ABSTRACT
Vitamin C (VC) is an essential micronutrient and plays important roles in various biological processes including immune responses. Vaccination can stimulate immune responses effectively for prevention of virual infection. Adequate VC intake is required for the immune system to function efficiently. In this study, we evaluated the biological function of VC on immune response to inactivated foot-and-mouth disease (FMD) vaccine. The C57BL/6 mice were injected intraperitoneally with different dose of VC daily following immunization with inactivated FMD vaccine. The results showed high dose of VC supplementation significantly increased IgG level. Similarly, high dose of VC supplementation enhanced Th2 and Th17 cellular immune responses and also increased MHCII expression on dendritic cells. Taken together, these data indicate that high dose of VC supplementation can modulate Th2 and Th17 cellular immune responses to inactivated FMD vaccine and manifest the necessity for effective vaccination.
Introduction
Vitamin C (VC) is an essential nutrient required for normal physiological functions in the body. It is necessary for the health of humans, animals and cells. As an antioxidant, VC protects the body from the deleterious effects of free radicals, pollutants and toxins. VC deficiency is often associated with anaemia, infections and bleeding gums (Li & Schellhorn, Citation2007). Most animal species have the ability to synthesize VC, but humans and other primates cannot (Nishikimi, Fukuyama, Minoshima, Shimizu, & Yagi, Citation1994). The pig can synthesize VC, but clearly the body's principal need for VC is as an antioxidant, hydroxylation processes and hormone secretion. Feeding megadose of VC to young pigs increases urinary excretion of ascorbic acid. VC has positive effects on immune cells and responses which have been investigated in infection and immunity (Bergman et al., Citation2004; Chambial, Dwivedi, Shukla, John, & Sharma, Citation2013; Tewary & Patra, Citation2008). VC can enter mouse T cells as dehydroascorbic acid in vitro, but it had no effect on T-cell proliferation or cytokine secretion (Maeng et al., Citation2009). VC-treated murine bone marrow-derived dendritic cells (DCs) preferentially drived differentiation of T cells into T helper 1 (Th1) cells by increasing interleukin (IL-12) expression, demonstrating an effect of VC on DCs (Jeong et al., Citation2011). In vitro exposure of B cells to VC slightly decreased isotype switching to IgG1 and increased isotype switching to IgG2a, but VC had no effect on proliferation or co-stimulator expression in B cells (Jeong et al., Citation2011). VC dose-dependently inhibited the lipopolysaccharide-induced number of monocytes producing IL-6 and tumour necrosis factor-α (TNF-α, 20 mM VC) in a human whole blood assay (Härtel, Strunk, Bucsky, & Schultz, Citation2004). VC has a positive effect on immune responses in animals and humans. When guinea pigs were fed a VC-deficient diet and immunized with chicken erythrocytes in Freund's complete adjuvant, only cell-mediated toxicity was less than that in pair- or ad libitum-fed controls. However, the VC-deficient diet did not affect spleen weight, white blood counts or antibody levels in guinea pigs (Anthony, Kurahara, & Taylor, Citation1979). A high dose of VC (3 g ascorbate daily) enhanced neutrophil motility in response to chemotactic stimulus of endotoxin-activated autologous serum in normal adult volunteers, while no alteration was observed at lower doses (2 g ascorbate daily) (Anderson, Oosthuizen, Maritz, Theron, & Van Rensburg, Citation1980). Guinea pigs receiving 100 or 280 mg/day VC through their diet during immunization enhanced humoral antibody production to bovine serum albumin (BSA), indicating an effect of VC on humoral immunity (Feigen et al., Citation1982). High doses of VC (100 mg/kg body weight) increased the efficiency of immunization with a human cell culture rabies vaccine in guinea pigs (Banic, Citation1982). Megadose VC administration before or after the appearance of cold and flu symptoms relieved and prevented the symptoms in test subjects compared with the control group (Gorton & Jarvis, Citation1999). VC increased production of interferon-α/β (IFN) and induced in vivo antiviral immune responses at the early stage of influenza virus infection in L-gulono-γ-lactone oxidase knockout (Gulo-/-) mice (Kim et al., Citation2013). These findings indicated different effects of VC on immune cells and responses according to the dosage, route of administration and animal species. By comparing the administration route, VC supplementation in the diet is not as accurate as injection in terms of the dose. Hence, VC injection was applied in this study for accurate doses of VC supplementation.
