ABSTRACT
This study mainly investigated the effects of different dietary levels of glutamate (Glu) and aspartate (Asp) on growth performance, blood amino acids, and amino acid transporters in piglets. Forty-two healthy piglets were randomly divided into six groups (n = 7): a control group in which piglets were fed 2.9% Glu and 1.5% Asp and other groups in which piglets received 1.3% or 1.7% Asp and 2.6%, 3.2%, or 3.5% Glu for 21 days. Growth performance, serum amino acid profiles from the mesenteric vein, portal vein, and anterior vena cava, and amino acid transporters in the liver were determined. The results showed that lower doses of Asp promoted growth and enhanced the amino acids, while high doses of Asp and Glu reduced growth and the amino acid pool in piglets (P < 0.05). Meanwhile, 3.2% Glu increased branched chain amino acids in the portal vein and anterior vena cava (P < 0.05). 3.5% Glu downregulated SLC7A1, SLC7A7, and SLC6A19 expression in the liver (P < 0.05). Collectively, these results indicated that different dietary doses of Glu and Asp influenced growth performance and serum amino acid.
Introduction
Glutamate (Glu) and aspartate (Asp), two acidic amino acids, are considered as non-essential amino acids in classical nutrition (Wu, Citation2010). Glu and Asp have been indicated to play important roles in physiological and biochemical processes (Duan et al., Citation2014; Wang et al., Citation2016). For example, Glu and Asp serve as major sources of energy and improve intestinal and liver function by alleviating oxidative stress (Duan et al., Citation2016; Leng et al., Citation2014; Lin et al., Citation2014). Meanwhile, dietary supplementations with Glu and Asp have been reported to enhance growth performance (Yin, Liu, et al., Citation2015), act as excitatory neurotransmitters in the brain (Wang, Wang, Xia, & Wood, Citation2014), and mediate feeding behaver (Khropycheva, Andreeva, Uneyama, Torii, & Zolotarev, Citation2011). Bin et al reported that 1% Asp lowered the ratio of Firmicutes: Bacteroidetes in mice and affected the innate immunity(Bin et al., Citation2017). Duan et al reported that 2% glutamate acted as a nutritional regulating factor to ameliorate the adverse effects of mycotoxins and improved growth performance in mycotoxins-challenged pigs (Duan et al., Citation2014) . Although various studies indicate that Glu and Asp protect piglets against different pathological conditions, such as oxidative stress and mycotoxin infection, little is known about the effects of different dietary contents of Glu and Asp on growth performance and amino acid metabolism in healthy piglets. Thus, the present study examined the amino acid metabolic responses to dietary Glu and Asp in piglets and growth performance was also focused.
Materials and methods
Animal and diets
All animal procedures were approved by the Committee on Animal Care of the Institute of Subtropical Agriculture, Chinese Academy of Sciences (Changsha, Hunan Province, China). Forty-two piglets (Duroc × Landrace × Yorkshire, male, 13.24 ± 0.25 kg) (Hunan New Wellful Co., Ltd., Hunan Province, China) were randomly assigned into one of six treatment groups (n = 7/group). Piglets in the control group (CG) were fed a basic diet containing 2.9% Glu and 1.5% Asp according to our previous study (Wu et al., Citation2015). Other piglets received 1.3% (LA) or 1.7% (HA) Asp and 2.6% (LG), 3.2% (HG), or 3.5% (HHG) Glu for 21 days, respectively (). Each animal was housed in a single cage. Piglets had free access to drinking water and diets during the experimental period.
Table 1. Composition and nutrient levels of experimental diets for piglets (air-dried basis, %).
Growth performance
During the experimental period, feed intake was recorded daily and all piglets were weighed individually at day 0 and 21. Average daily feed intake (ADFI), average daily weight gain (ADG), and the ratio of feed intake to weight gain (F/G) were calculated according to the feed consumption and weight of each piglet.
