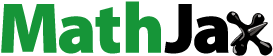
ABSTRACT
Herein, a simple and cost-effective chemiluminescence immunoassay combined with the magnetic particles (MPCLIA) is presented for the rapid detection and analysis of Aflatoxin B1, which is a potent carcinogen found in the environment. Several physicochemical factors that potentially influence the assay performance of the proposed method were investigated in detail, including concentrations of FITC-labelled antibody, AFB1-alkaline phosphatase and magnetic particles concentration. Under the optimized experiment condition, the method showed: (i) a low limit detection of 0.05 ng/mL; (ii) satisfactory recoveries in real grain and oil samples ranging from 78% to 109%, which was well within the requirement of residue analysis, and (iii) excellent sensitivity, wide dynamic range and easy to separate in the washing process, which was superior to enzyme-linked immunosorbent assay (ELISA) method. In addition, the device used is of low cost. Moreover, the MPCLIA results for the real samples were in good agreement with that obtained by the high-performance liquid chromatography. These results suggest that the proposed MPCLIA method has potential application for screening other toxic mycotoxins occur in the food industry or other applied fields.
Introduction
Aflatoxins, one of the most important mycotoxins in agriculture products, are the secondary metabolites of Aspergillus flavus, Aspergillus parasiticus, Aspergillus nomius, Aspergillus tamari and several other unknown species of Aspergillus, which are noted to be strong toxic, carcinogenic, mutagenic and immunosuppressive compounds. They could not only affect the safety of humans and animals, but also hinder the benign growth of economy (Battacone, Nudda, Palomba, Mazzette, & Pulina, Citation2009; Shim, Mun, Joung, Chung, & Kim, Citation2014; Vdovenko, Lu, Yu, & Sakharov, Citation2014). During the multifarious kinds of aflatoxin, Aflatoxin B1 (AFB1) seems to be commonly found and of poisonous molecules in the wide ranges of agriculture crops, including cereals, peanuts, cottonseed meal and corn, as well as the animal feeds (Goud et al., Citation2016; Yu, Anastasia, Marina, & Ivan, Citation2013). To minimize the potential exposure to the aflatoxins, many countries have built the maximum regulatory standard and have strict control over the residue level for AFB1. On the basis of the Food and Agriculture Organization (FAO) standard, the worldwide maximum tolerated levels of Aflatoxin B1 were reported to be in the range of 1–20 µg/kg in food, and 5–50 µg/kg in dietary cattle feed in 2003 (Shim, Kyeongyeol, Ofori, Chung Y, & Chung, Citation2012). Even though the usage of AFB1 is prohibited, it is frequently present in commercial animal foods and various edible food samples in levels higher than the maximum acceptable limit. To protect the legitimate rights and interests of consumers, using the sensitive and reliable analytical methods for the monitoring of AFB1 residue is of great importance.
To date, various analytic means have been widely established to detect the AFB1, including the high-performance liquid chromatography (HPLC) (Ghalia et al., Citation2009; Li, Chen, Cao, Yao, & Liu, Citation2011; Muhammad et al. Citation2016), mass spectroscopy (Bojidarka & Michael, Citation2014; Meritxell et al., Citation2004) and thin-layer chromatography (Cheraghali et al., Citation2007). However, most of the methods have huge drawbacks, such as they are sophisticated, time-consuming, laboratory based and unsuitable for the onsite monitoring of AFB1 analysis. As an alternative, immunoassays as rapid, low cost and high-throughput tests are becoming reliable analytical tools for the detection of AFB1 residues (Jiang et al., Citation2013; Liu, Hsu, Lu, & Yu, Citation2013; Liu, Lu, Liu, & Yu, Citation2016).
