ABSTRACT
Polybrominated diphenyl ethers are contaminants that are widely distributed in the environment and in food samples. In this study, a highly sensitive biotin–streptavidin system-based real-time immuno-polymerase chain reaction assay (BA-IPCR) was developed to detect 2,2′,4,4′-tetrabromodiphenyl ether (BDE-47). The BDE-47 immunogen and coating antigen were prepared, and a specific polyclonal anti-BDE-47 antibody was obtained. Several physiochemical factors in the immunoassay were optimised. The established BA-IPCR method was used to detect BDE-47 with a linearity ranging from 10 pg L−1 to 100 ng L−1. The limit of detection was 2.96 pg L−1. The recovery of the spiked samples was 92.97–107.19% and the coefficient of variation was 6.93–11.06%. The proposed method was applied to successfully detect BDE-47 in marine fish. The BA-IPCR data for the detection of BDE-47 were consistent with gas chromatography–mass spectrometry findings. The BA-IPCR method is accurate, sensitive and suitable for detecting trace amount of BDE-47 in food samples.
Introduction
Polybrominated diphenyl ethers (PBDEs) are a class of brominated flame retardants that exist extensively in a wide variety of products, including building materials, electronic and electrical devices, textiles, furnishings and plastics (Król, Zabiegała, & Namieśnik, Citation2012). Commercial mixtures of PBDE are mainly used in the manufacturing processes. Because of their persistent nature, the emitted PBDEs are widely distributed into the environment i.e. air, water and soil. Due to their long-range transport, PBDEs were detected even in the Arctic environment. PBDEs can accumulate in the biological tissues (Cloutier et al., Citation2017) and also found out in a variety of biota matrices (Lee & Kim, Citation2015; Tanaka et al., Citation2015). For example, in the United States, PBDE concentrations in pregnant, predominantly immigrant women were associated with stuffed furniture in the home and increased with time (Castorina et al., Citation2011). Several studies have shown that PBDE concentrations in serum of children are positively associated with the duration of breastfeeding and the maternal serum during pregnancy (Bradman et al., Citation2012; Stapleton, Eagle, Sjödin, & Webster, Citation2012).
Recent studies have investigated the occurrence of PBDEs in food, and the exposure of the general population to PBDEs has been estimated. In Italy, among various food samples, fish oil and milk products are found to be the predominant contributors to the intake of PBDEs (Martellini et al., Citation2016), while in China, meat products are the predominant source of PBDEs (Gong et al., Citation2015). Eggs are another type of food items that can result in high human exposure to PBDEs when consumed (Shang et al., Citation2016). 2,2′,4,4′-tetrabromodiphenyl ether (BDE-47), one of the major components of commercial penta-BDE mixtures (La Guardia, Hale, & Harvey, Citation2006), is usually selected as the model congener because of its wide distribution in the environment and in food samples. Food analyses in China have revealed that BDE-47 occurs most frequently among the PBDE congeners. Moreover, several studies have shown that it is easy to enrich fish with BDE-47 and this has been used as an important indicator of PBDE pollution in marine fish (Hites, Citation2004). A wide variety of marine fish commonly form part of the die for people living in China. As BDE-47 is likely to accumulate in organisms, BDE-47 accumulation in fish can ultimately affect human health through the food chain. Therefore, it is critical to establish highly sensitive and high-throughput techniques for detecting BDE-47 in marine fish.
Several instrumental analysis techniques are commonly applied to detect BDE-47 in food samples, such as gas chromatography–mass spectrometry (GC–MS) and high-performance liquid chromatography (HPLC) (Thorenz, Musa Bandowe, Sobocka, & Wilcke, Citation2010; Lacorte, Ikonomou, & Fischer, Citation2010). Although reliable and effective for the analysis of pollutants, the instrumental analyses take an extended period of time and the follow-up maintenance of the equipment is expensive (Wang & Li, Citation2010; Covaci et al., Citation2011; Ionas et al., Citation2015; Martellini et al., Citation2016; Troisi & Borjesson, Citation2005). Nowadays, some immunoassays that are applied for the monitoring of trace pollutants are highly sensitive and specific (Zhuang, Gao, Gao, & Fan, Citation2012). Several studies have described the development and characterisation of colorimetric immunoassays for the detection of BDE-47 with chemiluminescence or fluorescence (Ahn et al., Citation2009; Shelver et al., Citation2008).