Amino acid determination
Blood samples were obtained from the mesenteric vein, hepatic portal vein, and anterior vena cava after anesthetic treatment using Zoletil 50 (Virbac S.A., France). Serum was prepared via centrifugation at 3000 g and 4°C for 10 min and stored at −80°C until analysis (Fang et al., Citation2017). Eighteen amino acids (Lys, Met, Thr, Trp, Glu, Asp, Val, Ile, Leu, Phe, Arg, Ser, His, Gly, Ala, Pro, Cys, and Tyr) in the serum were detected via 1260 liquid chromatograph (Agilent 1260) according to our previous reports (Duan et al., Citation2016; Liao et al., Citation2017).
Real-time quantitative (RT–PCR)
Total RNA from liver samples was isolated with TRIZOL reagent (Invitrogen, USA) and then treated with DNase I (Invitrogen, USA) (Meng et al., Citation2017; Reschke et al., Citation2017). Reverse transcription was performed at 37°C for 15 min, 95°C 5 sec. Primers used in this study were designed using Primer 5.0 according to the pig gene sequence (). β-actin was chosen as the house-keeping gene to normalize target gene levels. The PCR cycling conditions were 36 cycles at 94°C for 40 sec, 60°C for 30 sec and 72°C for 35 sec. Relative expression was expressed as a the ratio of the target gene to the control gene using the formula 2-(ΔΔCt), where ΔΔCt=(CtTarget-Ctβ-actin)treatment-(CtTarget-Ctβ-actin)control (Feng et al., Citation2015; Gupta et al., Citation2017; Lin et al., Citation2016; Sohail, Doran, Riedemann, Macaulay, & Southern, Citation2003). Relative expression was normalized and expressed relative to the expression in the control group according to our previous report (Gupta et al., Citation2017; Reschke et al., Citation2017; Yin et al., Citation2013; Yin et al., Citation2017).
Table 2. Primers used in this study.
Statistical analysis
All statistical analyses were performed using IBM SPSS 20 software. The data were performed by using the one-way analysis of variance (ANOVA) to test homogeneity of variances via Levene’s test and followed with student’s t test Data are expressed as mean ± SEM. Values in the same row with * are significant (P < 0.05)
Results
Effect of Asp and Glu on growth performance in piglets
Effect of Asp on growth performance was summarized in . There was no significant difference in body weight of piglets compared with control group (P > 0.05). Interestingly, ADG in the LA group was higher in the control group and F/G was markedly lower in the LA group (P < 0.05). However, dietary high Asp (1.7%) markedly decreased ADFI and ADG but increased F/G (P < 0.05), suggesting that low Asp improve growth but high dosage of Asp exert negative effect.
Table 3. Effects of Asp on growth performance in piglets.
Dietary supplementation with different dosages of Glu failed to affect body weight in piglets (P > 0.05; ). However, dietary high Glu significantly reduced ADG and increased F/G (P < 0.05). Moreover, ADFI in the HHG group was markedly lower than that in the control group (P < 0.05). These results suggested that excess Glu intake inhibit growth performance in piglets.
Table 4. Effects of Glu on growth performance in piglets.
Effect of dietary Asp on the circulating amino acids in piglets
The levels of amino acids in the mesenteric vein, hepatic portal vein, and anterior vena cava were determined to evaluate amino acid metabolic response to dietary amino acids. In the mesenteric pool, different doses of Asp in the diet failed to affect the levels of Asp and other amino acids (P > 0.05; ). In the hepatic portal, Trp and Tyr levels were significantly higher in the LA group than those in the control group (P < 0.05). In the anterior vena cava, Glu level in the HA group was markedly decreased compared with the control group (P < 0.05).
Table 5. Effect of dietary Asp on the circulating amino acids in piglets (μg/mL).
Effect of dietary Glu on the circulating amino acids in piglets
In the mesenteric pool, Met and Tyr were decreased in the HHG group, while Glu was increased in the HG group compared with the control group (P < 0.05; ). In the hepatic portal, Trp, Val, Ile, Leu, and Ser levels were higher in the HG group than those in the control group. Trp in HHG group and Ser in LG group were markedly increased (P < 0.05). In the anterior vena cava, Ile and Tyr were decreased in the LG group while His was increased compared with the control group (P < 0.05). Moreover, Glu and Tyr in the HG group were decreased (P < 0.05) and Ile, Leu, and Tyr in the HHG group were also decreased compared with control group (P < 0.05).