Up to now, many immunoassays have been successfully developed for detecting AFB1. However, the sensitivities of these methods are still not high enough for some applications. The chemiluminescence immunoassay (CLIA) is considered as one of the most sensitive classical immunoassays (Jin et al., Citation2009; Zhang et al., Citation2013). In addition, the light intensity of chemiluminescence (CL) reaches its maximum in 1–2 min after substrate addition, thus shortening the overall analytical procedure when compared with the conventional colorimetric assays. It is considered to be a promising analytical method to study in multi-domains, for example clinical diagnosis, immunoassays, pharmaceutical analysis and food analysis (Fan, Lau, & Lu, Citation2005; Fu et al., Citation2012; Kong, Xiong, Yue, & Fu, Citation2015; Liu et al., Citation2010; Qin & Lin, Citation2015; Zhang & Su, Citation2011). At the same time, to further improve the detection efficiency, magnetic particles (MPs) were introduced. MPs could possess remarkable merits, such as large surface area, magnetic susceptibility, low toxicity, low cost of synthesis, compatibility with biomaterials and easy to separate from the matrix.
In this paper, the chemiluminescence immunoassays based on the optimized MPs for the specific and sensitive determination of AFB1 were reported. The specificity and repeatability of the immunoassay was investigated by the cross-reactivity and the recycle experiments. In addition, the assay has been successfully utilized to the quantitative detection of AFB1 in the real grain and oil samples, which was well within the requirements of residue analysis. The MPCLIA method performance was evaluated in terms of precision and accuracy by comparison with HPLC.
Experimental design
Chemicals, buffers, calibrators and samples
N-Hydroxysuccinimide (NHS), Fluorescein isothiocyanate (FITC), 1-ethyl-3-(3-dimethylaminopropyl)-carbodimide hydrochloride (EDC), 2-(N-morpholino)ethanesulphonic acid (MES), bovine serum albumin (BSA), O-(carboxymethyl)hydroxylamine hemihydrochloride (CMO) and Sephadex G-25 were bought from Sigma-Aldrich. Alkaline phosphatase (ALP) was procured from BBI Enzymes. Ethyl acetate, N, N-dimethylformamide (DMF), sodium hydroxide (NaOH), hydrogen chloride (HCl), pyridine, petroleum ether, methanol, phenol and tris (hydroxymethyl) aminomethane hydrochloride were from Sinopharm Chemical Reagent Co., Ltd. Lumigen APS-5 was purchased from Lumigen, Inc. All the chemicals were used without further treatment.
The phosphate buffer (pH 7.4) contained 0.01 M KH2PO4 and 0.01 M Na2HPO4; Sodium bicarbonate buffer (pH 9.0) contained 0.05 M NaHCO3; The washing buffer (PBST): PBS with 0.5 mL/L Tween 20; AFB1 standard solution was prepared in the 0.05 M PBS solution (pH 7.4).
Aflatoxin B1 (AFB1), Aflatoxin B2 (AFB2), Vomitoxin (DON), Ochratoxin (OTA), Aflatoxin G1 (AFG1), Aflatoxin G2 (AFG2), Zearalenone (ZEN) and Aflatoxin M1 (AFM1) were purchased from Fermentek Ltd (Israel). The mouse monoclonal anti-AFB1 was provided by Kangyuan Techbio Biological Technology Co., Ltd (China). Quality Control sample was purchased from VICAM (America), which was used for the evaluation of the method performance.
The real grain and oil samples were collected and bought from the local supermarket. Highly purified deionized water was used throughout the study.
Instrumentation
The immunomagnetic particles were provided by Merck (Beijing, China), of which the diameter is 1.0 μm. The magnetic separator was provided from the Tianjin Baseline Chromtech Research Centre (Tianjin, China). The chemiluminescence analyser (BHP9507, Beijing Hamamatsu Photon Techniques Inc., China) was utilized to estimate the chemiluminescence (CL) signal.
Procedures
Preparation of FITC-labelled antibody
The FITC-labelled antibody was prepared according to the previous report (Adrian1, Liliana, & Hugo, Citation2011). 1 mg AFB1 antibody was dissolved in 0.2 mL of 1 mg/mL FITC carbonate buffer (0.2 M, pH 9.0). Then, the mixture was stirred overnight. After this process, the FITC-labelled antibody was purified by Sephadex G-25 column with Tris-HCl (0.1 M, pH 7.4).