Foot-and-mouth disease (FMD) is caused by FMD virus (FMDV) and affects cloven-hoofed animals in the farming industry. Vaccination is an effective strategy to control infectious diseases including FMD. The traditional vaccine to prevent FMDV infection is an inactivated FMD vaccine that is often formulated with oil as the adjuvant (O’Hagan, MacKichan, & Singh, Citation2001). Mice are often employed as an animal model instead of natural hosts for FMDV virus and vaccine studies. The pathogenesis of FMDV in mice depends on virus strain, the route of virus challenge and the animal strain (Grubman, Citation2005). When mice were injected intraperitoneally with an FMDV, viral replication resulted in viremia and an increase of neutralizing antibody in the serum (Fernández et al., Citation1986). However, the adult C57BL/6 mice have been shown no sensitivity to natural infection because most FMDV do not affect the mortality of adult mice, except for a few that have been studied (Habiela et al., Citation2014). The FMDV vaccine immunized C57BL/6 mice were challenged with a series of challenge viruses FMDV types O, A, and Asia1 and the pathogenesis of each virus was evaluated in the mouse model. The result showed that serotype O, A and Asia1 FMDVs that were non-pathogenic in C57BL/6 mice gained pathogenicity after the repeated passages in mice and cells. This study revealed that FMD vaccine evaluation should be carried out using mouse-adapted challenge viruses as an effective test of experimental or commercial vaccines (Lee et al., Citation2016). Although the inactivated FMD vaccine has been successfully used for many years, animals must be revaccinated to maintain immune responses because of a decline in efficacy. A lack of nutrition may be one reason for the vaccine efficacy. It has been reported that VC affects immune responses to the inactivated FMD vaccine. Brown Swiss cattle aged about one year were immunized with a trivalent FMD vaccine (type A, O and Asia 1) and received VA, VD3 and VE, VC alone and in combination. VA, VD3 and VE were administered intramuscularly and VC intravenously at a dose of 20 mg/kg body weight. The antibody titres for type A and Asia 1 were superior in VC alone and combination groups, and the antibody titre for type O was significantly higher in the combination group than in other groups. These results suggest that VA, VD3 and VE plus VC together with injection of the trivalent FMD vaccine provided stronger immunity (Kizil & Gul, Citation2008). In this study, we investigated the effect of VC administration on immune responses to an inactivated FMD vaccine.
Materials and methods
Animals
Female C57BL/6 mice were provided by Animal Institute of Chinese Medical Academy (Beijing, China). The mice were 6–8 weeks old and housed under a specific pathogen-free facility (SPF) condition. All mice were kept 7 days before treatment. Animals were maintained in 48 × 14 × 15 cm cages and 6 mice were in one cage. The C57BL/6 mice were feeded with pathogen-free food (Animal Institute of Chinese Medical Academy, Beijing, China) and water under a 12 h light-cycle condition (07:00–19:00 light). Health status of mice was observed daily during the treatment. Adverse events did not happen. Each mouse was injected intraperitoneally with saline, 1 mg (20 IU) VC/day or 10 mg (200 IU) VC/day respectively according to the mice groups. Before serum from orbital blood were collected for IgG test (n = 3), the mice were anesthetized with pentobarbital sodium (Cat. No. P3761, Sigma) under dosing 40–45 mg/kg body weight. After the mice were sacrificed by exsanguination through cutting cervical artery under anaesthesia, the spleens were taken out. Each test was repeated three times and three mice were used for each experiment. All animal protocols [SKLAB-2016-01] were approved by the Animal Welfare Committee of China Agricultural University.
Reagents
VC was from Sinopharm RongSheng pharmaceutical company (Approved by H41023603). All monoclonal antibodies (mAbs) used in this study were purchased from eBioscience. The chemically inactivated FMD vaccine and 146S antigen were obtained from Lanzhou Institute of Veterinary Medicine (Lanzhou, China).
Animal groups and immunization
Mice were randomly divided into five groups (n = 9). The mice were injected intraperitoneally with 1 mg VC or 10 mg VC daily. The mice were injected with saline as control group (Control). Day 7 and day 21 after 1 mg or 10 mg VC treatment, the mice were immunized with 100 µl inactivated FMD vaccine intramuscularly in test groups (Vaccine/1 mg VC or Vaccine/10 mg VC). The mice were immunized with vaccine without VC treatment as control group of immunization (Vaccine). All experiments were repeated three times. The injection sites had no erythema and oedema and the mice were healthy after immunizations.
Blood routine examination
The mice were treated with VC or FMD vaccine. Day 7 after vaccination, the whole blood of mice (n = 5) were collected for blood routine examination (Veterinary Teaching Hospital, China Agricultural University, Beijing, China).
ELISA
Day 7 after the second immunization, serum was collected for IgG level test by ELISA. The 96-well ELISA plates were coated with 146S (5 µg/ml) overnight and blocked with 5% milk for 1 h. The serially dilutions of mice serum were added to the plates. As secondary antibodies, 100 µl of horseradish peroxidase-labeled goat anti-mouse IgG (Sigma, St. Louis) were incubated at 37°C for 1 h. The antibodies were diluted at 1:3000 for IgG. After four washes with phosphate buffered solution-tween 20 (PBST), 10 mg of TMB tablet (Sigma, St. Louis) were dissolved in 0.025 M phosphate-citrate buffer and added to each well. The reaction was stopped by addition of 0.2 M H2SO4. Optical density values at 450/620 nm were checked with a plate reader (Magellan, Tecan Austria GmbH). Antibody titre values were assigned as the highest dilution at which the OD was 2 SDs higher than the OD produced by the serum of naive mice at the same dilution. The mean of titres was calculated using log conversion for each dilution (Kang et al., Citation2005).
T cell proliferation
Three mice from each group were sacrificed and single splenocyte suspensions were prepared on day 4 after the second immunization. T cell proliferation was performed as described previously (Gao et al., Citation2013; Kang et al., Citation2005). The splenocytes were cultured in 96-well cell culture plates and stimulated with 146S as specific stimulator, anti-CD3 mAb as positive control and BSA as nonspecific antigen control for 72 h. MTT solutions (20μl, 5 mg/ml) was added and incubated with the cells in each well for 4 h. The samples were centrifuged at 1500 rpm for 10 min and the supernatants were removed. DMSO was added to each well and incubated for 10 min to dissolve the crystal substance. The plates were read at 492 nm by a microplate reader. The stimulation indexes (SI) were determined by mean OD value of triplicate antigen containing wells divided by that of triplicate wells not containing antigen.