Real-time immuno-polymerase chain reaction (rt-IPCR) is an immunoassay based on the chimeric conjugates of specific antibodies and DNA. In this assay, DNA is amplified for a particular signal generation, thereby dramatically enhanced the sensitivity of the immunoassay. The limit of detection (LOD) of the rt-IPCR assay is usually 100–10,000 times lower than that of enzyme-linked immunosorbent assay (ELISA) (Sano, Smith, & Cantor, Citation1992). Given its high sensitivity and good capability of quantification, the rt-IPCR assay can be used for the sensitive detection of analytes. For example, Chen developed the functionalised gold nanoparticles-based real-time immuno-PCR assay for detecting Hantaan virus nucleocapsid protein with a detection limit of 10 pg L−1 (Chen et al., Citation2009). Owing to the versatility and high affinity of the biotin–streptavidin binding system, the biotin–streptavidin-based real-time immuno-PCR (BA-IPCR) can eliminate the necessity of cumbersome synthesis and purification for antibody-DNA conjugates, and has high sensitivity and specificity (Niemeyer, Adler, & Wacker, Citation2005). The BA-IPCR assay has a large variety of novel applications in the detection of biomolecules in medicine (Lind & Kubista, Citation2005). However, there are fewer applications of the BA-IPCR method for the monitoring of environment and food contaminations. Moreover, to our knowledge, no information about the detection of BDE-47 in food samples using the BA-IPCR assay has been reported.
In the present study, a highly sensitive real-time immuno-polymerase chain reaction method using the biotin–streptavidin system was developed for detecting BDE-47. Two diverse BDE-47 antigens (immunogen and coating antigen) were prepared, and the polyclonal anti-BDE-47 antibody (pAb-BDE-47) was obtained. Several types of marine fishes that are prevalent in Chinese diet were collected from the East China Sea and tested using the developed BA-IPCR method. The stability of the BA-IPCR method was investigated. The detection results of the immunoassay were consistent with those obtained by GC–MS. The principle of the BA-IPCR assay is shown in . To begin, the surface of PCR tubes is coated with coating antigens. Then, the biotinylated pAb-BDE-47 (bio-pAb-BDE-47) and BDE-47 samples are added, BDE-47 analytes compete with coating antigens to bind with bio-pAb-BDE-47. With the increasing BDE-47 concentration, the amount of bio-pAb-BDE-47 binding with coating antigens decreases. As a result, the number of template DNA on the solid phase carrier also decreases; less target DNA is released and more amplification cycles are needed to reach the fluorescent threshold in the PCR analysis. Thus, BDE-47 samples can be quantified by the cycle number.
Experimental
Reagent and solutions
BDE-47 standard and the chemicals for BDE-47 hapten synthesis were purchased from J&K Chemical Co. Ltd. (Beijing, China). Bovine serum albumin (BSA), concentrated hydrochloric acid, egg albumin (OVA), 25% glutaraldehyde solution, dimethylsulfoxide (DMSO), N,N-dimethylformamide (DMF), inorganic salts, gelatin, urea, Coomassie brilliant blue G250, Tween 20, incomplete Freund’s adjuvant (IFA) and complete Freund’s adjuvant (CFA) were purchased from Sinopharm Chemical Reagent Co. Ltd. (Shanghai, China). Streptavidin, biotinylated N-hydroxysuccinimide ester (BNHS), SYBR Green I PCR mixture were all obtained from Sangon Co. Ltd. (Shanghai, China). The nucleotide sequences of biotinylated double-stranded DNA (bio-DNA) are 5′-Bio-AAGCTTGCATGCCTGCAGGTCGACTCTAGAGGATCCCCGGGTACCGA GCTCGAATTC-3′ and 5′-Bio-GAATTCGAGCTCGGTACCCGGGGATCCTCTAG AGTCGACCTGCAGGCATGCAAGCTT-3′ respectively. The nucleotide sequences of forward primer and reverse primer are 5′-GTAAAACGACGGCCAGT-3′ and 5′-CAGGAAACAGCTATGAC-3′, respectively. The bio-DNA and primers were all synthesised by Sangon Co. Ltd. (Shanghai, China).