Preparation of AFB1-ALP
The AFB1 hapten was prepared as per the following steps: 1 mg AFB1 was added to a 0.5 mL solution of 0.5 mL pyridine and 2 mg CMO. The mixture solution was gently refluxed at 70°C under constant magnetic stirring for 5 h. The pyridine was removed from the solution with 6 mL benzene. pH of the obtained sample was adjusted to 3 using 0.2 mol/L HCl, then the solution was extracted utilizing ethyl acetate and distilled water. The extraction was repeated three times. Finally, the product was dried in a vacuum at 50°C.
The AFB1-ALP was synthesized by the activated ester method (Yao, Wang, & Fang, Citation2017). Briefly, the AFB1 hapten was dissolved in 1 mL DMF, followed by adding 4 mg DCC and 4 mg NHS. The mixture was stirred at room temperature for 4 h. Then, the reaction solution was added to 7 mg ALP dissolved in 1 mL PBS by dropwise and the reaction continued 5 h at room temperature. The ultimate sample AFB1-ALP was purified by the Sephadex G-25 column.
Immuno-magnetic bead preparation
The anti-FITC antibody immobilized onto MPs was prepared according to a previous study (Zhang et al., Citation2014). Typically, 20 mg magnetic particles, 40 mg EDC and 30 mg NHS were mixed and stirred. Then, the particles were washed twice with the MES buffer (0.1 M, pH 6.0), further reacted with 6 mg anti-FITC antibody for 2 h. After the washing step, the residual uncombined sites on the particles were blocked with 20 mg BSA for several hours. Ultimately, the product was washed by the PBS several times and stored at 4°C.
Immunoassay procedure
The immunoassay procedures could be described in the following steps, as illustrated in . First, 60 μL of standard AFB1 solutions or samples was added into the assigned test tube. Secondly, 30 μL FITC-labelled AFB1 Ab (2 μg/mL) and 30 μL AFB1-ALP (2 μg/mL) were then added to the each tube stepwise and was incubated at 37°C for 15 min. When the “sandwich” reaction completed, after adding 60 μL magnetic particles coated with FITC antibody (3 mg/mL), the mixture was incubated for 5 min under gentle shaking at 37°C. In the washing process, the materials were dragged by the magnets in the test tubes rack for the full separation (2 min). After repeating three times of washing process, 200 μL of Lumigen APS-5 solution (substrate solution) was added and measured.
Determination of AFB1 in grain and oil samples
The practicability of this assay was analysed and validated by quantitative detection of AFB1 in the grain and oil samples. Grain samples for the precision analysis were prepared as follows: 5.0 g crushed grain using a Midea Food Mixer (BP252AG, Guangdong) was initially extracted with 25 mL 70% methanol solution after spiked with a known concentration of AFB1 and stirred for 3 min, followed by centrifugating at 5000×g for 10 min. Then the 100 μL upper liquid was diluted with 200 μL PBS and kept at 4°C before used. For the oil samples, 5.0 g oil was mixed well with 20.0 mL petroleum ether, and then adding 20 mL 50% methanol solution, the known different amounts of AFB1 were spiked into the above solution. The solution was centrifugated at 5000×g for 10 min at room temperature. The 200 μL filtrate liquid was diluted with 300 μL PBS and kept at 4°C before used. The blank grain and oil samples were prepared according to the above method but not mixed with the AFB1. The as-prepared grain and oil samples were further tested based on the MPCLIA procedure.