Flow cytometry analysis
Total splenocytes from each group were prepared and total splenocytes in per spleen from all groups were counted. For surface staining, the samples were blocked with anti-CD16/32 mAb and stained with anti-mouse CD4-PE/ CD8-FITC/B220-APC mAbs for flow cytometry analysis. For intracellular staining, the splenocytes in all groups were prepared and performed as described previously (Gao et al., Citation2013; Kang et al., Citation2005). The splenocytes were stimulated with Cell Stimulation Cocktail (eBioscience) and treated with Protein Transport Inhibitor Cocktail (eBioscience) for cytokines produced in CD4 T cells while the splenocytes were stimulated with FMDV CD8 T cell specific peptide for cytokine produced in CD8 T cells for 8 h. The splenocytes were blocked with anti-CD16/32 mAb and stained with anti-CD4-FITC mAb for intracellularly staining of Th cells or stained with anti-CD8-FITC mAb for intracellularly staining of cytotoxic T (Tc) cells. The samples were treated with Fixation/Permeabilization Diluent (eBioscience) and stained with anti-mouse IFN-γ, IL-4 or IL-17 expression in Th cells and IFN-γ in Tc cells. The samples were analysed with a FACScalibur and the Cell Quest Pro Software (BD Bioscience).
Statistical analysis
Pairwise differences were analysed by the two-sided Student’s t-test. ANOVA and the Bonferroni test were used for multi-group analysis. Differences were considered significant if p < .05 and very significant if p < .01.
Results
High dose of VC improved the peripheral blood status
The blood routine is an important method to value the healthy condition. Many blood indexes can change after infection or vaccination. As shown in , the mice treated with Vaccine/10 mg VC showed high red blood count (RBC), haemoglobin (HGB) and haematocrit (HCT) significantly compared with other groups. The result indicated that high dose of VC improved the peripheral blood condition and is good for animal health.
Table 1. RBC, HGB and HCT in whole blood of mice.
High dose of VC increased the percentage and numbers of CD4 T cells in immunized mice
As a nutrient element, VC supplement can modify function of immune system and immune cells. To evaluate the effect of VC on immune cells, the spleens of mice from all groups were taken out. The total splenocytes in each spleen from all groups were counted, but there were no differences of splenocytes among all groups (Data not shown). The samples were stained with anti-mouse CD4-PE/CD8-FITC/B220-APC mAbs for flow cytometry analysis. The CD4+ T, CD8+ T or B220+ B cells were counted relatively to total splenocytes. As shown in , 10 mg VC significantly increased the percentage and number of CD4+ T cells compared with that in other groups (p < .05), but there were no differences of CD8+ T cells among all groups. The percentage of B cell was significantly increased in Vaccine/10 mg VC group than that in other groups, but there were no differences of B cell numbers among all groups.
Figure 1. High dose of VC supplementation increased the percentage and number of CD4T cells in spleens of immunized mice. Notes: (A) 4 days after the second immunization, the splenocytes were prepared and stained with anti-mouse CD4-PE/CD8-FITC/B220-APC mAbs for flow cytometry analysis. (B) The statistical result of CD4+ T, CD8+ T or B220+ B cells in total splenocytes was shown. Shown in each panel is 1 of at least 3 experiments with similar results. Bar, mean and SD from 3 independent experiments, each using at least three mice per group (n = 3); *p < .05.
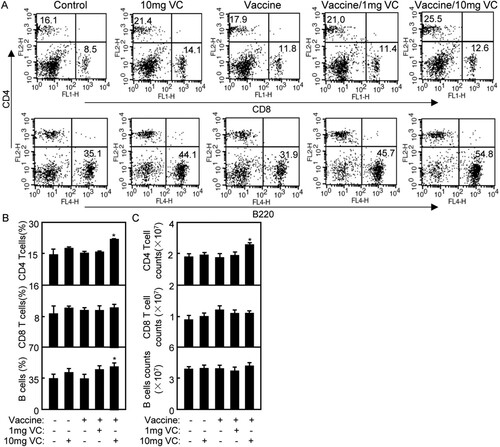
High dose of VC increased IgG level in immunized mice
To evaluate the effect of VC on humoral immunity of inactivated FMD vaccine, serum of immunized mice was collected for ELISA on day seven after the second immunization. The titres of total IgG was defined as the highest dilution that gave a ratio above 2:1 for the test serum and the negative control. As shown in , the IgG level of Vaccine/10 mg VC group was higher than that in Vaccine and Vaccine/1 mg VC groups (p < .01). There were no differences between Vaccine/1 mg VC and Vaccine groups which were consistent with the increased percentage of B cells.
Figure 2. High dose of VC supplementation increased IgG level of immunized mice. Notes: The C57BL/6 mice were treated daily by intraperitoneal injection with VC. On day 7 and day 21, the mice were immunized with vaccine respectively. The mice were injected with saline, VC or vaccine alone as control groups. Seven days after the second immunization, serum of immunized mice was collected for ELISA. Bar, mean and SD from 3 independent experiments, each using at least three mice per group (n = 3); **p < .01.
