ABSTRACT
Ovotransferrin (OVT) is one of the major proteins in avian albumen that exerts diverse biological activities. This study is aimed to investigate the antiviral effect and mechanism of OVT from the avian egg. Effects of OVT on vesicular stomatitis virus (VSV)-infected mouse peritoneal macrophage were detected by real-time quantitative polymerase chain reaction, enzyme-linked immunosorbent assay, and western blot. In OVT-pretreated mouse peritoneal macrophages, results showed that replication of VSV was significantly suppressed and production of type I interferon (IFN) was increased. OVT down-regulated ring finger protein 125 (RNF125) production and up-regulated retinoic-acid-inducible gene I (RIG-I) expression by the nuclear factor-κB signalling pathway, resulting in the enhancement of the production of type I IFN. It demonstrated that the antiviral effect of OVT on innate immunity is enhanced by promoting type I IFN expression, indicating its potential as a functional food ingredient.
Introduction
The egg is considered as a rich source of nutrients with high-quality proteins (Kovacs-Nolan, Phillips, & Mine, Citation2005), especially in the egg white. These proteins are equipped with various bioactive components. Proteins make up about 11–13% of egg white, including ovalbumin, ovotransferrin (OVT), ovomucoid, lysozyme, and ovomucin. These proteins are a major research focus in the field of food science and nutritional science. OVT is one of the major proteins in avian albumen, accounting for approximately 12% of total egg white proteins (Kovacs-Nolan et al., Citation2005). It is an iron-binding glycoprotein consisting of 686 amino acids. It is well known for iron transport and biological abilities (Giansanti, Leboffe, Pitari, Ippoliti, & Antonini, Citation2012), such as antibacterial (Prajanban, Jangpromma, Araki, & Klaynongsruang, Citation2017; Varon, Allen, Bennett, Mesak, & Scaman, Citation2013), anticarcinogenic (Ibrahim & Kiyono, Citation2009), antioxidant (Ibrahim, Hoq, & Aoki, Citation2007), especially immunomodulatory (Liu et al., Citation2017), and antiviral activities(Giansanti, Giardi, Massucci, Botti, & Antonini, Citation2007; Giansanti et al., Citation2002). OVT can inhibit chicken embryo fibroblast (CEF) infection by Marek’s disease virus (MDV) (Giansanti et al., Citation2002). It has been verified that OVT is expressed and released by CEF and lymphoblastoid cells in the absence of infection (Giansanti et al., Citation2007). When viral infection of CEF and lymphoblastoid cells takes place, the increase of OVT release occurs, which plays an important role in protecting the whole organism from viral infection spreading (Giansanti et al., Citation2007).
Viruses are major challenges to human health and survival (Morens, Folkers, & Fauci, Citation2004). The human body will mobilize various defense when it is attacked and infected by pathogens, and the innate immunity is the first line of defense against virus infection (Akira, Uematsu, & Takeuchi, Citation2006; Beutler et al., Citation2007; Medzhitov, Citation2007). Macrophages are the most plastic cells of the haematopoietic system and found in all tissues and show great functional diversity (Wynn, Chawla, & Pollard, Citation2013). They are important for recognizing and responding to pathogens in innate immunity. These front-line cells under innate immunity recognize viral infection through host pattern-recognition receptors (PRRs), trigger activation of downstream signalling pathways, express pro-inflammatory cytokines, such as IFN, and eventually exert antiviral effects. IFN synthesis and secretion in innate immune responses play a pivotal role. IFN can combine with type I interferon receptor IFNAR1/2 on the cytomembrane, triggering signalling cascades to transfer the signals to the cell nucleus, which regulates IFN-stimulated genes (ISGs), induces target cells to produce specific antiviral proteins, and inhibits the transcription of viral proteins or degradation of virus RNA (Shi et al., Citation2010).
The antiviral effect of OVT has been confirmed, but its mechanism has been rarely reported. Moreover, it is also reported that OVT regulates macrophage functions in chickens (Xie, Huff, Huff, Balog, & Rath, Citation2002). Therefore, there is an increasing interest in the search for bioactive food components, such as OVT, for the enhancement of innate immunity against viruses. This study aimed to explore effects of OVT on virus replication and type I IFN production by using vesicular stomatitis virus (VSV)-infected mouse peritoneal macrophages as a model in vitro, eventually clarifying its antiviral mechanism.
Materials and methods
Materials
Ovotransferrin (purity ≥ 98%) from chicken egg white was purchased from Sigma-Aldrich Co., Ltd. (St. Louis, MO, USA). RPMI-1640 medium, foetal bovine serum, and Western Blot Protein Marker were purchased from Thermo Scientific (Waltham, MA, USA). 0.25% trypsin with ethylenediaminetetraacetic acid (EDTA) was obtained from Gibco/Life Technology (Carlsbad, CA, USA). Trizol was purchased from Invitrogen (Carlsbad, CA, USA). Fast200 mRNA Miniprep Kit was purchased from Xunjie Company (Shanghai, China). Reverse transcriptase was purchased from Takara Biotechnology (Dalian) Co., Ltd (Dalian, China). The RT–PCR SYBR Green Kit was obtained from Sangon Biotech Corporation of Shanghai (Shanghai, China). IFN-β and IFN-4α ELISA Kits were purchased from PBL (Norwich, Norfolk, UK). Horseradish peroxidase-linked second antibody was purchased from Santa Cruz Biotechnologies (Santa Cruz, CA, USA). Specific first antibodies including glyceraldehyde 3-phosphate dehydrogenase and RIG-I were obtained from Cell Signaling Technology (Boston, MA, USA). Pyrrolidinecarbodithoic acid (PDTC, PD98059, SP600125, and SB203580 were obtained from Calbiochem (San Diego, CA, USA). The bicinchoninic acid (BCA) Kit for Protein Determination and Western Chemiluminescent Substrate were obtained from Pierce (Rockford, IL, USA). EZ-ChIP kit, trimethylation of histone H3 at lysine 4 (H3K4me3), and H3K27me3 antibodies were purchased from Millipore (Boston, MA, USA). All other reagents were of analytic grade.