Table 6. Effect of dietary Glu on the circulating amino acids in piglets(μg/mL).
Liver expression of amino acid transporters
In this study, expressions of SLC1A1, SLC7A1, SLC7A7, and SLC6A19 were determined via RT–PCR (). Low-dose Asp down-regulated SLC6A19 expression in the liver (P < 0.05). Moreover, expressions of SLC7A1, SLC7A7 and SLC6A19 were markedly down-regulated in the HHG group (P < 0.05) mRNA in the liver. Also, dietary high Glu significantly down-regulated SLC6A19 expression compared with the control group (P < 0.05).
Figure 1. Effects of dietary Asp (A, B, C, D) and Glu (E, F, G, H) on amino acid transporters in the liver. Data are presented as mean ± SEM, n = 6. SLC1A1 solute carrier family 1 (neuronal/epithelial high affinity glutamate transporter, system XAG, member 1), SLC7A1 solute carrier family 7 (cationic amino acid transporter, y + system; member 1), SLC7A7 solute carrier family7 (amino acid transporter light chain, y + L system, member 7), and SLC6A19 solute carrier family 6 (neurotransmitter/neutral amino acid transporter, member 19).
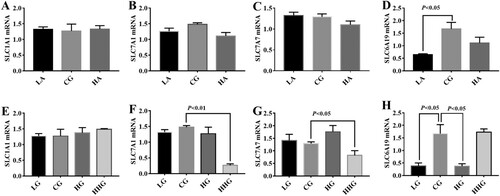
Discussion
Glu and Asp play important roles in the nervous system, immunity, and nutrition in animals and humans (Khropycheva et al., Citation2011; Leng, Citation2014; Lin et al., Citation2014; Wang et al., Citation2016; Yanni et al., Citation2010). Our previous studies showed that dietary supplementation with Asp and Glu reduced oxidative stress-induced growth suppression (Duan et al., Citation2016; Yin, Liu, et al., Citation2015). Shi et al. also reported that dietary 0.5% and 1% Asp improved ADG in piglets after exposure to lipopolysaccharide (Shi, Citation2013). Meanwhile, dietary 2% Glu attenuated mycotoxin-induced growth suppression by improving ADFI, ADWG, and the feed/weight ratio in piglets (Wu, Citation2014). The animals may change in diet and bring about poor growth performance under weaning stress (Li et al., Citation2017; Ren et al., Citation2018). Thus, we investigated dietary Asp and Glu in healthy piglets with initial weight 10 kg and pre-feeding for a week. In the current study indicated that dietary supplementation with high dosages of Asp and Glu reduced growth performance and diets with low levels of Asp or Glu improved growth performance in piglets. Together, we speculated that low doses of Asp and Glu may satisfy the nutritional requirements in healthy piglets, while supplementations with excess Asp and Glu exhibit a negative effect on growth performance under healthy conditions.
Serum amino acid levels are highly associated with animal health and growth performance (Li et al., Citation2016; Liao et al., Citation2017; Wu et al., Citation2014; Yin, Ren, et al., Citation2015). Previous studies have shown that dietary supplementation with 2.0% Glu, 0.5% −2.0% glutamine, 1.0% Asp or their combinations reduced oxidized stress or a pathological amino acid profile in weaned piglets (Duan et al., Citation2016; Ren et al., Citation2014). Moreover, Glu and Asp can transform alanine, citrulline, glutathione, ornithine and arginine and other precursor substances (Wu et al., Citation2007; Zhang et al., Citation2012). A portion of the free amino acids in the intestine are selectively absorbed into the mesenteric vein, and some amino acids, such as Glu and Asp, are used by the intestine to maintain intestinal health (Deutz, Bruins, & Soeters, Citation1998; Pi et al., Citation2014; Stoll & Burrin, Citation2006; Xi et al., Citation2012). Portal vein blood is collected by the stomach, intestine, spleen, and other organs (extracorporeal tissue, Portal-Drained Viscera, PDV) (Stoll et al., Citation1998). Studies have shown that the liver contains high levels of Glu and Asp under low crude protein diets (17% crude protein) (Zheng, Citation2015). Meanwhile, Glu and Asp are absorbed and oxidized in the intestine and liver to maintain the intestinal and liver structure and function (Jinap & Hajeb, Citation2010; Leng et al., Citation2014; Wang et al., Citation2015; Wang et al., Citation2017). In the current work, only Glu levels in the anterior chamber of the HA and HG group were markedly decreased. The reason may be explained by that low doses of Glu and Asp meet the needs of the body, thus extra Glu and Asp may be oxidized by intestinal epithelial cells and liver cells to provide energy.
