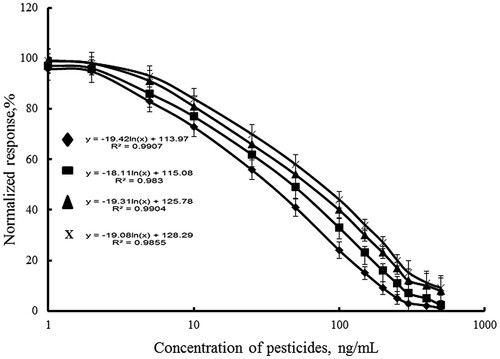
The results from the paraoxon and DDVP immunoassay were compared to the values obtained by similar ELISA protocols in the literature (). The reported paraoxon and DDVP immunoassay provided higher sensitivity with linearity to lower concentrations compared to the previously reported procedures.
Table 2. Comparison of organophosphorus pesticide immunoassay with literature protocols.
Influence of milk type on immunoassay
The influence of different animal species milk on the bioassay was studied. The calibration curves of individual immunoassay of paraoxon, individual immunoassay of DDVP, and simultaneous immunoassay of paraoxon and DDVP were investigated in raw goat’s milk. The change in IC50 values of the four immunoassays are negligible – 50, 75, 90, and 110 ng/mL, respectively compared to the results in cow’s milk (). The linear ranges of curves of individual and simultaneous immunoassays in goat’s milk were the same as the linear ranges of curves in cow’s milk. The sensitivity of the pesticide assay in goat’s milk is closely to the sensitivity of cow’s milk immunoassays.
Table 3. Basic analytical characteristics of MNPs-FIA of paraoxon (Ab1), DDVP (Ab2), paraoxon (Ab1+Ab2), DDVP (Ab1+Ab2) in cow’s, goat’s, and sheep’s milk.
The linear ranges in sheep’s milk are different () from the results in two other types of milks. The IC50 values of the four immunoassays in sheep milk are higher – 65 (individual, paraoxon), 80 (individual, DDVP), 110 (simultaneous, paraoxon), and 140 ng/mL (simultaneous, DDVP) (). The linear working ranges of individual DDVP and paraoxon immunoassays were shorter from 10 to 300 ng/mL, and of simultaneous assays from 25 to 400 ng/mL. The main reason for the obtained results was the higher fat concentration (7.8%) in sheep’s milk compared to the fat content in cow’s milk (3.8%) and goat’s milk (3.5%). The pesticide limit detections of MNPs-FIA in sheep’s milk were higher than the limit detections in cow’s milk. The high fat content in sheep’s milk (7.8%) suppressed the sensitivity of pesticide immunoassay. High correlation coefficients were achieved in goat’s and sheep’s milk samples using the MNPs-FIA (0.96–0.99).
It was found that the results of individual and simultaneous determination of pesticides in all used types of milk () are comparable. Obviously, the simultaneous immunoassay is more effective than individual, because it is possible to measure two types of pesticides in the same sample at the same time. Therefore, the obtained MNPs-FIA for individual and simultaneous determination of pesticides is simple and convenient to use, allowing direct use of undiluted sample; at the same time this assay maintains high sensitivity with a wide linear range of pesticides.
Recovery test
The cow’s milk samples were spiked with mixture of DDVP and paraoxon (1:1) and detected by the developed simultaneous immunoassay to evaluate the accuracy and precision of the optimized MNPs-FIA performance. The milk samples were free of pesticides, measured by commercial Abraxis OP/Carbamate Assay (Abraxis LLC, Warminster, PA, USA). The recovery, standard deviation (SD), and coefficient of variation (CV) values are shown in . As indicated in , the recovery of all the samples approached ranged from 97% to 100%.
Table 4. Recovery simultaneously determination of DDVP and paraoxon (N = 3).