Virus and mice
VSV was obtained from professor Wei Pan in the Second Military Medical University. C57 mice (6–8 weeks) were purchased from Shanghai Picard Experimental Animals Company. Toll-like receptor 3 (TLR3)-deficient mice were obtained from American Jackson Laboratories (B6, 129S1-Tlr3tm1Flv/J, 005217). TLR4-, TLR9-, and myeloid differentiation primary-response protein 88 (MyD88)-deficient mice were obtained from Professor Shizuo Akir in Osaka University. RIG-I-deficient mice were obtained from Professor Zhugang Wang in Shanghai Jiao Tong University School of Medicine. All animals were handled according to protocols approved by the Institutional Animal Care and Use Committee of the National Health Research Institute.
Separation and culture of macrophages
Macrophages were separated according to the method of Lang Rutschman, Greaves, and Murray (Citation2002). The collected cells were re-suspended at a density of 2 × 105 cells/mL in a 24-well plate containing 0.5 mL RPMI-1640 medium supplemented with 10% foetal bovine serum, 100 U/mL penicillin, and 100 µg/mL streptomycin and cultured in a humidified 5% CO2 incubator at 37°C for 2 h. The non-adherent cells were removed, and the adherent cells were used for the following experiments. Macrophages were pretreated with OVT at 100 ng/mL final concentration for 12 h. Then, VSV (MOI = 100) infected the cells for 1 h. The supernatant was replaced with fresh medium and the cells were cultured in a humidified 5% CO2 incubator at 37°C for 24 h for the following experiments.
Real-time quantitative polymerase chain reaction
Total RNA was isolated using Trizol (Invitrogen, Carlsbad, CA, USA), and total mRNA was isolated using the Fast200 mRNA Miniprep Kit (Shanghai Xunjie Company, Shanghai, China). Real-time quantitative polymerase chain reaction (RT-qPCR) was performed by using the SYBR Green RT–PCR Master Mix Kit (Takara, Dalian China) on Roche Light Cycler 1.5. Gene of GAPDH was used as a reference according to the N gene sequence from the structural protein of VSV published by Banerjee, Rhodes, and Gill (Citation1984). Design primers with software Oligo6.0. All primers are listed as .
Table 1. Primers of qRT–PCR.
Enzyme-linked immunosorbent assay
A total of 2 × 105 cells/mL mouse peritoneal macrophage cells (0.5 mL culture supernatant) were plated into 24-well plates and pretreated with OVT and VSV, as previously described. Supernatants were then collected. The concentrations of IFN-β and IFN-4α in the supernatants were measured using the ELISA Kit according to the methods of Suzuki and Tamada (Citation2009).
Western blot analysis
About 2 × 106 cells were taken in an EP tube followed by centrifugation (3500g × 5 min), and then the supernatant was discarded. A total of 300 μL of M-PER protein extraction reagent (Pierce, Rockford, IL, USA) containing Triton X-100, deoxy sodium cholate, NO-40, sodium metavanadate, and EDTA, which could effectively inhibit the activity of protease and maintain the integrity of the protein extraction, were added for precipitation, placed in an ice bath for 30 min and oscillated per 10 min. After centrifugation at 12,500g × 15 min at 4°C, the supernatant was transferred to a new EP tube stored in −20°C to be used for the following experiments. We took a handful of supernatants to perform the protein quantification according to the instruction book of the BCA Kit (Pierce, Rockford, IL, USA) for Protein Determination. Each protein sample concentration was adjusted to the same amount. Equal quantities of 6× loading buffer (100 mmol/L Tris–HCl, 200 mmol/L DTT, 4% SDS, 0.2% bromophenol blue, 20% glycerinum, and pH 6.8) were added into samples and shaken well. Afterwards, samples were heated to 100°C in a boiling water bath for 5 min. Samples (30 μg protein/lane) and a protein marker (Thermo Scientific, Waltham, MA, USA) were loaded on the same gel simultaneously. Protein separation was done on 12% sodium dodecyl sulfate–polyacrylamide gel electrophoresis, and proteins were transferred to nitrocellulose blotting membranes using Trans-Blot Turbo (Bio-Rad). Membranes were blocked for 2 h using Tris-buffered saline Tween (TBST) containing 5% skim milk (pH 7.6, containing 0.05% Tween20). After elution by TBST twice for 15 min, primary antibodies RIG-I (D14G6) rabbit monoclonal antibody and GAPDH (D16H11) rabbit monoclonal antibody (1:1000) (Cell Signaling Technology, Boston, MA, USA) were added and incubated overnight at 4°C. After the first antibody was eluted for 15 min thrice, horseradish peroxidase-linked second antibody (Santa Cruz Biotechnology, Santa Cruz, CA, USA) was added and incubated for 1 h, then eluted again as previously described. Isopyknic solutions A and B of chemiluminescence Western blot reagents were mixed and incubated for 5 min at room temperature, and photos were taken using an E-Gel Imager.
Determination of the titre of VSV
Macrophages were incubated with 100 ng/mL final concentration of OVT for 12 h. VSV (MOI = 100) infected the cells for 1 h. Then replace with the fresh medium for 24 h and collect the cell culture supernatants. Perform serial 10-fold dilutions for a total of 12 dilutions. 1 × 104 cells/well HEK293 cells (Cell Bank of Chinese Academy of Sciences) were plated into a 96-well plate and cultured in a humidified 5% CO2 incubator at 37°C overnight. Add diluted supernatants to the corresponding well and cells were cultured for 72 h. Each dilution was repeated eight times. Calculate tissue culture infective dose 50 (TCID50) (Montefiori, Citation2005) by using the Reed–Muench formula.