Method validation by HPLC
To validate the effectiveness of the developed MPCLIA, the samples were separately analysed using the MPCLIA and HPLC. For the HPLC analysis, the samples were detected according to the method of the announcement GB 5009.22-2016 of the National Heath and Family Planning Commission of the People’s Republic of China, China Food and Drug Administration. In brief, 5.0 g of homogenized sample was extracted with 0.5 g NaCl and 20 mL methanol: water (7:3) by the high-speed blender, centrifuging for 10 min under 6000 r/min. Take 4 mL of extract supernatant was diluted with 50 mL of PBS (pH 7.4). After extracting as described above, the supernatants were purified through the immunoaffinity column. The immunoaffinity column was conditioned with 10 mL of PBS, and 50 mL of the diluted filtrate was applied to the column at a flow rate of 2 mL/min. After the clean-up step the column was washed with 20 mL of water and air was forced through the column prior to eluating aflatoxins by applying 3 mL of methanol with a flow rate of 2 mL/min. The eluate was nearly dried by the N2 slowly blowing under 50°C. Then the eluate was diluted with 1 mL of the organic mobile phase. The organic phase was concentrated and dissolved with 2 mL of methanol and further confirmed by HPLC. The mobile phase consisted of acetonitrile: methanol: water 1:1:2, v/v/v) with a flow rate of 1.0 mL/min. The AFB1 was detected at the excitation and emission wavelength of 360 and 440 nm, respectively. The employed column was a C18 150*4.6 mm, 5 μm.
Results and discussion
Optimization of FITC-labelled AFB1 and AFB1-ALP
Theoretically, the appropriate concentration of immuno-reaction reagents is significant for the linear range and sensitivity of the immunoassay. By this, the varying concentrations of FITC-labelled AFB1 antibody and AFB1-ALP were investigated and evaluated in terms of the CL response ratio, which was counted on the basis of the CL signal of AFB1 to that of blank sample (RLU), as shown in . Combined consideration of lower non-specific binding and suitable CL values, the 2 mg/mL FITC-labelled AFB1 antibody and 2 mg/mL AFB1-ALP were used in the following experiments.
Optimization of MPs concentration
The amounts of MPs could also be dramatically significant for the experiment result. The redundant amounts of MPs could result in the lower sensitivity owing to the black colour of MPs; moreover, the deposition of MPs in the bottom could unavoidably absorb the light. Therefore, to optimize the concentration of magnetic beads, different concentrations of magnetic beads (2, 3, 4, 5, 6 mg/mL) were chosen in the study. shows that the CL intensity increased as the concentration was increasing from 2 to 3 mg/mL; however, the ratio did not change obviously while the concentration was increasing. Hence, 3 mg/mL was chosen in the following study.
Evaluation of the methodology
The performance of the proposed MPCLIA was further discussed to evaluate the ability to determine AFB1, according to the optimization records.
As demonstrated in , the signal ratio has a good linear relationship with the logarithm of AFB1 concentration in the range of 0.1–100 ng/mL, which indicated the wide linear range.
The sensitivity of the immunoassay was measured according to the function equation as given below:
(1)
(1) where SD is the standard deviation of 20 blank samples and K is the absolute value of slope for the linear calibration curve.
As a result, the sensitivity value of this method for AFB1 was less than 0.05 ng/mL, which conformed to the acceptable limit requirement. Compared with other typical methods, the results in this work demonstrate an excellent sensitivity for the detection of AFB1. Although the LOD was somewhat higher than those of some methods (Potentiometric immunosensor, Li, Lv, Lu, Lin, & Tang, Citation2016), it offered relative advantages of low cost, simpler operation procedure and good reproducibility, meanwhile, the detection time is short. The results are listed in .
Table 1 . Comparison of this method and other typical methods.
The specificity of the immunoassay was studied by CR experiments. The CR was estimated in terms of the percentage of the IC50 of AFB1 over the IC50 of interfering mycotoxins compounds structurally related to AFB1, including AFB1, AFB2, AFG1, AFG2, AFM1, ZEN, DON and OTA. The AFB1 concentration causing 50% inhibition (IC50) was applied to calculate the CR as follows:
(2)
(2)
The experimental results displayed that AFB1 did not cross-react with the structurally related mycotoxins compounds, containing CR lower than 15%, as illustrated in . Therefore, the MPCLIA assay utilized in this work was sufficiently favourable for the evaluation of AFB1 in the grain and oils samples.