Carbonate buffer solution (CBS: Na2CO3 [15 mmol L−1] and NaHCO3 [34.9 mmol L−1], pH 9.60), borate buffer (BB: Na2B4O7 [10 mmol L−1], pH 9.16), phosphate-buffer saline (PBS: NaCl [137 mmol L−1], KCl [2.7 mmol L−1], Na2HPO4 [10 mmol L−1], and KH2PO4 [2 mmol L−1], pH 7.40) and PBS with 0.05% Tween 20 (PBST, pH 7.40) were used in the immunoassay.
Instruments and apparatus
The nuclear magnetic resonance (NMR) spectrometer was an Avance III 400-MHz instrument (Bruker, Inc., Switzerland) with CDCl3 solution. Mass spectrometry analysis was performed on an LC-MS 2010 mass spectrometer (Shimadzu Scientific Instruments, Inc., Japan). Ultrapure water was prepared using a Milli-Q System (Millipore, Bedford, MA, USA). Quantitative real-time PCR was performed with the StepOne Plus Real-Time PCR System (Applied Biosystems, America). Ultraviolet-visible (UV-vis) spectra was obtained on UV-7504 PC spectrophotometer (Shanghai Xin Mao Instrument, Inc., China). PCR tubes were purchased from Applied Biosystems, USA. Cross-reactivity test was completed using a Multiskan photometer purchased from Thermo Labsystems (Vantaa, Finland). GC–MS analysis was performed on a GC–MS QP 2010 gas chromatography and mass spectrometer (Shimadzu Scientific Instruments, Inc., Japan).
Synthesis of BDE-47 hapten
As BDE-47 molecule does not contain functional groups that can connect with proteins, BDE-47 hapten should be synthesised (Shelver et al., Citation2005). The reaction route was in accordance to the method described by Wei (Shelver et al., Citation2005). The specific synthetic route is illustrated in .
The mass spectrum of BDE-47 hapten is shown in . The 1H-NMR spectrum and the 13C-NMR spectrum of BDE-47 hapten are shown in . BDE-47 hapten (4-amino-2,2′,4′-tribromodiphenyl ether, BDE-47-NH2): C12H8NOBr3, m.w.: 421.914, yield: 61.9%. MS (GC–MS, 70 eV): 186 (M+-PhBr2), 188 (M++2-PhBr2), 261 (M+-2Br), 263 (M++2-2Br), 419 (M+), 421 (M++2), 423 (M++4), 425 (M++6). 1H-NMR (δ, CDCl3) 3.75 (br s, 2H), 6.45(d, J = 9.2 Hz, 1H), 6.60 (d, J = 8.4 Hz, 1H), 6.80 (d, J = 8.4 Hz, 1H), 6.90 (d, J = 2.0 Hz, 1H), 7.35 (s, 1H), 7.80 (s, 1H); 13C-NMR (δ, CDCl3) 113.7, 115.2, 115.6, 116.7, 117.9, 120.0, 123.4, 131.8, 136.1, 136.3, 145.3, 155.1.
Preparation of BDE-47 immunogen and coating antigen
In order to reduce the specific binding caused by the linking arm (the contact portion between the carrier protein and the BDE-47 hapten) (Goodrow & Hammock, Citation1998), the hapten-protein conjugates OVA-BDE-47 (coating antigen) and BSA-BDE-47 (immunogen) were prepared by the glutaraldehyde method and the diazotisation method, respectively ().