Chromatin immunoprecipitation
The chromatin immunoprecipitation (ChIP) kit (Millipore, Boston, MA, USA) standard steps were used for cross-linking and processing of cells. Specific antibodies, including H3K4me3 and H3K27me3, were purchased from Millipore. Five micrograms of antibodies as previously described were used in the ChIP system, and 10 μL of sonicated DNA served as the input control. ChIP results were subjected to qPCR with primer 1 and primer 2. Primer 1 and Primer 2 are primers of the RNF125 promoter region.
Statistical analysis
Data are presented as the mean ± standard deviation (SD) for the indicated number of independent experiments that were repeated at least thrice. Data were subjected to the Student’s t-test for analysis of statistical significance, and values of p < .05 are considered statistically significant.
Results
OVT suppressed replication of RNA virus in macrophages
Firstly, we incubated macrophages with 100 ng/mL final concentration of OVT for 12 h. VSV (MOI = 100) was added into the cells for 1 h. Afterwards, cells were incubated with fresh medium for 72 h. The total RNA of cells was extracted to analyse VSV replication in macrophages, and the supernatant was collected to determine the titre of virus. Results showed that replication of virus in OVT-pretreated cells was inhibited significantly ((A)). The titre of virus in the supernatant was significantly decreased as well ((B)). Obviously, OVT suppressed VSV replication in mouse peritoneal macrophages.
Figure 1. Effect of OVT on replication of VSV in macrophages. (A) Macrophages were incubated with medium or 100 ng/mL final concentration of OVT for 12 h. VSV (MOI = 100) infected the cells for 72 h and then carry out RT–PCR. (B) Collect the supernatant of A. Determine the titre of VSV. Data shown are mean ± SD of triplicate measurements. Compared with the control group: **p < .01. All data are representative of three independent experiments.
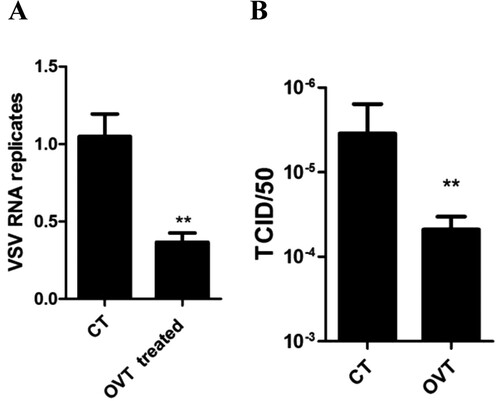
OVT up-regulated IFN expression in macrophages
Macrophages captured the virus and suppressed its replication in a type I IFN-dependent manner (Honke et al., Citation2011). OVT suppressed VSV replication in macrophages. Therefore, the next was to explore the effect of OVT on the type I IFN production.
Production of IFN-β and IFN-4α was detected here. As shown in , mRNA expression of IFN-β ((A)) and IFN-4α ((C)) significantly increased in OVT-treated macrophages. Moreover, productions of IFN-β ((B)) and IFN-4α ((D)) from OVT-treated supernatant were significantly up-regulated in the same way. These results suggested OVT promoted the type I IFN production in VSV-infected macrophages to enhance the antiviral ability of macrophages.
Figure 2. Effect of OVT on the production of type I IFN. (A) VSV-infected macrophages which were pretreated with medium and 100 ng/mL final concentration of OVT for 18 and 24 h, and gene expression of IFN-β was examined by RT-qPCR. (B) Collect supernatant from A to test production of IFN-β by ELISA. (C) VSV-infected macrophages which were pretreated with medium and 100 ng/mL final concentration of OVT for 18 and 24 h, and gene expression of IFN-4α was examined by RT-qPCR. (D) Collect the supernatant from C to test production of IFN-β by ELISA. Data shown are mean ± SD of triplicate measurements. Compared with the control group: *p < .05 and **p < .01. All data are representative of three independent experiments.
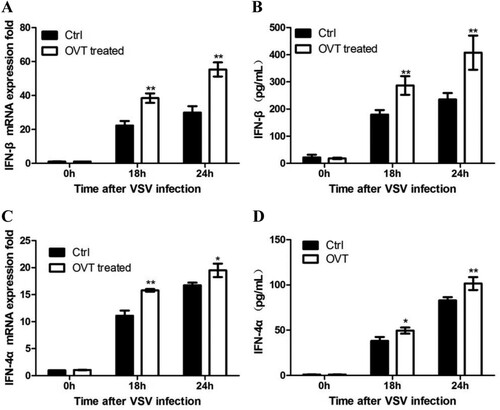
OVT promoted type I IFN production by RIG-I pathway
Considering that TLRs and retinoic-acid-inducible gene-I-like receptors (RLRs), two PRRs are important in inhibiting viral infection in cells, the effect of TLR signalling pathway on IFN-β secretion in OVT-treated macrophages was determined. After macrophages from different deficient mice were incubated with 100 ng/mL final concentration of OVT for 12 h, VSV (MOI = 100) infected the cells. Total RNA was extracted at 0 and 24 h. The expression of IFN-β was tested by RT-qPCR. All data were involved in the results. Compared with wild-type mouse peritoneal macrophages ((A)), there are the same up-regulation trends of IFN-β mRNA expression in TLR3- ((B)), TLR4- ((C)), TLR9- ((D)), and MyD88- ((E)) deficient mouse peritoneal macrophages which were treated with OVT. However, it was found that VSV infection was unable to up-regulate IFN-β mRNA expression in RIG-I-deficient mouse peritoneal macrophages which were treated with OVT ((F)). It suggested that it was not via the TLR pathways, and it was through the RIG-I pathway that OVT up-regulated the type I IFN expression.
Figure 3. Signalling pathway of the production of type I IFN. (A) VSV-infected different mice peritoneal macrophages which were pretreated with medium or 100 ng/mL final concentration of OVT for 24 h, then detect the IFN-β gene expression. (A) Wild-type C57 mice peritoneal macrophages. (B) TLR3-deficient mice peritoneal macrophages. (C) TLR4-deficient mice peritoneal macrophages. (D) TLR9-deficient mice peritoneal macrophages. (E) MyD88-deficient mice peritoneal macrophages. (F) RIG-I-deficient mice peritoneal macrophages. Data shown are mean ± SD of triplicate measurements. Compared with the control group: *p < .05 and **p < .01. All data are representative of three independent experiments.