Zhenyukh et al. found that pathological conditions increased plasma levels of branched chain amino acids (Zhenyukh et al., Citation2017). In this study, the hepatic portal vein in the HG group (3.2% Glu group) contained the highest levels of branched chain amino acids, which may explain why 3.2% Glu appeared to have a negative effect on growth performance. Try is a precursor of the neurotransmitter serotonin, which promotes feed intake (Burgoon, Knabe, & Gregg, Citation1992; Eder, Nonn, Kluge, & Peganova, Citation2003; Kendall, Gaines, Kerr, & Allee, Citation2007), reduces energy waste, and enhances feed utilization (Zhang et al., Citation2013). Meanwhile, tryptophan inhibits xanthine oxidase activity and reactive oxygen, as well as oxidative stress (Lv et al., Citation2012). The current study showed that the portal vein of the LA, HG, and HHG group showed a high tryptophan level, which might affect growth performance and amino acid metabolism and detailed mechanisms should be further investigated.
Amino acids are mainly sensed and transported by specific amino acid transporters (Burckhardt Birgitta & Burckhardt, Citation2017; Yin et al., Citation2017; Zuo et al., Citation2015). For example, SLC1A1 transports Asp and Glu and SLC7A1 contributes to Lys, Arg, and His uptake (Verrey, Meier, Rossier, & Kuhn, Citation2000). In this study, high dosage of Glu downregulated SLC7A1, SLC7A1, and SLC6A19 expressions, which may be associated with the feedback regulation as excess of amino acids are toxic to cells. SLC6A19 transports all neutral amino acids and is the only transporter of Trp in the small intestine (Bröer et al., Citation2004). Thus, we speculated that SLC6A19 may mediate Trp metabolism in this study.
Conclusions
Low levels of Glu and Asp in the diet increased growth performance of piglets and the serum tryptophan level. High doses of Glu and Asp, especially 3.2% Glu, inhibited the growth of piglets, increased BCAA levels in the hepatic portal vein and may have toxicological effects.
Acknowledgements
We would like to thank Yao Jiming, Fang Wenjun, and Chen Yuquan from Guangdong Wangda group for the animal feeding support. We are grateful to the Public Service Technology Center, Institute of Subtropical Agriculture, Chinese Academy of Sciences for technical support.
Disclosure statement
No potential conflict of interest was reported by the authors.