First, 0.042 g (0.1 mmol) BDE-47 hapten was dissolved in 1 mL of DMF and added to OVA solution (15 mg mL−1, in PBS). Then, 25% glutaraldehyde solution (100 µL) was added to the mixture and allowed to react with stirring for 24 h at 4°C. After the centrifugation for 15 min at 5000 rpm and 4°C, the supernatant was dialysed against PBS for three days. The BDE-47 coating antigen (OVA-BDE-47) was stored at −20°C. 0.042 g (0.1 mmol) BDE-47 hapten was added in 0.75 mL of double distilled water (ddH2O) while stirring. Then, 0.025 mL of HCl was added and allowed to react with stirring for 20 min under heating, and the mixture was cooled in an ice bath. Afterward, 1 mol L−1 sodium nitrite solution (200 µL) was added. After another reaction, while stirring for 1 h at 4°C, 0.5 g urea was added to remove the excess sodium nitrite. After the centrifugation for 15 min at 5000 rpm and 4°C, the supernatant was added to BSA solution (15 mg mL−1, in BB) until the mixture became orange. Then, the mixture was allowed to react for 2 h at 4°C, and the reaction solution was dialysed against PBS for three days. The BDE-47 immunogen (BSA-BDE-47) was stored at −20°C.
Both OVA-BDE-47 and BSA-BDE-47 were identified using an UV-vis spectrophotometer (). The characteristic peaks were observed at 312 nm for BDE-47 hapten; 227 and 278 nm for BSA; and 230, 236 and 279 nm for OVA. The characteristic peaks for OVA-BDE-47 and BSA-BDE-47 were observed at 368 and 358 nm, respectively. There were relationships and differences among the characteristic peaks of BDE-47 hapten, proteins and conjugates. The results showed that the coating antigen and immunogen were prepared successfully. Measured by the Coomassie brilliant blue assay, the coating antigen and immunogen concentrations were 2.10 and 3.39 mg mL−1, respectively.
Preparation of bio-pAb-BDE-47
The polyclonal anti-BDE-47 antibody was prepared by immunising a healthy rabbit with BSA-BDE-47 through subcutaneous and intramuscular injections. The initial immunisation was performed by injecting 1 mg of BSA-BDE-47 dissolved in 0.5 mL of normal saline and emulsified with 0.5 mL of CFA. After three weeks, the rabbit was boosted six times by injecting 1 mg of BSA-BDE-47 dissolved in 0.5 mL of normal saline and emulsified with 0.5 mL of IFA at two-week intervals. After the antiserum titer surpassed 64,000 determined by the indirect competitive ELISA method, the pAb-BDE-47 was separated and purified from rabbit serum, and then stored at −20°C. The pAb-BDE-47 concentration was 3.51 mg mL−1.
The bio-pAb-BDE-47 was prepared as follows: 5.0 mg of pAb-BDE-47 was dissolved in CBS buffer at a concentration of 1.0 mg mL−1. The solution was mixed with BNHS (1 mg mL−1, in DMSO) in the mass ratio of 1:10. The mixture was allowed to react with stirring for 4 h and then dialysed against PBS for three days. Afterwards, the small amount of precipitation was removed; the bio-pAb-BDE-47 was obtained and stored at −20°C. The bio-pAb-BDE-47 was identified by the UV-vis spectrophotometer (). The characteristic peaks were observed at 265 nm for BNHS; 208 and 279 nm for pAb-BDE-47; and 216 and 282 nm for bio-pAb-BDE-47. The results revealed that the BNHS was conjugated with the pAb-BDE-47 successfully.
Preparation of BDE-47 samples
The marine fish samples, including codfish (C1, C2, C3, C4), yellow croaker (Y1, Y2, Y3, Y4), pomfret (P1, P2, P3, P4) and Spanish mackerel (S1, S2, S3, S4) were provided by East China Sea Fisheries Research Institute. All of the fish samples were collected from the East China Sea and stored at −20°C. The pretreatment of the samples was performed following the procedure described by Liu et al. (Citation2011) with slight modification. First, the muscle tissue of the marine fish was stripped, homogenised and freeze-dried. Next, 2 g of the fish sample was extracted with hexane/methylene chloride (1/1, [v/v]) using the pressurised liquid extraction-ASE 300 system (Dionex, Sunnyvale, CA, USA). The bulk lipids were dissolved in hexane and removed by stirring for 20 min with acid-modified silica gel (44% H2SO4, w/w) in a water bath at 60°C. Then, the extract liquid was dried with Na2SO4 and concentrated down to approximately 3 mL. A chromatographic column filled with acidified silica, alumina and silica gel was used for further purification (leachate:hexane/methylene chloride = 1/1 [v/v]). The leachate was collected and evaporated to near dryness and then dissolved in 1 mL of hexane. The treated samples were divided into two fractions: one is for the BA-IPCR method after being diluted 1000 times with PBS containing 5% DMSO before analysis, and the other is for the GC–MS analysis.