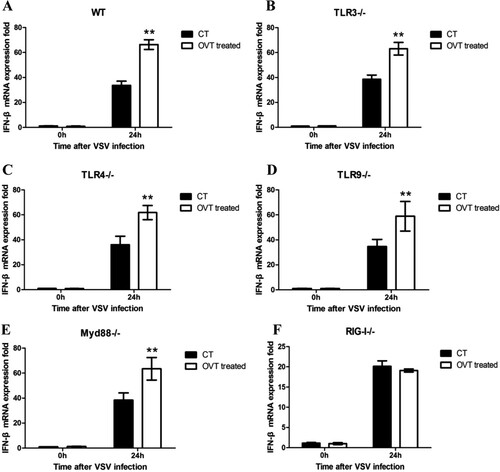
OVT up-regulated RIG-I expression
RIG-I, a member of RLR family, detects viral RNA in the cytosol of most cell types. Extensive functional studies have demonstrated the importance of RIG-I in sensing RNA virus infections, with RIG-I playing a critical role in the detection of rhabdoviruses (Chan & Gack, Citation2015). Especially, RIG-I is the primary sensor of viral RNA for VSV (Loo & Gale, Citation2011; Zhu et al., Citation2014). However, how OVT affects the RIG-I remains unclear. Therefore, this study continued to test the changes of RIG-I mRNA in VSV-infected macrophages which were pretreated with OVT. (A) showed that OVT had no effect on RIG-I mRNA expression in macrophages, which indicated that RIG-I were unaffected by OVT at the level of transcription. Hence, it was speculated that OVT influenced the protein expression of RIG-I. Then total proteins were extracted to detect the protein level of RIG-I by using Western blot analysis. The protein expression of RIG-I was up-regulated significantly after the treatment of OVT ((B)), implying that OVT may up-regulate RIG-I via post-translational modification control (Chen et al., Citation2013; Liu, Qian, & Cao, Citation2016).
Figure 4. Effect of OVT on RIG-I expression. (A) Macrophages were incubated with medium or 100 ng/mL final concentration of OVT for 12 h. VSV (MOI = 100) infected the cells for 12 and 24 h, then determine the gene expression of RIG-I by RT–PCR. (B) Macrophages were incubated with medium or 100 ng/mL final concentration of OVT for 12 h. VSV (MOI = 100) infected the cells for 24 h and then determine the protein phosphorylation of RIG-I by Western blot. Data shown are mean ± SD of triplicate measurements. All data are representative of three independent experiments.
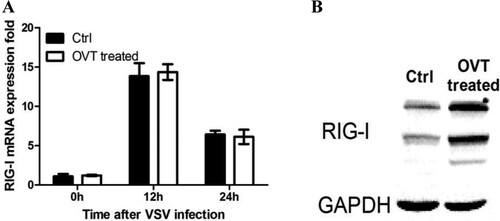
OVT down-regulated the expression of RNF125
Because OVT antiviral activity was dependent on RIG-I, in the next series of experiments, how OVT modulated RIG-I expression was tested. Regulatory factors of RIG-I, including RNF-125, cylindromatosis (CYLD), tripartite motif containing 25 (TRIM25), laboratory of genetics and physiology 2 (LGP2), ISG15, autophagy-related gene 5 (Atg5), and Atg12, can positively or negatively regulate RIG-I expression in the cells (Arimoto et al., Citation2007; Chan & Gack, Citation2015; Loo & Gale, Citation2011; Satoh et al., Citation2010). Cells were treated as described above, and RT-qPCR was used to test expressions of RNF-125, CYLD, TRIM25, LGP2, ISG15, Atg5, and Atg12. Results showed that mRNA expression of RNF125 had remarkably decreased ((A)). RNF125, one of the key negative regulatory factors of the RIG-I pathway, can cause ubiquitination of RIG-I, thereby resulting in degradation (Porritt & Hertzog, Citation2015). Hence, OVT down-regulated RNF125 production, leading to the up-regulation of RIG-I. The result was compatible with those above.
Figure 5. Effects of OVT on RIG-I regulators and methylation of promoter region of RNF125. (A) Macrophages were pretreated with medium or 100 ng/mL final concentration of OVT, then VSV(MOI = 100) infected the cells for 24 h. Determine the production of RNF-125,CYLD, TRIM25, LGP2, ISG15, Atg5 and Atg12 by RT–PCR. B: Macrophages were pretreated with medium or 100 ng/mL final concentration of OVT, and then the cells were incubated with DMSO, PDTC, PD98059, SP600125 and SB203580 for 30 min. Then, VSV(MOI = 100) infected the cells for 0, 12 and 24 h to detect the expression of RNF125 mRNA. (C) Macrophages were pretreated with PBS + DMSO, OVT (100 ng/mL) +DMSO, PBS + PDTC and OVT (100 ng/mL) +PDTC, and then carry out ChIP by using H3K4me3 antibodies to test modification of H3K4me3 in RNF125 promoter region. (D) Macrophages were pretreated with PBS + DMSO, OVT (100 ng/mL) +DMSO, PBS + PDTC and OVT (100 ng/mL) +PDTC, and then carry out ChIP by using H3K27me3 antibodies to test modification of H3K27me3 in the RNF125 promoter region. Data shown are mean ± SD of triplicate measurements. Compared with the control group: **p < .01. All data are representative of three independent experiments.