Additional information
Funding
References
- Bin, P., Liu, S., Chen, S., Zeng, Z., Huang, R., Yin, Y., & Liu, G. (2017). The effect of aspartate supplementation on the microbial composition and innate immunity on mice. Amino Acids, 49, 2045–2051. doi: https://doi.org/10.1007/s00726-017-2467-5
- Bröer, A., Klingel, K., Kowalczuk, S., Rasko, J. E., Cavanaugh, J., & Bröer, S. (2004). Molecular cloning of mouse amino acid transport system B0, a neutral amino acid transporter related to Hartnup disorder. Journal of Biological Chemistry, 279(23), 24467–24476. doi: https://doi.org/10.1074/jbc.M400904200
- Burckhardt Birgitta, C., & Burckhardt, G. (2017). Interaction of excitatory amino acid transporters 1–3 (EAAT1, EAAT2, EAAT3) with N-carbamoylglutamate and N-acetylglutamate. Cellular Physiology & Biochemistry, 43(5), 1907–1916. doi: https://doi.org/10.1159/000484110
- Burgoon, K. G., Knabe, D. A., & Gregg, E. J. (1992). Digestible tryptophan requirements of starting, growing, and finishing pigs. Journal of Animal Science, 70(8), 2493–2500. doi: https://doi.org/10.2527/1992.7082493x
- Deutz, N. E., Bruins, M. J., & Soeters, P. B. (1998). Infusion of soy and casein protein meals affects interorgan amino acid metabolism and urea kinetics differently in pigs. Journal of Nutrition, 128(12), 2435–2445. doi: https://doi.org/10.1093/jn/128.12.2435
- Duan, J., Yin, J., Ren, W., Liu, T., Cui, Z., Huang, X., … Yin, Y. (2016). Dietary supplementation with L-glutamate and L-aspartate alleviates oxidative stress in weaned piglets challenged with hydrogen peroxide. Amino Acids, 48(1), 53–64. doi: https://doi.org/10.1007/s00726-015-2065-3
- Duan, J., Yin, J., Wu, M., Liao, P., Deng, D., Liu, G., … Yin, Y. (2014). Dietary glutamate supplementation ameliorates mycotoxin-induced abnormalities in the intestinal structure and expression of amino acid transporters in young pigs. PloS one, 9(11), e112357. doi: https://doi.org/10.1371/journal.pone.0112357
- Eder, K., Nonn, H., Kluge, H., & Peganova, S. (2003). Tryptophan requirement of growing pigs at various body weights. Journal of Animal Physiology and Animal Nutrition, 87(9–10), 336–346. doi: https://doi.org/10.1046/j.1439-0396.2003.00442.x
- Fang, J., Martínez, Y., Deng, C., Zhu, D., Peng, H., Jiang, H., & Li, A. (2017). Effects of dietary enzymolysis products of wheat gluten on the growth performance, serum biochemical, immune, and antioxidant status of broilers. Food and Agricultural Immunology, 57, 1–13. doi: https://doi.org/10.1080/09540105.2017.1332009
- Feng, Z., Li, T., Wu, C., Tao, L., Blachier, F., & Yin, Y. (2015). Monosodium L-glutamate and dietary fat exert opposite effects on the proximal and distal intestinal health in growing pigs. Applied Physiology, Nutrition, and Metabolism, 40(4), 353–363. doi: https://doi.org/10.1139/apnm-2014-0434
- Gupta, A., Quijano, E., Liu, Y., Bahal, R., Scanlon, S. E., Song, E., … Saltzman, W. M. (2017). Anti-tumor activity of miniPEG-γ-modified PNAs to inhibit microRNA-210 for cancer therapy. Molecular Therapy - Nucleic Acids, 9, 111–119. doi: https://doi.org/10.1016/j.omtn.2017.09.001
- Jinap, S., & Hajeb, P. (2010). Glutamate. Its applications in food and contribution to health. Appetite, 55(1), 1–10. doi: https://doi.org/10.1016/j.appet.2010.05.002
- Kendall, D. C., Gaines, A. M., Kerr, B. J., & Allee, G. L. (2007). True ileal digestible tryptophan to lysine ratios in ninety- to one hundred twenty-five-kilogram barrows. Journal of Animal Science, 85(11), 3004–3012. doi: https://doi.org/10.2527/jas.2007-0013
- Khropycheva, R., Andreeva, J., Uneyama, H., Torii, K., & Zolotarev, V. (2011). Dietary glutamate signal evokes gastric juice excretion in dogs. Digestion, 83(Suppl 1), 7–12. doi: https://doi.org/10.1159/000323398
- Leng, W. (2014). Regulative effect of asparetic acid on liver injury and muscle protein degradation of piglets after lipopolysaccharide challenge. Wuhan University of Light Industry.