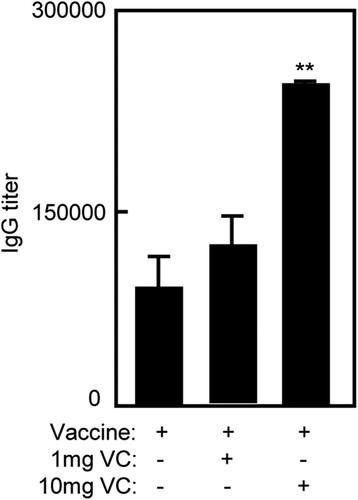
High dose of VC enhanced Th2 and Th17 cellular immune responses in immunized mice
The cellular immune response plays an important role in preventing FMDV infection. The splenocytes were prepared for T cell proliferation to analyse T cell responses to vaccination. The splenocytes were stimulated with 146S as specific stimulator, anti-mouse CD3 mAb as positive control and BSA as irrelevant antigen control. The results were shown as SI, calculated as the mean reading of triplicate wells stimulated with an antigen, divided by the mean reading of triplicate wells stimulated with medium. As shown in , there were no differences of SI value among Vaccine/10 mg VC, Vaccine/1 mg VC and Vaccine groups.
Figure 3. VC had no effect on T cell proliferative response of immunized mice. Notes: The C57BL/6 mice were treated daily by intraperitoneal injection with VC. On day 7 and day 21, the mice were immunized with vaccine respectively. The mice were injected with saline, VC or vaccine alone as control groups. Four days after the second immunization, the splenocytes were prepared. T cell proliferation was analysed by the MTT method and expressed as SI. The anti-CD3 mAb was used as positive control while BSA was used as negative control. Bar, mean and SD from 3 independent experiments, each using at least three mice per group (n = 3).
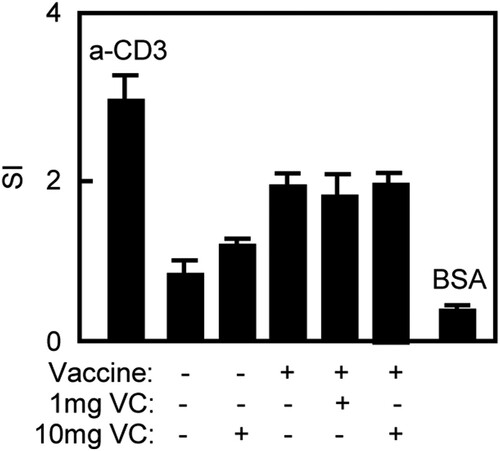
Cytokines produced by T cells play a central role in the modulation of immune responses. To analyse the effect of VC on cytokine profiles induced by vaccine, the splenocytes of immunized mice were prepared and intracellularly stained for flow cytometry analysis. CD4+ or CD8+ T cells were gated for the cytokine analysis. As shown in , the percentage of Th2 cytokine (CD4+IL-4+) was significantly increased in Vaccine/10 mg VC group compared with that in other groups (p < .05) which was consistent with the result of IgG. Furthermore, the percentage of Th17 (CD4+IL-17+) was significantly increased in Vaccine/10 mg VC group compared with that in other groups (p < .05). However, there were no differences of Th1 cytokine (CD4+IFN-γ+) and Tc1 cytokines (CD8+IFN-γ+) among all groups.
Figure 4. High dose of VC supplementation enhanced Th2 and Th17 cellular immune responses of immunized mice. Notes: The C57BL/6 mice were treated daily by intraperitoneal injection with VC. On day 7 and day 21, the mice were immunized with vaccine respectively. The mice were injected with saline, VC or vaccine alone as control groups. Four days after the second immunization, the splenocytes were prepared and analysed by flow cytometry. (A) The splenocytes were stained with anti-mouse CD4-FITC/IFN-γ-PE, anti-mouse CD4-FITC/ IL-4-PE, anti-mouse CD4-FITC/ IL-17-PE or anti-mouse CD8-FITC/ IFN-γ-PE mAbs. The CD4+IFN-γ+ cells were counted relatively to total CD4+ T cells for Th1; the CD4+IL-4+ cells were counted relatively to total CD4+ T cells for Th2; the CD4+IL-17+ cells were counted relatively to total CD4+ T cells for Th17cell analysis. The CD8+ cells were gated and CD8+IFN-γ+ cells were counted relatively to total CD8+ T cells for Tc1cell analysis. (B) The statistical results of Th1, Th2, Th17 and Tc1were shown. Shown in each panel is 1 of at least 3 experiments with similar results. Bar, mean and SD from 3 independent experiments, each using at least three mice per group (n = 3); *p < .05.