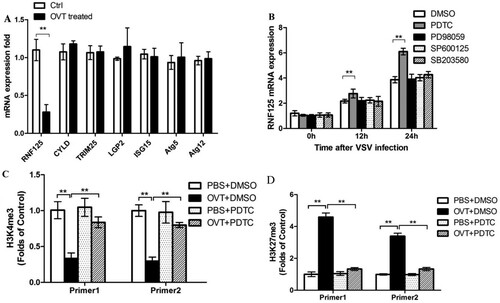
OVT down-regulated RNF125 by nuclear factor-κB signalling pathway
Then the mechanism of RNF125 down-regulation was studied in detail. After macrophages were incubated with 100 ng/mL final concentration of OVT for 12 h, PDTC, PD98059, SP600125, and SB203580, which are inhibitors of nuclear factor-κB (NF-κB), extracellular signal-regulated kinase (ERK), c-Jun N-terminal kinase (JNK), and p38 pathway, were, respectively, used to incubate cells for 30 min. The control group was incubated with dimethyl sulfoxide. VSV (MOI = 100) was added to stimulate cells for 0, 12, and 24 h. Results of RT-qPCR revealed that PDTC, an NF-κB pathway inhibitor, significantly inhibited OVT-induced down-regulation of RNF125, whereas others had no effect on down-regulation of RNF125 ((B)). This explained that OVT-induced down-regulation of RNF125 depended on the NF-κB signalling pathway.
OVT affected histone methylation status in the RNF125 promoter region
Effect of OVT treatment on histone methylation status in the RNF125 promoter region was further detected by RT-qPCR. As shown in the (C, D), OVT down-regulated H3K4 methylation and up-regulated H3K27 methylation in the RNF125 promoter region. H3K4 is the histone methylation modification that opens gene expression (Pekowska et al., Citation2011), and H3K27 is the histone methylation modification that inhibits gene expression (Kim et al., Citation2013). The methylation modification can be inhibited by PDTC, indicating that OVT down-regulated H3K4 trimethylation and up-regulated H3K27 trimethylation in the RNF125 promoter region, thereby down-regulating the expression of RNF125.
Discussion
Innate immunity is the first line of defense against pathogenic infections. It was speculated whether OVT is important in regulating the innate immune system. During infection of virus, the innate immune response is activated to suppress viral replication through up-regulation of antiviral molecules and direct the adaptive immune response. Macrophages under innate immunity recognize viral infection and express IFNs and pro-inflammatory cytokines to inhibit viral replication. This was the reason to choose VSV-infected mouse peritoneal macrophage as a research object to investigate the effect of OVT on viral replication.
The replication of VSV in mouse peritoneal macrophages which were pretreated with OVT was significantly inhibited. It turned out that OVT had an antiviral effect. OVT is an iron-binding protein in avian albumen and belongs to the transferrin family. As a member of the transferrin family, OVT has 50% sequence homology with mammalian serum transferrin and lactoferrin. They have numerous similar characters in bioactivities, such as antimicrobial activity, antiviral activity, the immune defense function, etc. (Giansanti et al., Citation2012; Wu & Acero-Lopez, Citation2012). Human and bovine lactoferrin possess antiviral effects on enveloped and non-enveloped viruses (Redwan, Uversky, El-Fakharany, & Al-Mehdar, Citation2014), such as Mayaro virus (Carvalho et al., Citation2014), cytomegalovirus (Harmsen et al., Citation1995), Norovirus (Ishikawa, Awano, Fukui, Sasaki, & Kyuwa, Citation2013), herpes simplex virus (HSV) (Marchetti et al., Citation1996), human immunodeficiency virus (HIV) (Swart et al., Citation1996), and hepatitis C virus (HCV) (El-Fakharany, Sánchez, Al-Mehdar, & Redwan, Citation2013; Liao, El-Fakkarany, Lonnerdal, & Redwan, Citation2012). Interestingly, OVT is more effective than lactoferrin and serum transferrin in antiviral activities (Giansanti et al., Citation2002). OVT can inhibit MDV in CEF and is more effective than serum transferrin and lactoferrin (Giansanti et al., Citation2002, Citation2007). This study verified the anti-RNA virus function of OVT and illuminated its mechanism in vitro. Based on the results above, OVT inhibited replication of VSV in mouse peritoneal macrophage. Similarly, lactoferrin has been reported to inhibit Echovirus 5 and HSV-1 virus replication in the host cells (Wakabayashi, Oda, Yamauchi, & Abe, Citation2014).
Macrophages are important innate immune cells (Chan-Zapata et al., Citation2017; Ghazanfari, Yaraee, Farahnejad, Hakimzadeh, & Danialy, Citation2009). A number of PRRs, such as TLRs and RIG-I-like receptors, exist on the surface of macrophages. They can recognize corresponding pathogen-associated molecular patterns (Ahn et al., Citation2016; Loo & Gale, Citation2011). They also can activate a series of downstream signalling pathways and activate innate immune cells to produce inflammatory cytokines and IFN after identifying viruses to start the natural immune response (Kawai & Akira, Citation2010; Takeuchi & Akira, Citation2010). After VSV infected mouse peritoneal macrophages which were pretreated with OVT, the production of type I IFN in macrophages increased. Apparently, OVT plays a role in antiviral effects via affecting the type I IFN production. This is similar to lactoferrin. Lactoferrin might inhibit viral replication in the cells by inducing antiviral cytokines IFN-α/β (Wakabayashi et al., Citation2014).
Activation of TLRs leads to the production of type I IFNs and expression of RLRs is greatly enhanced in response to type I IFN stimulation (Takeuchi & Akira, Citation2010). Considering the critical roles of RIG-I and TLRs in the induction of type I IFN, this study indicated that OVT-induced up-regulation of the type I IFN production was by the RIG-I pathway but not the TLRs pathway in macrophages. To investigate how OVT affected RIG-I expression, the production of RIG-I on gene levels and protein levels was detected. Results demonstrated that OVT might up-regulate RIG-I via post-translational modification control.