Conclusion
A sensitive and rapid MNPs-FIA for individual and simultaneous determination of pesticides was developed. The assay can be used to detect paraoxon and DDVP in untreated milk. Recoveries of paraoxon and DDVP from spiked milk samples were acceptable. The MNPs-immunoassay, with the optimal assay conditions, demonstrated to be able to detect multiple pesticides accurately even in whole milk samples. The capacity of the MNPs-immunoassay technique could be further expanded to higher throughput targets simultaneously. The system could also become fully automatic if dealing with a larger volume of routine testing. In addition, the details of assay development from this study will help others optimizing similar multiplex detection using MNPs-immunoassays for different purposes in the future.
Suppording_material.docx
Download MS Word (9.4 MB)Acknowledgements
The authors gratefully acknowledge to the Bulgarian National Science Fund and to the Bulgarian Ministry of Education for their financial support and encouragement of the scientific research work in state universities.
Disclosure statement
No potential conflict of interest was reported by the authors.
Additional information
Notes on contributors
Marina Yaneva
Marina Yaneva is PhD Student in department of Biotechnology at “Prof. Dr Asen Zlatarov” University, Bourgas, Bulgaria. The dissertation title is “Immunofluorescence analysis for pesticides in milk”. The immunoanalysis are based on polyclonal antibody immobilized on magnetic nanoparticles.
Yavor Ivanov
Yavor Ivanov is assistant professor in department of Biotechnology at “Prof. Dr Asen Zlatarov” University, Bourgas, Bulgaria. He works in the field of immunoassays, immobilized enzymes, immobilized antibody and application for food safety.
Nikola Todorov
Nikola Todorov is assistant professor in department of Ecology at “Prof. Dr Asen Zlatarov” University, Bourgas, Bulgaria. He works in the field UV-Vis spectrometry, IR spectrometry and ecological environmental.
Tzonka Godjevargova
Tzonka Godjevargova is Professor in department of Biotechnology at “Prof. Dr Asen Zlatarov” University, Bourgas, Bulgaria. She is Head of the Biotechnology Department. She works in the field of the immunoassays, enzyme biosensors, nanoparticle producing and food safety.
References
- Biagini, E., Murphy, M., Sammons, L., Smith, P., Striley, F., & MacKenzie, A. (2002). Development of multiplexed fluorescence microbead covalent assays (FMCAs) for pesticide biomonitoring. Bulletin of Environmental Contamination and Toxicology, 68, 470–477. doi: https://doi.org/10.1007/s001280278
- Biagini, E., Sammons, L., Smith, P., MacKenzie, A., Striley, A., Semenova, V., … Snawder, E. (2004). Comparison of a multiplexed fluorescent covalent microsphere immunoassay and an enzyme-linked immunosorbent assay for measurement of human immunoglobulin G antibodies to anthrax toxins. Clinical and Diagnostic Laboratory Immunology, 11, 50–55.
- Biagini, E., Schlottmann, A., Sammons, L., Smith, P., Snawder, C., Striley, A., … Weissman, N. (2003). Method for simultaneous measurement of antibodies to 23 pneumococcal capsular polysaccharides. Clinical and Diagnostic Laboratory Immunology, 10, 744–750.