- Leng, W., Liu, Y., Shi, H., Li, S., Zhu, H., Pi, D., … Gong, J. (2014). Aspartate alleviates liver injury and regulates mRNA expressions of TLR4 and NOD signaling-related genes in weaned pigs after lipopolysaccharide challenge. The Journal of Nutritional Biochemistry, 25(6), 592–599. doi: https://doi.org/10.1016/j.jnutbio.2014.01.010
- Li, L., Chen, S., Li, X., Wan, D., Liu, G., Liu, Y., … Yin, Y. (2017). Intestinal microbiota in growing pigs: Effects of stocking density. Food and Agricultural Immunology, 28, 1–12. doi:10.1080/09540105.2017.1409195 doi: https://doi.org/10.1080/09540105.2016.1202206
- Li, Y., Wei, H., Li, F., Chen, S., Duan, Y., Guo, Q., … Yin, Y. (2016). Supplementation of branched-chain amino acids in protein-restricted diets modulates the expression levels of amino acid transporters and energy metabolism associated regulators in the adipose tissue of growing pigs. Animal Nutrition, 2(1), 24–32. doi: https://doi.org/10.1016/j.aninu.2016.01.003
- Liao, P., Li, M., Li, Y., Tan, X., Zhao, F., Shu, X., & Yin, Y. (2017). Effects of dietary supplementation with cupreous N-carbamylglutamate (NCG) chelate and copper sulfate on growth performance, serum biochemical profile and immune response, tissue mineral levels and fecal excretion of mineral in weaning piglets. Food and Agricultural Immunology, 112, 1–15. doi: https://doi.org/10.1080/09540105.2017.1339668
- Lin, A. H., Twitty, C. G., Burnett, R., Hofacre, A., Mitchell, L. A., Espinoza, F. L., … Jolly, D. J. (2016). Retroviral replicating vector delivery of miR-PDL1 inhibits immune checkpoint PDL1 and enhances immune responses in vitro. Molecular Therapy Nucleic Acids, 6(C), 221–232.
- Lin, M., Zhang, B., Yu, C., Li, J., Zhang, L., Sun, H., … Zhou, G. (2014). L-Glutamate supplementation improves small intestinal architecture and enhances the expressions of jejunal mucosa amino acid receptors and transporters in weaning piglets. PloS one, 9(11), e111950. doi: https://doi.org/10.1371/journal.pone.0111950
- Lv, M., Yu, B., Mao, X. B., Zheng, P., He, J., & Chen, D. W. (2012). Responses of growth performance and tryptophan metabolism to oxidative stress induced by diquat in weaned pigs. animal, 6(6), 928–934. doi: https://doi.org/10.1017/S1751731111002382
- Meng, Z., Gao, P., Chen, L., Peng, J., Huang, J., Wu, M., … Zhou, Z. (2017). Artificial zinc-finger transcription factor of A20 suppresses restenosis in sprague dawley rats after carotid injury via the PPARα pathway. Molecular Therapy - Nucleic Acids, 8, 123–131. doi: https://doi.org/10.1016/j.omtn.2017.06.010
- Pi, D., Liu, Y., Shi, H., Li, S., Odle, J., Lin, X., … Leng, W. (2014). Dietary supplementation of aspartate enhances intestinal integrity and energy status in weanling piglets after lipopolysaccharide challenge. The Journal of Nutritional Biochemistry, 25(4), 456–462. doi: https://doi.org/10.1016/j.jnutbio.2013.12.006
- Ren, W., Wang, P., Yan, J., Liu, G., Zeng, B., Hussain, T., … Yin, Y. (2018). Melatonin alleviates weanling stress in mice: Involvement of intestinal microbiota. Journal of Pineal Research, 64, e12448. doi: https://doi.org/10.1111/jpi.12448
- Ren, W., Yin, J., Wu, M., Liu, G., Yang, G., Xion, Y., … Wu, G. (2014). Serum amino acids profile and the beneficial effects of L-arginine or L-glutamine supplementation in dextran sulfate sodium colitis. PloS one, 9(2), e88335. doi: https://doi.org/10.1371/journal.pone.0088335
- Reschke, C. R., Silva, L. F. A., Norwood, B. A., Senthilkumar, K., Morris, G., Sanz-Rodriguez, A., … Bauer, S. (2017). Potent anti-seizure effects of locked nucleic acid antagomirs targeting miR-134 in multiple mouse and rat models of epilepsy. Molecular Therapy - Nucleic Acids, 6(C), 45–56. doi: https://doi.org/10.1016/j.omtn.2016.11.002
- Shi, H. (2013). Regulatory effect of aspartic acid on intestinal injury in piglets after lipopolysaccharide challenge. Wuhan University of Light Industry.