Numerous regulatory factors in the RIG-I pathway regulate the expression of RIG-I, including RNF-125, CYLD, TRIM25, LGP2, ISG15, Atg5, and Atg12. Thus, a complex interplay of positive and negative regulators and post-translational modifications determine RIG-I to be a pathogen sensor in the antiviral IFN pathway (Patel & García-Sastre, Citation2014). Conventional post-translational modifications such as phosphorylation and polyubiquitination have been shown to influence PRR-dependent inflammatory responses via their targeting of innate sensors and downstream signalling molecules (Cao, Citation2016). A recent study suggested that down-regulation of RNF125 expression could suppress the degradation of RIG-I protein levels, thereby enhancing the IRF-3 phosphorylation and increasing the IFN production (Arimoto et al., Citation2007). To further comprehend the regulation of OVT on RNF125, changes of histone methylation status in the RNF125 promoter region in OVT-pretreated macrophages were tested by using ChIP and RT-qPCR. Results revealed that OVT down-regulated H3K4 methylation and up-regulated H3K27 methylation in the RNF125 promoter region. Histone methylation, DNA methylation, chromatin remodelling, and non-coding RNA (ncRNA) regulation are the main ways to control gene expression in epigenetics (Renthal & Nestler, Citation2008). Methylation of histone lysine and arginine residues may lead transcription factors to approach the gene promoter region to suppress or promote gene expression (Levenson et al., Citation2004). Previous research proved that H3K4 trimethylation is related to the start and extension of transcription factors, serving as open histone gene expression, whereas H3K27 trimethylation is related to inhibition of genetic transcription serving as inhibiting histone gene expression (Rice & Allis, Citation2001). In this study, methylations of histone H3K4 and H3K27 could be inhibited by PDTC, indicating that OVT caused down-regulation of H3K4 trimethylation and up-regulation of H3K27 trimethylation in the RNF125 promoter region through the NF-κB signalling pathway, thereby leading to down-regulation of RNF125. In view of RNF125 as a negative regulatory factor of RIG-I, its down-regulation could lead to up-regulation of RIG-I expression, sequentially increasing production of type I IFN, and finally exerting its antiviral effects.
In summary, OVT can exert similar effects to lactoferrin in the innate immune system. OVT changes histone methylation status in the RNF125 promoter region by NF-κB signalling pathway, inhibiting the RNF125 expression. Its decreasing expression can result in up-regulation of RIG-I expression and promotion of type I IFN production in macrophages to exert an antiviral effect of OVT. Our discovery of a central role of OVT in antiviral innate immune has opened new directions for further studies, especially some potential applications in the development of functional food fields.
Acknowledgements
The authors thank Prof. Quanxing Wang and Meng Guo (National Key Laboratory of Medical Immunology, Second Military Medical University, Shanghai, China) for guiding the experiment and help. The authors also thank researcher Yan Jiang (Key Laboratory of Natural Product and Functional Food Jiangxi Agricultural University, Nanchang, China) for providing materials and machines.
Disclosure statement
No potential conflict of interest was reported by the authors.
Additional information
Funding
References
- Ahn, S.-I., Kim, J.-S., Hong, C.-Y., Gu, G.-J., Shin, H.-M., Paek, J. H., … Youn, H.-S. (2016). Eupatorium makinoi suppresses toll-like receptor signaling pathways. Food and Agricultural Immunology, 27(2), 242–250. doi: https://doi.org/10.1080/09540105.2015.1086315
- Akira, S., Uematsu, S., & Takeuchi, O. (2006). Pathogen recognition and innate immunity. Cell, 124(4), 783–801. doi: https://doi.org/10.1016/j.cell.2006.02.015
- Arimoto, K. I., Takahashi, H., Hishiki, T., Konishi, H., Fujita, T., & Shimotohno, K. (2007). Negative regulation of the RIG-I signaling by the ubiquitin ligase RNF125. Proceedings of the National Academy of Sciences, 104(18), 7500–7505. doi: https://doi.org/10.1073/pnas.0611551104
- Banerjee, A. K., Rhodes, D. P., & Gill, D. S. (1984). Complete nucleotide sequence of the mRNA coding for the N protein of vesicular stomatitis virus (New Jersey serotype). Virology, 137(137), 432–438. doi: https://doi.org/10.1016/0042-6822(84)90237-X
- Beutler, B., Eidenschenk, C., Crozat, K., Imler, J. L., Takeuchi, O., Hoffmann, J. A., & Akira, S. (2007). Genetic analysis of resistance to viral infection. Nature Reviews Immunology, 7(10), 753–766. doi: https://doi.org/10.1038/nri2174
- Cao, X. (2016). Self-regulation and cross-regulation of pattern-recognition receptor signalling in health and disease. Nature Reviews Immunology, 16(1), 35–50. doi: https://doi.org/10.1038/nri.2015.8
- Carvalho, C. A., Sousa, I. P., Jr., Silva, J. L., Oliveira, A. C., Goncalves, R. B., & Gomes, A. M. (2014). Inhibition of Mayaro virus infection by bovine lactoferrin. Virology, 452–453, 297–302. doi: https://doi.org/10.1016/j.virol.2014.01.022
- Chan, Y. K., & Gack, M. U. (2015). RIG-I-like receptor regulation in virus infection and immunity. Current Opinion in Virology, 12, 7–14. doi: https://doi.org/10.1016/j.coviro.2015.01.004
- Chan-Zapata, I., Canul-Canche, J., Fernández-Martín, K., Martín-Quintal, Z., Torres-Romero, J. C., Lara-Riegos, J. C., … Arana-Argáez, V. E. (2017). Immunomodulatory effects of the methanolic extract from Pouteria campechiana leaves in macrophage functions. Food and Agricultural Immunology, 5, 1–14. doi: https://doi.org/10.1080/09540105.2017.1386163