- Bradford, M. (1976). A rapid and sensitive method for the quantitation of microgram quantities of protein utilizing the principle of protein-dye binding. Analytical Biochemistry, 72, 248–254. doi: https://doi.org/10.1016/0003-2697(76)90527-3
- Celik, I., Yilmaz, Z., & Turkoglu, V. (2009). Hematotoxic and hepatotoxic effects of dichlorvos at sublethal dosages in rats. Environmental Toxicology, 24(2), 128–132. doi: https://doi.org/10.1002/tox.20390
- Cengiz, M., Certel, M., & Göçmen, H. (2006). Residue contents of DDVP (dichlorvos) and diazinon applied on cucumbers grown in greenhouses and their reduction by duration of a pre-harvest interval and post-harvest culinary applications. Food Chemistry, 98, 127–135. doi: https://doi.org/10.1016/j.foodchem.2005.05.064
- Chen, G., Jin, M., Du, P., Zhang, C., Cui, X., Zhang, Y., … Zheng, L. (2017). A review of enhancers for chemiluminescence enzyme immunoassay. Food and Agricultural Immunology, 28, 315–327. doi: https://doi.org/10.1080/09540105.2016.1272550
- Darling, B., Laing, H., & Harkness, A. (1974). A survey of steroid in cow’s milk. Journal of Endocrinology, 62(2), 291–297. doi: https://doi.org/10.1677/joe.0.0620291
- Díaz-Cruz, M., de Alda, L., López, R., & Barcelo, D. (2003). Determination of estrogens and progestogens by mass spectrometric techniques (GC/MS, LC/MS and LC/MS/MS). Journal of Mass Spectrometry, 38(9), 917–923. doi: https://doi.org/10.1002/jms.529
- Feng, Q., Xu, Y., Zhou, Y., Lu, L., Chen, F., & Wang, X. (2010). Preparation of dichlorvos-protein complete antigen by mannich-type reaction. Journal of Molecular Structure, 977(1), 100–105. doi: https://doi.org/10.1016/j.molstruc.2010.05.020
- Feng, Y., Zhou, X., Zou, Q., Wang, X., Chen, S., & Gao, X. (2009). Preparation and characterization of bisphenol A-cationized bovine serum albumin. Journal of Immunological Methods, 340(2), 138–143. doi: https://doi.org/10.1016/j.jim.2008.10.011
- Fulton, J., McDade, L., Smith, L., Kienker, J., & Kettman, R. (1997). Advanced multiplexed analysis with the FlowMetrix system. Clinical Chemistry, 43, 1749–1756.
- Gillis, H., Gosling, P., Sreenanc, M., & Kanea, M. (2002). Development and validation of a biosensor-based immunoassay for progesterone in bovine milk. Journal of Immunological Methods, 267(2), 131–138. doi: https://doi.org/10.1016/S0022-1759(02)00166-7
- Ivanova, T., & Godjevargova, T. (2015). Sensitive progesterone determination using a magnetic particle-based enzyme-linked immunosorbent assay. Analytical Letters, 48(5), 843–860. doi: https://doi.org/10.1080/00032719.2014.963596
- Joel, I., Mukunzi, D., Chen, Y., Suryoprabowo, S., Liu, L., Kuang, H., & Hu, C. (2017). Development of a monoclonal antibody assay and a lateral flow strip test for the detection of paromomycin residues in food matrices. Food and Agricultural Immunology, 28, 355–373. doi: https://doi.org/10.1080/09540105.2016.1272551
- Limei, P., Chifang, P., Yiping, Y., Xianggang, Y., Chuanlai, X., Yuan, Y., & Ma, W. (2008). CN Patent No. 101334409 A. Methyl paraoxon enzyme linked immunosorbent assay method (CN101334409).
- Li, Y., Sun, Y., Lei, H., Wang, H., Shen, Y., Yang, J., … Sun, X. (2017). Immunochemical techniques for multianalyte analysis of chemical residues in food and the environment: A review. TrAC Trends in Analytical Chemistry, 88, 25–40. doi: https://doi.org/10.1016/j.trac.2016.12.010
- Li, K., Zhu, Y., Yin, G., Penq, F., Chen, W., Liu, Q., … Xu, L. (2009). Development of an indirect enzyme-linked immunosorbent assay for the organophosphorus pesticide paraoxon-methyl. Immunological Investigations, 38(6), 510–525. doi: https://doi.org/10.1080/08820130902803689
- Muckerheide, A., Apple, J., Pesce, J., & Michael, G. (1987). Cationization of protein antigens. I. Alteration of immunogenic properties. Journal of Immunology, 138, 833–837.