Table 2. Cross-reactivity comparison of various related mycotoxins developed for determination of AFB1.
The accuracy and feasibility of the developed analytical method are studied by the recovery test. During the recovery experiment, samples should stand for at least 24 h before processing. Various known concentrations (3, 15 and 30 ng/mL) of AFB1 were added to the target grains and oils samples. The analytical recovery (%) rate for each group was calculated as follows:
(3)
(3)
These experiments were analysed and repeated five times using the MPCLIA method The final results listed in showed that the acceptable and favourable recoveries were ranging from 78% to 109%; these data were within the requirement of residue analysis and in good agreement with the previously reported literature (Zhang et al., Citation2014), revealing that the developed method could be reliable and useful as a quantitative tool for AFB1 in real sample analysis.
Table 3. Determination of AFB1 spiked in the grain and oils.
To determine the precision of the method, the two samples were repeatedly detected for 10 times and calculated on a single day, the results of which are given in . The coefficient of variation (CV) for measuring the concentration of 1 and 4 ng/mL in the sample was 9.7% and 6.3%, respectively. Both the CV values were less than 10.0%. Such reproducibility was highly acceptable and in favour of the MPCLIA assay (Xu et al., Citation2016; Zeng et al., Citation2015).
Table 4. Coefficient of variation of AFB1 from spiked samples.
To further evaluate the developed detection method for AFB1, HPLC was selected for the comparative study with the MPCLIA immunoassay. Therefore, different samples with the unknown levels of AFB1 from grains were simultaneously analysed by HPLC and MPCLIA immunoassay. The results obtained by the two detection methods were fitted an excellent linear relationship, which displayed the good correlation between MPCLIA and HPLC, as shown in . Moreover, quality control was used for the evaluation of the method performance, ranging from 4.55 to 8.45 ng/mL. The detection value for MPCLIA was 5.86 ng/mL while the detection value for HPLC was 6.75 ng/mL. All of these indicated that the sensitivity, accuracy and precision of our established method could satisfy the requirements of trace AFB1 determination in aquatic grains.
Conclusions
In summary, an appealing magnetic particle-based chemiluminescence immunoassay was developed and successfully detected AFB1 residue in agriculture samples. Under the optimum conditions, the LOD of the MPCLIA immunoassay was significantly improved compared to mostly already reported immunoassays. Meanwhile, the method has been exploited to the quantitative determination of AFB1 in the real samples, which was with satisfactory recoveries ranging from 78% to 109%, well within the requirements of residue analysis for AFB1. Furthermore, a good correlation was obtained between the MPCLIA immunoassay and HPLC results for the real samples. Hence, such magnetic particle-based chemiluminescence immunoassays could be used for the quantitative detection of other mycotoxins in food products, which may open the door to improve quality control of the food safety domain.
Disclosure statement
No potential conflict of interest was reported by the authors.