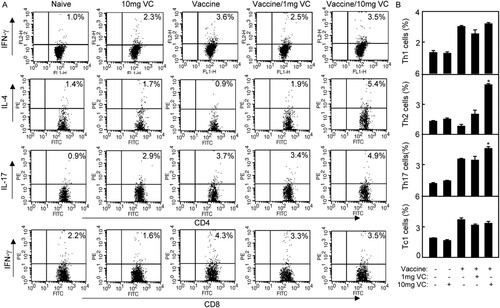
High dose of VC enhanced antigen presentation of DCs in immunized mice
The expression of MHC molecules and co-stimulatory molecules plays an essential role in the maturation of DCs which interact with T cells for T cell activation. As shown in , the expression of MHCII on DCs was increased significantly in Vaccine/10 mg VC group compared with that in other groups (p < .05). There were differences of CD40, CD80 and CD86 expression on DCs among all groups. The results suggested that 10 mg VC supplementation could improve the antigen presenting of DCs of mice in vaccination with inactivated FMD vaccine.
Figure 5. High dose of VC supplementation improved antigen presenting of DCs. Notes: The C57BL/6 mice were treated daily by intraperitoneal injection with VC. On day 7 and day 21, the mice were immunized with vaccine respectively. The mice were injected with saline, VC or vaccine alone as control groups. (A) On day 3 after the second immunization, the splenocytes of immunized mice were prepared and stained with anti-CD11c-FITC, anti-MHCII-PE, CD40-PE, CD80-PE or CD86-PE. The samples were analysed by flow cytometry. The CD11c+MHCII+ cells, CD11c+CD40+ cells, CD11c+CD80+ cells or CD11c+CD86+ cells were counted relatively to total CD11c+ cells. (B) The statistical results of MHCII+, CD40+, CD80+ or CD86+ DCs were shown. Shown in each panel is 1 of at least 3 experiments with similar results. Summary of CD11c+MHCII+ cells, CD11c+CD40+ cells, CD11c+CD80+ cells or CD11c+CD86+ cells in immunized mice. Bar, mean and SD from 3 independent experiments, each using at least three mice per group (n = 3). *p < .05.
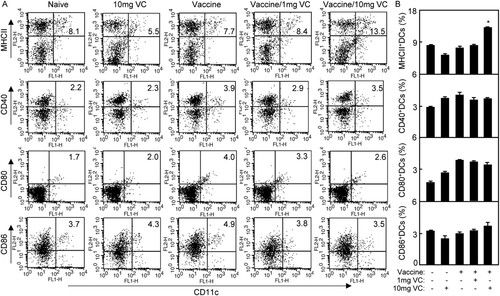
Discussion and conclusion
In this study, we revealed the effects of VC supplementation on immune responses to an inactivated FMD vaccine in C57BL/6 mice. The results showed that C57BL/6 mice treated with 10 mg VC had improved antigen presentation of DCs, which elicited antigen-specific humoral and Th2/Th17 cellular immune responses. These results suggest that a high dose of VC modulates immune responses to the inactivated FMD vaccine, and VC supplementation is imperative for effective vaccination.
Oxygen free radicals exert a noxious effect on cell functions. An increasing number of reports have drawn attention to the reactive oxygen species (ROS) as inducers of potential cell damage (Kehrer & Klotz, Citation2015; Maltepe & Saugstad, Citation2009). The antioxidants exerting a protective effect on human cells (Maeng et al., Citation2009). VC has a central role in controlling oxidative reactions in the cell and its antioxidant role involves trapping ROS and making them less reactive. Ascorbic acid reacts rapidly with the tocopherol radical, reducing it back to its native form (Nagaoka, Kakiuchi, Ohara, & Mukai, Citation2007). As an antioxidant, VC is required for normal physiological functions of the body. VC is easily oxidized and destroyed. Humans and other primates cannot synthesize VC (Nishikimi et al., Citation1994), so VC supplementation is necessary for animal and human health. VC deficiency often causes low immunity and certain diseases (Li & Schellhorn, Citation2007). The supplementation with VC could prevent severe liver damage induced by inflammation (Bae et al., Citation2013). The blood routine is an important method to value the healthy condition and blood indexes can change after infection or vaccination. VC enhanced neutrophil motility in response to endotoxin-activated autologous serum in normal adult volunteers (Anderson et al., Citation1980). The 21-day old broilers with VC supplementation increased HCT, HGB and RBC (Yang et al., Citation2014). During summer season, Holstein growing-calves were supplemented with VC and some blood parameters including RBC, HGB, HCT, basophil, eosinophil and eosinophil were positively influenced in calves fed with VC (Kim et al., Citation2012). Children suffering from sickle cell anaemia with VC supplementation significantly increased packed cell volume, HGB concentration and per cent foetal HGB (Jaja, Ikotun, Gbenebitse, & Temiye, Citation2002). In this study, the mice treated with Vaccine/10 mg VC improved RBC, HGB and HCT () which indicated that high dose of VC is necessary for animal health.
VC affects the functions of immune cells including peripheral white blood cells (Bergman et al., Citation2004) and T cells (Maeng et al., Citation2009). It has positive effects on polymorphonuclear cells, B cells and DCs, but inhibits cytokine production of monocytes from human whole blood. VC has no effect on T-cell proliferation or cytokine secretion (Maeng et al., Citation2009). The number of T cell was decreased in the spleen of VC-insufficient (Gulo-/-) mice (Bae et al., Citation2013). VC has no effect on proliferation or co-stimulator expression in B cells (Jeong et al., Citation2011; Woo et al., Citation2010). In this study, 10 mg/mouse VC supplementation via intraperitoneal injection significantly increased CD4 T cells and B cell in mice immunized with the inactivated FMD vaccine, whereas 1 mg/mouse VC supplementation did not. Furthermore, 1 and 10 mg VC/day did not influence CD8 T cells of immunized mice () and FMDV-specific T cell proliferative response (). These results suggest that a high dose of VC improves the immune functions of CD4 T and B cells in immunized mice.