- Chen, W., Han, C., Xie, B., Hu, X., Yu, Q., Shi, L., … Cao, X. (2013). Induction of Siglec-G by RNA viruses inhibits the innate immune response by promoting RIG-I degradation. Cell, 152(3), 467–478. doi: https://doi.org/10.1016/j.cell.2013.01.011
- El-Fakharany, E. M., Sánchez, L., Al-Mehdar, H. A., & Redwan, E. M. (2013). Effectiveness of human, camel, bovine and sheep lactoferrin on the hepatitis C virus cellular infectivity: Comparison study. Virology Journal, 10(1), 199. doi: https://doi.org/10.1186/1743-422X-10-199
- Ghazanfari, T., Yaraee, R., Farahnejad, Z., Hakimzadeh, H., & Danialy, F. (2009). In vitro effect of Pleurotus floridaon macrophage cell viability and nitric oxide production. Food and Agricultural Immunology, 20(2), 105–110. doi: https://doi.org/10.1080/09540100902838198
- Giansanti, F., Giardi, M. F., Massucci, M. T., Botti, D., & Antonini, G. (2007). Ovotransferrin expression and release by chicken cell lines infected with Marek’s disease virus. Biochemistry and Cell Biology, 85(1), 150–155. doi: https://doi.org/10.1139/o06-210
- Giansanti, F., Leboffe, L., Pitari, G., Ippoliti, R., & Antonini, G. (2012). Physiological roles of ovotransferrin. Biochimica et Biophysica Acta (BBA) - General Subjects, 1820(3), 218–225. doi: https://doi.org/10.1016/j.bbagen.2011.08.004
- Giansanti, F., Rossi, P., Massucci, M. T., Botti, D., Antonini, G., Valenti, P., & Seganti, L. (2002). Antiviral activity of ovotransferrin discloses an evolutionary strategy for the defensive activities of lactoferrin. Biochemistry and Cell Biology, 80(1), 125–130. doi: https://doi.org/10.1139/o01-208
- Harmsen, M. C., Swart, P. J., de Béthune, M. P., Pauwels, R., De, C. E., The, T. H., & Meijer, D. K. (1995). Antiviral effects of plasma and milk proteins: Lactoferrin shows potent activity against both human immunodeficiency virus and human cytomegalovirus replication in vitro. Journal of Infectious Diseases, 172(2), 380–388. doi: https://doi.org/10.1093/infdis/172.2.380
- Honke, N., Shaabani, N., Cadeddu, G., Sorg, U. R., Zhang, D. E., Trilling, M., … Lang, K. S. (2011). Enforced viral replication activates adaptive immunity and is essential for the control of a cytopathic virus. Nature Immunology, 13(1), 51–57. doi: https://doi.org/10.1038/ni.2169
- Ibrahim, H. R., Hoq, M. I., & Aoki, T. (2007). Ovotransferrin possesses SOD-like superoxide anion scavenging activity that is promoted by copper and manganese binding. International Journal of Biological Macromolecules, 41(5), 631–640. doi: https://doi.org/10.1016/j.ijbiomac.2007.08.005
- Ibrahim, H. R., & Kiyono, T. (2009). Novel anticancer activity of the autocleaved ovotransferrin against human colon and breast cancer cells. Journal of Agricultural and Food Chemistry, 57(23), 11383–11390. doi: https://doi.org/10.1021/jf902638e
- Ishikawa, H., Awano, N., Fukui, T., Sasaki, H., & Kyuwa, S. (2013). The protective effects of lactoferrin against murine norovirus infection through inhibition of both viral attachment and replication. Biochemical and Biophysical Research Communications, 434(4), 791–796. doi: https://doi.org/10.1016/j.bbrc.2013.04.013
- Kawai, T., & Akira, S. (2010). The role of pattern-recognition receptors in innate immunity: Update on toll-like receptors. Nature Immunology, 11(5), 373–384. doi: https://doi.org/10.1038/ni.1863
- Kim, D. H., Tang, Z., Shimada, M., Fierz, B., Houck-Loomis, B., Bar-Dagen, M., … Lee, J. W. (2013). Histone H3K27 trimethylation inhibits H3 binding and function of SET1-like H3K4 methyltransferase complexes. Molecular and Cellular Biology, 33(24), 4936–4946. doi: https://doi.org/10.1128/MCB.00601-13
- Kovacs-Nolan, J., Phillips, M., & Mine, Y. (2005). Advances in the value of eggs and Egg components for human health. Journal of Agricultural and Food Chemistry, 53(22), 8421–8431. doi: https://doi.org/10.1021/jf050964f
- Lang, R., Rutschman, R. L., Greaves, D. R., & Murray, P. J. (2002). Autocrine deactivation of macrophages in transgenic mice constitutively overexpressing IL-10 under control of the human CD68 promoter. The Journal of Immunology, 168(7), 3402–3411. doi: https://doi.org/10.4049/jimmunol.168.7.3402
- Levenson, J. M., O’Riordan, K. J., Brown, K. D., Trinh, M. A., Molfese, D. L., & Sweatt, J. D. (2004). Regulation of histone acetylation during memory formation in the hippocampus. Journal of Biological Chemistry, 279(39), 40545–40559. doi: https://doi.org/10.1074/jbc.M402229200
- Liao, Y., El-Fakkarany, E., Lonnerdal, B., & Redwan, E. M. (2012). Inhibitory effects of native and recombinant full-length camel lactoferrin and its N and C lobes on hepatitis C virus infection of Huh7.5 cells. Journal of Medical Microbiology, 61(Pt 3), 375–383. doi: https://doi.org/10.1099/jmm.0.033894-0
- Liu, J., Qian, C., & Cao, X. (2016). Post-translational modification control of innate immunity. Immunity, 45(1), 15–30. doi: https://doi.org/10.1016/j.immuni.2016.06.020
- Liu, L., Xu, M., Tu, Y., Du, H., Zhou, Y., & Zhu, G. (2017). Immunomodulatory effect of protease hydrolysates from ovotransferrin. Food & Function, 8(4), 1452–1459. doi: https://doi.org/10.1039/c6fo01669c
- Loo, Y. M., & Gale, M. (2011). Immune signaling by RIG-I-like receptors. Immunity, 34(5), 680–692. doi: https://doi.org/10.1016/j.immuni.2011.05.003
- Marchetti, M., Longhi, C., Conte, M. P., Pisani, S., Valenti, P., & Seganti, L. (1996). Lactoferrin inhibits herpes simplex virus type 1 adsorption to Vero cells. Antiviral Research, 29(2–3), 221–231. doi: https://doi.org/10.1016/0166-3542(95)00840-3
- Medzhitov, R. (2007). Recognition of microorganisms and activation of the immune response. Nature, 449(7164), 819–826. doi: https://doi.org/10.1038/nature06246
- Montefiori, D. C. (2005). Evaluating neutralizing antibodies against HIV, SIV, and SHIV in luciferase reporter gene assays. Current Protocols in Immunology, Chapter 12(Unit 12), Unit 12.11.