Additional information
Funding
References
- Adrian1, H. M., Liliana, R. J., & Hugo, G. (2011). Assessment of aflatoxin B1 binding to Lactobacillus reuteri by microscopy and fluorescence techniques. Food Biotechnology, 25, 140–150. doi: https://doi.org/10.1080/08905436.2011.576561
- Battacone, G., Nudda, A., Palomba, M., Mazzette, A., & Pulina, G. (2009). The transfer of aflatoxin M1 in milk of ewes fed diet naturally contaminated by aflatoxins and effect of inclusion of dried yeast culture in the diet. Journal of Dairy Science, 92, 4997–5004. doi: https://doi.org/10.3168/jds.2008-1684
- Bojidarka, I., & Michael, S. (2014). Raman spectroscopic and mass spectrometric determination of aflatoxins. Food Analytical Methods, 7, 242–256. doi: https://doi.org/10.1007/s12161-013-9701-x
- Chen, L., Wen, F., Li, M., Guo, X. D., Li, S. L., Zheng, N., & Wang, J. Q. (2017). A simple aptamer-based fluorescent assay for the detection of Aflatoxin B1 in infant rice cereal. Food Chemistry, 215, 377–382. doi: https://doi.org/10.1016/j.foodchem.2016.07.148
- Cheraghali, A. M., Yazdanpanah, H., Doraki, N., Abouhossain, G., Hassibi, M., Ali-abadi, S., … Askarian, A. (2007). Incidence of aflatoxins in Iran pistachio nuts. Food and Chemical Toxicology, 45, 812–816. doi: https://doi.org/10.1016/j.fct.2006.10.026
- Fan, L. P., Lau, C. W., & Lu, J. Z. (2005). Magnetic bead-based chemiluminescent metal immunoassay with a colloidal gold label. Analytical Chemistry, 77, 3238–3242. doi: https://doi.org/10.1021/ac050163b
- Fu, X. L., Meng, M., Zhang, Y., Yin, Y. M., Zhang, X. S., & Xi, R. M. (2012). Chemiluminescence enzyme immunoassay using magnetic nanoparticles for detection of neuron specific enolase in human serum. Analytica Chimica Acta, 722, 114–118. doi: https://doi.org/10.1016/j.aca.2012.02.007
- Ghalia, R., Belouaera, I., Hdiria, S., Ghorbela, H., Maaroufib, K., & Hedillia, A. (2009). Simultaneous HPLC determination of aflatoxins B1, B2, G1 and G2 in Tunisian sorghum and pistachios. Journal of Food Composition and Analysis, 22, 751–755. doi: https://doi.org/10.1016/j.jfca.2009.04.009
- Goud, K., Catanante, G., Hayat, A., Satyanarayana, M., Gobi, K., & Marty, J. (2016). Disposable and portable electrochemical aptasensor for label free detection of aflatoxin B1 in alcoholic beverages. Sensors and Actuators B: Chemical, 235, 466–473. doi: https://doi.org/10.1016/j.snb.2016.05.112
- Jiang, W. X., Wang, Z. H., Greta, N., Zhang, J., Niu, L. L., & Shen, J. Z. (2013). Simultaneous determination of aflatoxin B1 and aflatoxin M1 in food matrices by enzyme-linked immunosorbent assay. Food Analytical Methods, 6, 767–774. doi: https://doi.org/10.1007/s12161-012-9484-5
- Jin, H., Lin, J. M., Wang, X., Xin, T. B., Liang, S. X., Li, Z. J., & Hu, G. M. (2009). Magnetic particle-based chemiluminescence enzyme immunoassay for free thyroxine in human serum. Journal of Pharmaceutical and Biomedical Analysis, 50, 891–896. doi: https://doi.org/10.1016/j.jpba.2009.06.011
- Kong, W. J., Xiong, J., Yue, H., & Fu, Z. F. (2015). Sandwich fluorimetric method for specific detection of Staphylococcus aureus based on antibiotic-affinity strategy. Analytical Chemistry, 87, 9864–9868. doi: https://doi.org/10.1021/acs.analchem.5b02301
- Li, S. C., Chen, J. H., Cao, H., Yao, D. S., & Liu, D. L. (2011). Amperometric biosensor for aflatoxin B1 based on aflatoxin-oxidase immobilized on multiwalled carbon nanotubes. Food Control, 22, 43–49. doi: https://doi.org/10.1016/j.foodcont.2010.05.005