VC affects the functions of B cells and IgG production (Feigen et al., Citation1982; Woo et al., Citation2010). T addition of VC to B cells in vitro slightly decreases isotype switching to IgG1 and increases isotype switching to IgG2a (Woo et al., Citation2010, #9). Guinea pigs with dietary VC during immunization showed enhanced humoral antibody production to BSA which indicate the effect of VC on humoral immunity (Feigen et al., Citation1982). In guinea pigs, a VC-deficient diet weakened cell-mediated toxicity after immunization with chicken erythrocytes in Freund’s complete adjuvant (Anthony et al., Citation1979).Specific antibody responses are important in defense against FMD (McCullough et al., Citation1992; Meloen, Rowlands, & Brown, Citation1979). Protection against FMDV infection is considered to be closely related to antibody levels (Davies, Citation2002). High FMDV-specific IgG level is related to the protection of swine challenged with FMDV. Here, we demonstrated that the FMD vaccine in combination with a high dose of VC (10 mg/day) significantly promoted IgG antibody levels compared with FMD vaccination alone () which was consistent with the increased B cells percentage in Vaccine/10 mg VC group. These results indicated that co-administration of the FMD vaccine and a high dose of VC may enhance protection against FMDV infection through stimulating B cells and IgG antibody production. Other immune cells such as plasma and T follicular helper cells may be affected and will be checked in the future.
The effect of VC on immune responses has been investigated in humans and guinea pigs. A total of 252 subjects aged from 18 to 30 years were included in a test group, while 463 subjects ranging in age from 18 to 32 years were included in a control group for a clinical study. Subjects in the test group, who reported symptoms of flu, were treated with hourly doses of 1000 mg VC for the first 6 h and then three times daily thereafter. The results showed that megadose VC therapy helped to prevent and therapeutically treat the symptoms of cold and flu (Gorton & Jarvis, Citation1999). VC enhanced neutrophil motility in response to chemotactic stimulus of endotoxin-activated autologous serum in normal adult volunteers after ingestion of VC daily (Anderson et al., Citation1980). Previous studies have shown that both VC affect cytokine production. Healthy adults supplemented with VC and VE showed increased production of IL-1β and TNF-α by their peripheral blood mononuclear cell (Jeng et al., Citation1996). VC levels exert an early effect on immune homeostasis via reactive oxygen intermediates dependent expression of interleukin genes, since the transcription factor NF-kB is sensitive to ROI and regulates the expression of interleukin genes (Schwager & Schulze, Citation1998). VC showed in vivo antiviral immune responses at the early time of infection, especially against influenza virus, through increased production of IFN-α/β (Kim et al., Citation2013). VC-treated murine bone marrow-derived DCs preferentially drived Th1 differentiation by increased IL-12 expression (Jeong et al., Citation2011). In this study, the high dose of VC improved Th2/Th17 cellular immune responses to FMDV vaccine () and enhanced antigen presentation by DCs ().
Mice model is employed for measuring the immune responses induced by vaccines and the pathogenesis of virus challenge before testing them in host animals (Lee et al., Citation2016). There were different pathogenesis of virus infection with different animal strain and various vaccines. It is crucial to continue performing mice model to prove and develop evaluation approaches. In FMD endemic countries or countries at risk of FMD outbreak, swift evaluation of FMD vaccines against existing or potential field viruses is necessary to control FMD. Recently, C57BL/6 mice were shown sensitivity to artificial FMDV infection and only a few FMDV could cause 100% fatality in the mice (Habiela et al., Citation2014). A comparison study on mice and cattle immunized with the same FMD vaccine have shown that it is possible to relate the antibody levels of vaccinated mice with both the antibody and protective responses of vaccinated cattle. This study demonstrated that mice model appears to be an inexpensive and rapid alternative for testing FMD vaccines in cattle (Dus Santos et al., Citation2000). In this study, the antibody level in VC treated mice increased () which indicated VC adequate supplementation in cattle might enhance humoral response of FMD vaccination. However, the cattle or pig experiments need to be checked in future.
In summary, our study revealed the immunological enhancement of megadose VC supplementation for inactivated FMD vaccination. We demonstrated that high dose of VC increase antibody levels and enhance Th2 and Th17 cellular responses to the inactivated FMD vaccine. Our findings suggest VC can improve the efficacy of vaccination.
Acknowledgments
The funding agencies had no role in study design, data collection and analysis, decision to publish or preparation of the manuscript.
Disclosure statement
No potential conflict of interest was reported by the authors.