- Morens, D. M., Folkers, G. K., & Fauci, A. S. (2004). The challenge of emerging and re-emerging infectious diseases. Nature, 430, 242–249. doi: https://doi.org/10.1038/nature02759
- Patel, J. R., & García-Sastre, A. (2014). Activation and regulation of pathogen sensor RIG-I. Cytokine & Growth Factor Reviews, 25(5), 513–523. doi: https://doi.org/10.1016/j.cytogfr.2014.08.005
- Pekowska, A., Benoukraf, T., Zacarias-Cabeza, J., Belhocine, M., Koch, F., Holota, H., … Spicuglia, S. (2011). H3k4 tri-methylation provides an epigenetic signature of active enhancers. The EMBO Journal, 30(20), 4198–4210. doi: https://doi.org/10.1038/emboj.2011.295
- Porritt, R. A., & Hertzog, P. J. (2015). Dynamic control of type I IFN signalling by an integrated network of negative regulators. Trends in Immunology, 36(3), 150–160. doi: https://doi.org/10.1016/j.it.2015.02.002
- Prajanban, B. O., Jangpromma, N., Araki, T., & Klaynongsruang, S. (2017). Antimicrobial effects of novel peptides cOT2 and sOT2 derived from Crocodylus siamensis and Pelodiscus sinensis ovotransferrins. Biochimica et Biophysica Acta (BBA) – Biomembranes, 1859(5), 860–869. doi: https://doi.org/10.1016/j.bbamem.2017.01.035
- Redwan, E. M., Uversky, V. N., El-Fakharany, E. M., & Al-Mehdar, H. (2014). Potential lactoferrin activity against pathogenic viruses. Comptes Rendus Biologies, 337(10), 581–595. doi: https://doi.org/10.1016/j.crvi.2014.08.003
- Renthal, W., & Nestler, E. J. (2008). Epigenetic mechanisms in drug addiction. Trends in Molecular Medicine, 14(8), 341–350. doi: https://doi.org/10.1016/j.molmed.2008.06.004
- Rice, J. C., & Allis, C. D. (2001). Histone methylation versus histone acetylation: New insights into epigenetic regulation. Current Opinion in Cell Biology, 13(3), 263–273. doi: https://doi.org/10.1016/S0955-0674(00)00208-8
- Satoh, T., Kato, H., Kumagai, Y., Yoneyama, M., Sato, S., Matsushita, K., … Takeuchi, O. (2010). LGP2 is a positive regulator of RIG-I- and MDA5-mediated antiviral responses. Proceedings of the National Academy of Sciences, 107(4), 1512–1517. doi: https://doi.org/10.1073/pnas.0912986107
- Shi, H. X., Yang, K., Liu, X., Liu, X. Y., Wei, B., Shan, Y. F., … Wang, C. (2010). Positive regulation of interferon regulatory factor 3 activation by Herc5 via ISG15 modification. Molecular and Cellular Biology, 30(10), 2424–2436. doi: https://doi.org/10.1128/MCB.01466-09
- Suzuki, T., & Tamada, H. (2009). WITHDRAWN: Modulation of microRNA processing by p53. Nature, 460(7254), 529–533. doi: https://doi.org/10.1038/nature08199
- Swart, P. J., Kuipers, M. E., Smit, C., Pauwels, R., de Béthune, M. P., Clercq, E., … Huisman, J. G. (1996). Antiviral effects of milk proteins: Acylation results in polyanion ic compounds with potent activity against human immunodeficiency virus types 1 and 2 in vitro. AIDS Research and Human Retroviruses, 12(9), 769–775. doi: https://doi.org/10.1089/aid.1996.12.769
- Takeuchi, O., & Akira, S. (2010). Pattern recognition receptors and inflammation. Cell, 140(6), 805–820. doi: https://doi.org/10.1016/j.cell.2010.01.022
- Varon, O., Allen, K. J., Bennett, D. C., Mesak, L. R., & Scaman, C. H. (2013). Purification and characterization of tinamou egg white ovotransferrin as an antimicrobial agent against foodborne pathogenic bacteria. Food Research International, 54(2), 1836–1842. doi: https://doi.org/10.1016/j.foodres.2013.02.041
- Wakabayashi, H., Oda, H., Yamauchi, K., & Abe, F. (2014). Lactoferrin for prevention of common viral infections. Journal of Infection and Chemotherapy, 20(11), 666–671. doi: https://doi.org/10.1016/j.jiac.2014.08.003
- Wu, J., & Acero-Lopez, A. (2012). Ovotransferrin: Structure, bioactivities, and preparation. Food Research International, 46(2), 480–487. doi: https://doi.org/10.1016/j.foodres.2011.07.012
- Wynn, T. A., Chawla, A., & Pollard, J. W. (2013). Macrophage biology in development, homeostasis and disease. Nature, 496(7446), 445–455. doi: https://doi.org/10.1038/nature12034
- Xie, H., Huff, G. R., Huff, W. E., Balog, J. M., & Rath, N. C. (2002). Effects of ovotransferrin on chicken macrophages and heterophil-granulocytes. Developmental & Comparative Immunology, 26(9), 805–815. doi: https://doi.org/10.1016/S0145-305X(02)00028-9
- Zhu, J., Zhang, Y., Ghosh, A., Cuevas, R. A., Forero, A., Dhar, J., … Sarkar, S. N. (2014). Antiviral activity of human OASL protein is mediated by enhancing signaling of the RIG-I RNA sensor. Immunity, 40(6), 936–948. doi: https://doi.org/10.1016/j.immuni.2014.05.007