- Li, Q. F., Lv, S. Z., Lu, M. H., Lin, Z. Z., & Tang, D. P. (2016). Potentiometric competitive immunoassay for determination of aflatoxin B1 in food by using antibody-labeled gold nanoparticles. Microchimica Acta, 183, 2815–2822. doi: https://doi.org/10.1007/s00604-016-1929-x
- Lin, Y., Zhou, Q., Tang, D., Niessner, R., Yang, H., & Knopp, D. (2016). Silver nanolabels-assisted ion-exchange reaction with CdTe quantum dots mediated exciton trapping for signal-on photoelectrochemical immunoassay of mycotoxins. Analytical Chemistry, 88, 7858–7866. doi: https://doi.org/10.1021/acs.analchem.6b02124
- Liu, B. H., Hsu, Y. T., Lu, C. C., & Yu, F. H. (2013). Detecting aflatoxin B1 in foods and feeds by using sensitive rapid enzyme-linked immunosorbent assay and gold nanoparticle immunochromatographic strip. Food Control, 30, 184–189. doi: https://doi.org/10.1016/j.foodcont.2012.07.008
- Liu, J. W., Lu, C. C., Liu, B. J., & Yu, F. Y. (2016). Development of novel monoclonal antibodies-based ultrasensitive enzyme-linked immunosorbent assay and rapid immunochromatographic strip for aflatoxin B1 detection. Food Control, 59, 700–707. doi: https://doi.org/10.1016/j.foodcont.2015.06.038
- Liu, Z. S., Zhang, L., Yang, H., Zhu, Y. H., Jin, W., Song, Q., & Yang, X. L. (2010). Magnetic microbead-based enzyme-linked immunoassay for detection of Schistosoma japonicum antibody in human serum. Analytical Biochemistry, 404, 127–134. doi: https://doi.org/10.1016/j.ab.2010.05.006
- Ma, H., Sun, J., Zhang, Y., & Xia, S. (2016). Disposable amperometric immunosensor for simple and sensitive determination of aflatoxin B1 in wheat. Biochemical Engineering Journal, 115, 38–46. doi: https://doi.org/10.1016/j.bej.2016.08.003
- Masoomi, L., Sadeghi, O., Banitaba, M. H., Shahrjerdi, A., & Davarani, S. S. H. (2013). A non-enzymatic nanomagnetic electro-immunosensor for determination of Aflatoxin B1 as a model antigen. Sensors and Actuators B: Chemical, 177, 1122–1127. doi: https://doi.org/10.1016/j.snb.2012.11.067
- Meritxell, V., Antonio, G., Ivan, A., Jordi, D., Francesc, B., Montserrat, A., & Lluís, C. (2004). Determination of aflatoxins B1, G1, B2 and G2 in medicinal herbs by liquid chromatography-tandem mass spectrometry. Journal of Chromatography A, 1048, 25–29. doi: https://doi.org/10.1016/j.chroma.2004.07.033
- Muhammad, A. A., Javed, I., Aftab, A., Mobeen, A. K., Zuzzer, A. S., & Khalid, J. (2016). Development and validation of a high-performance liquid chromatography method with post-column derivatization for the detection of aflatoxins in cereals and grains. Toxicology and Industrial Health, 32, 1122–1134. doi: https://doi.org/10.1177/0748233714547732
- Qin, X., & Lin, J. M. (2015). Advances and applications of chemiluminescence immunoassay in clinical diagnosis and foods safety. Chinese Journal of Analytical Chemistry, 43, 929–938. doi:10.1016/S1872-2040(15)60831-3 doi: https://doi.org/10.1016/S1872-2040(15)60809-X
- Saha, D., Acharya, D., Roy, D., Shrestha, D., & Dhar, T. K. (2007). Simultaneous enzyme immunoassay for the screening of aflatoxin B1 and ochratoxin A in chili samples. Analytica Chimica Acta, 584, 343–349. doi: https://doi.org/10.1016/j.aca.2006.11.042