- Rad, Y., Yavuz, H., Kocakulak, M., & Denizli, A. (2003). Bilirubin removal from human plasma with albumin immobilised magnetic poly(2-hydroxyethyl methacrylate) beads. Macromolecular Bioscience, 3, 471–476. doi: https://doi.org/10.1002/mabi.200350018
- Roex, M., Keijzers, R., & van Gestel, M. (2003). Acetylcholinesterase inhibition and increased food consumption rate in the zebrafish, Danio rerio, after chronic exposure to parathion. Aquatic Toxicology, 64, 451–460. doi: https://doi.org/10.1016/S0166-445X(03)00100-0
- Smith, E. A., & Chen, W. (2008). How to prevent the loss of surface functionality derived from aminosilanes. Langmuir, 24, 12405–12409. doi: https://doi.org/10.1021/la802234x
- Solé, S., Merkoçi, A., & Alegret, S. (2001). New materials for electrochemical sensing III. Beads. TrAC Trends in Analytical Chemistry, 20, 102–110. doi: https://doi.org/10.1016/S0165-9936(00)00059-5
- Tang, J., Zhang, M., Cheng, G., Li, A., & Lu, Y. (2008). Development of IC-ELISA for detection of organophosphorus pesticides in water. Journal of Environmental Science and Health, Part B, 43(8), 707–712. doi: https://doi.org/10.1080/03601230802388827
- Tudorache, M., Co, M., Lifgren, H., & Emneus, J. (2005). Ultrasensitive magnetic particle-based immuno supported liquid membrane assay (m-ISLMA). Analytical Chemistry, 77(22), 7156–7162. doi: https://doi.org/10.1021/ac050978k
- Tudorache, M., Zdrojewska, I. A., & Emneus, J. (2006). Evaluation of progesterone content in saliva using magnetic particle-based immuno supported liquid membrane assay (m-ISLMA). Biosensors and Bioelectronics, 22(2), 241–246. doi: https://doi.org/10.1016/j.bios.2006.01.002
- Vignali, D. A. (2000). Multiplexed particle-based flow cytometric assays. Journal of Immunological Methods, 243, 243–255. doi: https://doi.org/10.1016/S0022-1759(00)00238-6
- Watanabe, E., Miyake, S., & Yogo, Y. (2013). Review of enzyme-linked immunosorbent assays (ELISAs) for analyses of neonicotinoid insecticides in agro-environments. Journal of Agricultural and Food Chemistry, 61, 12459–12472. doi: https://doi.org/10.1021/jf403801h
- Wu, W., He, Q., Chen, H., Tang, J., & Nie, L. (2007). Sonochemical synthesis, structure and magnetic properties of air-stable Fe3O4/Au nanoparticles. Nanotechnology, 18, 145609–145613. doi: https://doi.org/10.1088/0957-4484/18/14/145609
- Xu, Z., Deng, H., Deng, X., & Sun, Y-M. (2012). Monitoring of organophosphorus pesticides in vegetables using monoclonal antibody-based direct competitive ELISA followed by HPLC–MS/MS. Food Chemistry, 131, 1569–1576. doi: https://doi.org/10.1016/j.foodchem.2011.10.020
- Xu, Z., Shen, Y., Zheng, W., Beier, R., Xie, G., Donq, J. … Sun, J. (2010). Broad-Specificity immunoassay for O,O-diethyl organophosphorus pesticides: Application of molecular modeling to improve assay sensitivity and study antibody recognition. Analytical Chemistry, 82, 9314–9321. doi: https://doi.org/10.1021/ac1018414
- Yamaura, M., Camilo, R. L., Sampaio, L. C., Macedo, M. A., Nakamura, M., & Toma, H. E. (2004). Preparation and characterization of (3-aminopropyl)triethoxysilane-coated magnetite nanoparticle. Journal of Magnetism and Magnetic Materials, 279, 210–217. doi: https://doi.org/10.1016/j.jmmm.2004.01.094
- Yaneva, M., Ivanov, Y., & Godjevargova, T. (2017). Preparation of polyclonal antibodies with application for an organophosphorus pesticide immunoassay. Analytical Letters, 50(8), 1307–1324. doi: https://doi.org/10.1080/00032719.2016.1221417
- Zhao, X., & Shippy, A. (2004). Competitive immunoassay for microliter protein samples with magnetic beads and near-infrared fluorescence detection. Analytical Chemistry, 76(7), 1871–1876. doi: https://doi.org/10.1021/ac035198v