- Sohail, M., Doran, G., Riedemann, J., Macaulay, V., & Southern, E. M. (2003). A simple and cost-effective method for producing small interfering RNAs with high efficacy. Nucleic Acids Research, 31(7), e38. doi: https://doi.org/10.1093/nar/gng038
- Stoll, B., & Burrin, D. G. (2006). Measuring splanchnic amino acid metabolism in vivo using stable isotopic tracers. Journal of Animal Science, 84(13_suppl), E60–E72. doi: https://doi.org/10.2527/2006.8413_supplE60x
- Stoll, B., Henry, J., Reeds, P. J., Yu, H., Jahoor, F., & Burrin, D. G. (1998). Catabolism dominates the first-pass intestinal metabolism of dietary essential amino acids in milk protein-fed piglets. Journal of Nutrition, 128(3), 606–614. doi: https://doi.org/10.1093/jn/128.3.606
- Verrey, F., Meier, C., Rossier, G., & Kuhn, L. C. (2000). Glycoprotein-associated amino acid exchangers: Broadening the range of transport specificity. Pflügers Archiv - European Journal of Physiology, 440(4), 503–512. doi: https://doi.org/10.1007/s004240000274
- Wang, L., Hou, Y., Yi, D., Li, Y., Ding, B., Zhu, H., … Wu, G. (2015). Dietary supplementation with glutamate precursor α-ketoglutarate attenuates lipopolysaccharide-induced liver injury in young pigs. Amino Acids, 47(7), 1309–1318. doi: https://doi.org/10.1007/s00726-015-1966-5
- Wang, H., Liu, Y., Shi, H., Wang, X., Zhu, H., Pi, D., … Li, S. (2016). Aspartate attenuates intestinal injury and inhibits TLR4 and NODs/NF-κB and p38 signaling in weaned pigs after LPS challenge. European Journal of Nutrition, 56(4), 1433–1443. doi: https://doi.org/10.1007/s00394-016-1189-x
- Wang, H., Liu, Y., Shi, H., Wang, X., Zhu, H., Pi, D., … Li, S. (2017). Aspartate attenuates intestinal injury and inhibits TLR4 and NODs/NF-kappaB and p38 signaling in weaned pigs after LPS challenge. European Journal of Nutrition, 56, 1433–1443. doi: https://doi.org/10.1007/s00394-016-1189-x
- Wang, G. D., Wang, X. Y., Xia, Y., & Wood, J. D. (2014). Dietary glutamate: Interactions with the enteric nervous system. Journal of Neurogastroenterology and Motility, 20(1), 41–53. doi: https://doi.org/10.5056/jnm.2014.20.1.41
- Wu, G. (2010). Functional amino acids in growth, reproduction, and health. Advances in Nutrition: An International Review Journal, 1(1), 31–37. doi: https://doi.org/10.3945/an.110.1008
- Wu, M. (2014). Regulation of glutamate supplementation on intestinal stress in piglets infected with vomit toxin. Graduate School of Chinese Academy of Sciences.