- Seok, Y., Byun, J., Shim, W., & Kim, M. (2015). A structure-switchable aptasensor for aflatoxin B1 detection based on assembly of an aptamer/split DNAzyme. Analytica Chimica Acta, 886, 182–187. doi: https://doi.org/10.1016/j.aca.2015.05.041
- Shim, W. B., Kyeongyeol, K., Ofori, J. A., Chung Y, Y. C., & Chung, D. H. (2012). Occurrence of aflatoxins in herbal medicine distributed in South Korea. Journal of Food Protection, 75, 1991–1999. doi: https://doi.org/10.4315/0362-028X.JFP-12-190
- Shim, W. B., Mun, H., Joung, H. A., Chung, D. H., & Kim, M. G. (2014). Chemiluminescence competitive aptamer assay for the detection of aflatoxin B1 in corn samples. Food Control, 36, 30–35. doi: https://doi.org/10.1016/j.foodcont.2013.07.042
- Sun, X. L., Zhao, X. L., Tang, J., Gu, X. H., & Zhou, J. (2006). Development of an immunochromatographic assay for detection of aflatoxin B1 in foods. Food Control, 17, 256–262. doi: https://doi.org/10.1016/j.foodcont.2004.10.007
- Vdovenko, M. M., Lu, C. C., Yu, F. Y., & Sakharov, I. Y. (2014). Development of ultrasensitive direct chemiluminescent enzyme immunoassay for determination of aflatoxin M1 in milk. Food Chemistry, 158, 310–314. doi: https://doi.org/10.1016/j.foodchem.2014.02.128
- Xu, J., Li, W., Liu, R., Yang, Y., Lin, Q. X., Xu, J. J., Shen, P., et al. (2016). Ultrasensitive low-background multiplex mycotoxin chemiluminescence immunoassay by silica-hydrogel photonic crystal microsphere suspension arrays in cereal samples. Sensors and Actuators B: Chemical, 232, 577–584. doi: https://doi.org/10.1016/j.snb.2016.03.123
- Xu, K., Sun, Y., Li, W., Xu, J., & Cao, B. (2014). Multiplex chemiluminescent immunoassay for screening of mycotoxins using photonic crystal microsphere suspension array. The Analyst, 139, 771–777. doi: https://doi.org/10.1039/C3AN02032K
- Yao, M. W., Wang, L. Y., & Fang, C. Z. (2017). The chemiluminescence immunoassay for aflatoxin B1 based on functionalized magnetic nanoparticles with two strategies of antigen probe immobilization. Luminescence, 32, 661–665. doi: https://doi.org/10.1002/bio.3235
- Yu, F. Y., Anastasia, V. G., Marina, M. V., & Ivan, Y. S. (2013). Development of ultrasensitive direct chemiluminescent enzyme immunoassay for determination of aflatoxin B1 in food products. Talanta, 107, 25–29. doi: https://doi.org/10.1016/j.talanta.2012.12.047
- Zeng, X. Q., Gao, H. J., Pan, D. D., Sun, Y. Y., Cao, J. X., Wu, Z., & Pan, Z. Y. (2015). Highly sensitive electrochemical determination of alfatoxin B1 using quantum dots-assembled amplification labels. Sensors, 15, 20648–20658. doi: https://doi.org/10.3390/s150820648
- Zhang, B., Du, D. L., Meng, M., Serge, A. E., Victor, B. R., He, X. D., … Xi, R. M. (2014). A magnetic particle-based competitive enzyme immunoassay for rapid determination of ciprofloxacin: A potential method for the general detection of fluoroquinolones. Analytical Letters, 47, 1134–1146. doi: https://doi.org/10.1080/00032719.2013.865197
- Zhang, H. S., Qi, S. W., Rao, J., Li, Q. L., Yin, L., & Lu, J. L. (2013). Development of a rapid and high-performance chemiluminescence immunoassay based on magnetic particles for protein S100B in human serum. Luminescence, 28, 927–932. doi: https://doi.org/10.1002/bio.2461
- Zhang, H. S., & Su, W. Q. (2011). A rapid and sensitive chemiluminescence immunoassay based on magnetic particles for squamous cell carcinoma antigen in human serum. Clinica Chimica Acta, 412, 1572–1577. doi: https://doi.org/10.1016/j.cca.2011.05.005