ORCID
Youmin Kang http://orcid.org/0000-0003-2597-6117
Additional information
Funding
References
- Anderson, R., Oosthuizen, R., Maritz, R., Theron, A., & Van Rensburg, A. J. (1980). The effects of increasing weekly doses of ascorbate on certain cellular and humoral immune functions in normal volunteers. The American Journal of Clinical Nutrition, 33, 71–76. doi: https://doi.org/10.1093/ajcn/33.1.71
- Anthony, L. E., Kurahara, C. G., & Taylor, K. B. (1979). Cell-mediated cytotoxicity and humoral immune response in ascorbic acid-deficient Guinea pigs. The American Journal of Clinical Nutrition, 32, 1691–1699. doi: https://doi.org/10.1093/ajcn/32.8.1691
- Bae, S., Cho, C. H., Kim, H., Kim, Y., Kim, H. R., & Hwang, Y. I. (2013). In vivo consequence of Vitamin C insufficiency in liver injury: Vitamin C ameliorates T-cell-mediated acute liver injury in Gulo(−/−) mice. Antioxidants & Redox Signaling, 19, 2040–2053. doi: https://doi.org/10.1089/ars.2012.4756
- Banic, S. (1982). Immunostimulation by vitamin C. International Journal for Vitamin and Nutrition Research. Supplement, 23, 49–52.
- Bergman, M., Salman, H., Djaldetti, M., Fish, L., Punsky, I., & Bessler, H. (2004). In vitro immune response of human peripheral blood cells to Vitamins C and E. The Journal of Nutritional Biochemistry, 15, 45–50. doi: https://doi.org/10.1016/j.jnutbio.2003.10.001
- Chambial, S., Dwivedi, S., Shukla, K. K., John, P. J., & Sharma, P. (2013). Vitamin C in disease prevention and cure: An overview. Indian Journal of Clinical Biochemistry, 28, 314–328. doi: https://doi.org/10.1007/s12291-013-0375-3
- Davies, G. (2002). Foot and mouth disease. Research in Veterinary Science, 73, 195–199. doi: https://doi.org/10.1016/S0034-5288(02)00105-4
- Dus Santos, M., et al. (2000). A comparison of methods for measuring the antibody response in mice and cattle following vaccination against foot and mouth disease. Veterinary Research Communications, 24, 261–273. doi: https://doi.org/10.1023/A:1006450900739
- Feigen, G. A., Smith, B. H., Dix, C. E., Flynn, C. J., Peterson, N. S., & Rosenberg, L. T. (1982). Enhancement of antibody production and protection against systemic anaphylaxis by large doses of Vitamin C. Research Communications in Chemical Pathology and Pharmacology, 38, 313–333.
- Fernández, F. M., Borca, M. V., Sadir, A. M., Fondevila, N., Mayo, J., & Schudel, A. A. (1986). Foot-and-mouth disease virus (FMDV) experimental infection: Susceptibility and immune response of adult mice. Veterinary Microbiology, 12, 15–24. doi: https://doi.org/10.1016/0378-1135(86)90037-4
- Gao, W., Sun, Y., Chen, S., Zhang, J., Kang, J., & Wang, Y. (2013). Mushroom lectin enhanced immunogenicity of HBV DNA vaccine in C57BL/6 and HBsAg-transgenic mice. Vaccine, 31, 2273–2280. doi: https://doi.org/10.1016/j.vaccine.2013.02.062
- Gorton, H. C., & Jarvis, K. (1999). The effectiveness of vitamin C in preventing and relieving the symptoms of virus-induced respiratory infections. Journal of Manipulative and Physiological Therapeutics, 22, 530–533. doi: https://doi.org/10.1016/S0161-4754(99)70005-9
- Grubman, M. J. (2005). Development of novel strategies to control foot-and-mouth disease: Marker vaccines and antivirals. Biologicals, 33, 227–234. doi: https://doi.org/10.1016/j.biologicals.2005.08.009
- Habiela, M., Seago, J., Perez-Martin, E., Waters, R., Windsor, M., & Salguero, F. J. (2014). Laboratory animal models to study foot-and-mouth disease: A review with emphasis on natural and vaccine-induced immunity. Journal of General Virology, 95, 2329–2345. doi: https://doi.org/10.1099/vir.0.068270-0
- Härtel, C., Strunk, T., Bucsky, P., & Schultz, C. (2004). Effects of vitamin C on intracytoplasmic cytokine production in human whole blood monocytes and lymphocytes. Cytokine, 27, 101–106. doi: https://doi.org/10.1016/j.cyto.2004.02.004
- Jaja, S. I., Ikotun, A. R., Gbenebitse, S., & Temiye, E. O. (2002). Blood pressure, hematologic and erythrocyte fragility changes in children suffering from sickle cell Anemia following ascorbic acid supplementation. Journal of Tropical Pediatrics, 48, 366–370. doi: https://doi.org/10.1093/tropej/48.6.366
- Jeng, K. C., Yang, C. S., Siu, W. Y., Tsai, Y. S., Liao, W. J., & Kuo, J. S. (1996). Supplementation with vitamins C and E enhances cytokine production by peripheral blood mononuclear cells in healthy adults. The American Journal of Clinical Nutrition, 64, 960–965. doi: https://doi.org/10.1093/ajcn/64.6.960
- Jeong, Y.-J., Hong, S. W., Kim, J. H., Jin, D. H., Kang, J. S., Lee, W. J., & Hwang, Y. I. (2011). Vitamin C-treated murine bone marrow-derived dendritic cells preferentially drive naïve T cells into Th1 cells by increased IL-12 secretions. Cellular Immunology, 266, 192–199. doi: https://doi.org/10.1016/j.cellimm.2010.10.005
- Kang, Y., Jin, H., Zheng, G., Xie, Q., Yin, J., & Yu, Y. (2005). The adjuvant effect of levamisole on killed viral vaccines. Vaccine, 23, 5543–5550. doi: https://doi.org/10.1016/j.vaccine.2005.07.017
- Kehrer, J. P., & Klotz, L.-O. (2015). Free radicals and related reactive species as mediators of tissue injury and disease: Implications for health. Critical Reviews in Toxicology, 45, 765–798. doi: https://doi.org/10.3109/10408444.2015.1074159
- Kim, Y., Kim, H., Bae, S., Choi, J., Lim, S. Y., & Lee, N. (2013). Vitamin C is an essential factor on the anti-viral immune responses through the production of interferon-α/β at the initial stage of influenza A virus (H3N2) infection. Immune Network, 13, 70–74. doi: https://doi.org/10.4110/in.2013.13.2.70
- Kim, J.-H., Mamuad, L. L., Yang, C. J., Kim, S. H., Ha, J. K., & Lee, W. S. (2012). Hemato-biochemical and cortisol profile of Holstein growing-calves supplemented with vitamin C during summer season. Asian-Australasian Journal of Animal Sciences, 25, 361–368. doi: https://doi.org/10.5713/ajas.2011.11438
- Kizil, O., & Gul, Y. (2008). Effects of vitamin AD3E and C on immune responses of cattle to trivalent foot and mouth disease vaccine. Revue de Médecine Vétérinaire, 159, 599–602.