The GC column was a DB-5MS fused silica capillary column (30 m × 0.25 mm × 0.10 µm), and helium was used as the carrier gas (purity > 99.999%, flow-rate: 2.0 mL min−1). The injection volume was 1 µL. The column temperature was held at 150°C for 2 min then programmed at a rate of 20°C min−1 to 320°C, and held at 320°C for 18 min.
Establishment of the BA-IPCR method
PCR tubes were pre-treated with 0.8% glutaraldehyde solution to improve the adsorbability (Xu, Xiao, & Ju, Citation1999). After washing three times with PBST and three times with ddH2O, the PCR tubes were coated with 20 µL/tube OVA-BDE-47 dilution overnight at 4°C. After washing three times with PBST, the unbound sites were blocked with 200 µL/tube blocking reagent and incubated for 1 h at 37°C. After an additional washing step (three times with PBST), 10 µL/tube of BDE-47 standard solution and 10 µL/tube of bio-pAb-BDE-47 dilution were added and then incubated for 1 h at 37°C. After washing three times with PBST, 20 µL/tube of streptavidin dilution was added and incubated for 30 min at 37°C. After washing, 20 µL/tube of bio-DNA solution was added and incubated for 30 min at 37°C. After a final washing step (five times with PBST and five times with ddH2O), the SYBR Green I PCR mixture was added.
The BA-IPCR assay was performed in 20 μL of reaction mixture containing 0.4 μL of 10 μM forward primer, 0.4 μL of 10 μM reverse primer, 9.2 μL of ddH2O and 10 μL of SYBR Green I Real-Time PCR Master Mix. PCR amplification was performed as follows: initial denaturation for 4 min at 94°C, 30 cycles of 20 s at 94°C, 20 s at 55°C, and 20 s at 72°C, with the final extension of 3 min at 72°C. The fluorescent intensity was detected at the final step of each cycle.
Optimisation of reaction conditions
According to the relevant immunoassay (Sun & Zhuang, Citation2015), 0.5% gelatin was used as blocking reagent. Several other reaction conditions were optimised. The optimal concentrations of bio-pAb-BDE-47 and BDE-47 coating antigen were used in the immunoassay. The number of immunisation complexes reduced rapidly if the concentrations of reagents were not appropriate. The chessboard assay was used to optimise the working concentrations of reagents to improve the sensitivity of the immunoassay. The OVA-BDE-47 concentrations were 420, 210, 105, 53 and 26 µg mL−1; the bio-pAb-BDE-47 was diluted to 1:20, 1:40, 1:60, 1:80 and 1:100, respectively. The optimal combinations of reagents would result in a minimum Ct value between 10 and 15 (Lind & Kubista, Citation2005). The result is shown in . The optimal concentration of OVA-BDE-47 was 105 µg mL−1, and the bio-pAb-BDE-47 was diluted to 1:80.
Table 1. Optimal concentrations of bio-pAb-BDE-47 and OVA-BDE-47.
As streptavidin was the linker between bio-DNA and bio-pAb-BDE-47, different concentrations of streptavidin (2.5, 5, 7.5, 10, 12 and 15 µg mL−1, in PBS) were investigated. As shown in (a), the Ct value decreased with the increasing streptavidin concentration. Considering the coefficient of variation (CV), 10 µg mL−1 streptavidin, which resulted in the minimum CV, was used in the immunoassay. The bio-DNA concentration has an influence on the number of initial template DNA in the PCR analysis; different bio-DNA concentrations (0.075, 0.15, 0.45, 0.75, 1.2 and 1.5 µg mL−1, in PBS) were also investigated. As shown in (b), Ct decreased with the increasing bio-DNA concentration. Considering the CV and economic cost, the optimal concentration of bio-DNA was 0.75 µg mL−1.
Figure 8. The optimisation of several operating conditions in the BA-IPCR assay. (a) Streptavidin concentration; (b) bio-DNA concentration and (c) the percentage of DMSO in PBS buffer.