- Wu, G., Bazer, F. W., Dai, Z., Li, D., Wang, J., & Wu, Z. (2014). Amino acid nutrition in animals: Protein synthesis and beyond. Annual Review of Animal Biosciences, 2, 387–417. doi: https://doi.org/10.1146/annurev-animal-022513-114113
- Wu, G., Bazer, F. W., Davis, T. A., Jaeger, L. A., Johnson, G. A., Kim, S. W., … Yin, Y.-L. (2007). Important roles for the arginine family of amino acids in swine nutrition and production. Livestock Science, 112(1–2), 8–22. doi: https://doi.org/10.1016/j.livsci.2007.07.003
- Wu, L., He, L. Q., Cui, Z. J., Liu, G., Yao, K., Wu, F., … Li, T. J. (2015). Effects of reducing dietary protein on the expression of nutrition sensing genes (amino acid transporters) in weaned piglets. Journal of Zhejiang University Science B, 16(6), 496–502. doi: https://doi.org/10.1631/jzus.B1400259
- Xi, P., Jiang, Z., Dai, Z., Li, X., Yao, K., Zheng, C., … Wu, G. (2012). Regulation of protein turnover by L-glutamine in porcine intestinal epithelial cells. The Journal of Nutritional Biochemistry, 23(8), 1012–1017. doi: https://doi.org/10.1016/j.jnutbio.2011.05.009
- Yanni, A. E., Agrogiannis, G., Nomikos, T., Fragopoulou, E., Pantopoulou, A., Antonopoulou, S., & Perrea, D. (2010). Oral supplementation with L-aspartate and L-glutamate inhibits atherogenesis and fatty liver disease in cholesterol-fed rabbit. Amino Acids, 38(5), 1323–1331. doi: https://doi.org/10.1007/s00726-009-0340-x
- Yin, J., Han, H., Li, Y., Liu, Z., Zhao, Y., Fang, R., … Wu, F. (2017). Lysine restriction affects feed intake and amino acid metabolism via gut microbiome in piglets. Cellular Physiology and Biochemistry, 44(5), 1749–1761. doi: https://doi.org/10.1159/000485782
- Yin, J., Li, Y., Han, H., Zheng, J., Wang, L., Ren, W., … Yin, Y. (2017). Effects of lysine deficiency and Lys-Lys dipeptide on cellular apoptosis and amino acids metabolism. Molecular Nutrition & Food Research, 61, 1600754. doi: https://doi.org/10.1002/mnfr.201600754
- Yin, J., Liu, M., Ren, W., Duan, J., Yang, G., Zhao, Y., … Yin, Y. (2015). Effects of dietary supplementation with glutamate and aspartate on diquat-induced oxidative stress in piglets. PloS one, 10(4), e0122893. doi: https://doi.org/10.1371/journal.pone.0122893
- Yin, J., Ren, W., Hou, Y., Wu, M., Xiao, H., Duan, J., … Nyachoti, C. M. (2015). Use of homoarginine for measuring true ileal digestibility of amino acids in food protein. Amino Acids, 47(9), 1795–1803. doi: https://doi.org/10.1007/s00726-015-1958-5
- Yin, J., Ren, W., Liu, G., Duan, J., Yang, G., Wu, L., … Yin, Y. (2013). Birth oxidative stress and the development of an antioxidant system in newborn piglets. Free Radical Research, 47(12), 1027–1035. doi: https://doi.org/10.3109/10715762.2013.848277
- Zhang, S., Qiao, S., Ren, M., Zeng, X., Ma, X., Wu, Z., … Wu, G. (2013). Supplementation with branched-chain amino acids to a low-protein diet regulates intestinal expression of amino acid and peptide transporters in weanling pigs. Amino Acids, 45(5), 1191–1205. doi: https://doi.org/10.1007/s00726-013-1577-y
- Zhang, J., Yin, Y., He, Q., Chen, G., Li, T., Wu, X., … Wen, Q. (2012). Effects of MSG supplementation on free amino acids in plasma of growing-finishing pigs. Journal of Food Agriculture & Environment, 10(3–4), 600–605.
- Zheng, P. (2015). The effects of different levels of protein diets on the amino acid metabolism in the piglet liver. Huazhong agricultural university.
- Zhenyukh, O., Civantos, E., Ruiz-Ortega, M., Sanchez, M. S., Vazquez, C., Peiro, C., … Mas, S. (2017). High concentration of branched-chain amino acids promotes oxidative stress, inflammation and migration of human peripheral blood mononuclear cells via mTORC1 activation. Free Radical Biology & Medicine, 104, 165–177. doi: https://doi.org/10.1016/j.freeradbiomed.2017.01.009
- Zuo, J., Ling, B., Long, L., Li, T., Lahaye, L., Yang, C., & Feng, D. (2015). Effect of dietary supplementation with protease on growth performance, nutrient digestibility, intestinal morphology, digestive enzymes and gene expression of weaned piglets. Animal Nutrition, 1(4), 276–282. doi: https://doi.org/10.1016/j.aninu.2015.10.003