- Lee, S.-Y., Ko, M. K., Lee, K. N., Choi , J. H., You, S. H., & Pyo, H. M. (2016). Application of mouse model for effective evaluation of foot-and-mouth disease vaccine. Vaccine, 34, 3731–3737. doi: https://doi.org/10.1016/j.vaccine.2016.06.008
- Li, Y., & Schellhorn, H. E. (2007). New developments and novel therapeutic perspectives for vitamin C. The Journal of Nutrition, 137, 2171–2184. doi: https://doi.org/10.1093/jn/137.10.2171
- Maeng, H. G., Lim, H., Jeong, Y. J., Woo, A., Kang, J. S., Lee, W. J., & Hwang, Y. I. (2009). Vitamin C enters mouse T cells as dehydroascorbic acid in vitro and does not recapitulate in vivo vitamin C effects. Immunobiology, 214, 311–320. doi: https://doi.org/10.1016/j.imbio.2008.09.003
- Maltepe, E., & Saugstad, O. D. (2009). Oxygen in health and disease: Regulation of oxygen homeostasis-clinical implications. Pediatric Research, 65, 261–268. doi: https://doi.org/10.1203/PDR.0b013e31818fc83f
- McCullough, K. C., De Simone, F., Brocchi, E., Capucci, L., Crowther, J. R., & Kihm, U. (1992). Protective immune response against foot-and-mouth disease. Journal of Virology, 66, 1835–1840.
- Meloen, R. H., Rowlands, D. J., & Brown, F. (1979). Comparison of the antibodies elicited by the individual structural polypeptides of foot-and-mouth disease and polio viruses. Journal of General Virology, 45, 761–763. doi: https://doi.org/10.1099/0022-1317-45-3-761
- Nagaoka, S.-I., Kakiuchi, T., Ohara, K., & Mukai, K. (2007). Kinetics of the reaction by which natural vitamin E is regenerated by vitamin C. Chemistry and Physics of Lipids, 146, 26–32. doi: https://doi.org/10.1016/j.chemphyslip.2006.12.001
- Nishikimi, M., Fukuyama, R., Minoshima, S., Shimizu, N., & Yagi, K. (1994). Cloning and chromosomal mapping of the human nonfunctional gene for L-gulono-gamma-lactone oxidase, the enzyme for L-ascorbic acid biosynthesis missing in man. Journal of Biological Chemistry, 269, 13685–13688.
- O’Hagan, D., MacKichan, M., & Singh, M. (2001). Recent developments in adjuvants for vaccines against infectious diseases. Biomolecular Engineering, 18, 69–85. doi: https://doi.org/10.1016/S1389-0344(01)00101-0
- Schwager, J., & Schulze, J. (1998). Modulation of interleukin production by ascorbic acid. Veterinary Immunology and Immunopathology, 64, 45–57. doi: https://doi.org/10.1016/S0165-2427(98)00120-2
- Tewary, A., & Patra, B. C. (2008). Use of vitamin C as an immunostimulant. Effect on growth, nutritional quality, and immune response of labeo rohita (Ham.). Fish Physiology and Biochemistry, 34, 251–259. doi: https://doi.org/10.1007/s10695-007-9184-z
- Woo, A., Kim, J. H., Jeong, Y. J., Maeng, H. G., Lee, Y. T., & Kang, J. S. (2010). Vitamin C acts indirectly to modulate isotype switching in mouse B cells. Anatomy & Cell Biology, 43, 25–35. doi: https://doi.org/10.5115/acb.2010.43.1.25
- Yang, X., Luo, Y. H., Zeng, Q. F., Zhang, K. Y., Ding, X. M., Bai, S. P., & Wang, J. P. (2014). Effects of low ambient temperatures and dietary vitamin C supplement on growth performance, blood parameters, and antioxidant capacity of 21-day-old broilers. Poultry Science, 93, 898–905. doi: https://doi.org/10.3382/ps.2013-03438