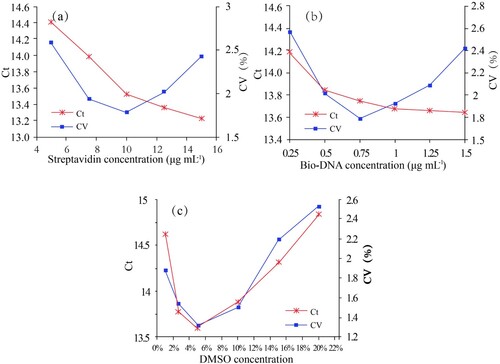
As BDE-47 is a type of hydrophobic organic compound, DMSO was added in PBS buffer to prepare BDE-47 standard solutions. Considering that different DMSO concentrations make differences to matrix effect and resulting interferences, the effect of DMSO concentration in BDE-47 solution (v/v = 1%, 2.5%, 5%, 10%, 15% and 20%) was investigated. As shown in (c), the matrix effects indicated that much higher or lower amounts of organic solvent negatively affected the assay performance. 5% DMSO, which obtain the Ct value of 13.68, was used in the assay.
Establishment of the standard curve
Under optimal conditions, different concentrations of BDE-47 standard solution (1 pg L−1, 10 pg L−1, 100 pg L−1, 1 ng L−1, 10 ng L−1, 100 ng L−1 and 1 µg L−1) were prepared with PBS buffer containing 5% DMSO and reacted using the BA-IPCR method (). The standard curve was constructed by plotting the average threshold cycle values (Ct, the cycle number at which the amplification curve crosses the fluorescent threshold) of six replicates against the logarithm of BDE-47 concentrations.
Cross-reactivity
The cross-reactivity (CR) was used to evaluate the specificity of the immunoassay. Several other types of PBDEs, which commonly exist in food or environment samples, were selected as BDE-47 analogues, such as BDE-15, BDE-28, BDE-66, BDE-99, BDE-100, BDE-153, BDE-183 and BDE-209. BDE-209, which is obviously different from BDE-47 in the number of bromine atom, was also tested because of its high application and widespread distribution in the environment and in food samples. CR was calculated according to the formula: CR = (IC50 of BDE-47)/(IC50 of analogues) × 100%.
Recovery test
The recoveries of the samples spiked with the target analyte were tested to evaluate the accuracy and precision of the immunoassay. Three marine fish samples (P4, S4, Y3), which have been tested using the BA-IPCR method, were used as known concentration samples and were spiked with different concentrations of the BDE-47 standard before extraction. Each sample was pre-treated as described above and detected by the BA-IPCR method.
Results and discussion
The sensitivity and stability of BA-IPCR
The competitive BA-IPCR assay was used to detect BDE-47 standard solutions under optimum conditions. The standard curve is shown in ; the linear working range was from 10 pg L−1 to 100 ng L−1; the linear equation was Ct = 0.2419logCBDE-47 + 13.325 (R2 = 0.9843). The limit of detection (LOD) was 2.96 pg L−1 (LOD = 3.3σ/S, σ is the standard deviation of the blank sample, S is the slope of the standard curve). The stability of the immunoassay was evaluated by performing the BA-IPCR procedure with several different concentrations of BDE-47 standard solutions (10 pg L−1, 100 pg L−1, 1 ng L−1, 10 ng L−1 and 100 ng L−1). The intra-assay repeatability was estimated over six replicates, and the inter-assay repeatability was evaluated over six individual times. Both the intra-assay and inter-assay CV were below 15%, indicating good repeatability of the immunoassay.
Specificity of BA-IPCR
As described earlier, the specificity of immunoassay was evaluated in the ability of the antibodies to combine with only the target molecule, i.e. CR indirectly. The chemical structure of the analogues and the CR results are shown in . In all cases, there was a low CR (below 6.12%) between BDE-47 and other structurally similar compounds, indicating that the pAb-BDE-47 was suitable for the specific determination of BDE-47 at low levels.
Table 2. The cross-reactivity of pAb-BDE-47with structural analogues.
Recovery rate
The recovery of the spiked samples was tested, and the results are shown in . The recoveries ranged from 92.97% to 107.19% and the coefficient of variations were 6.93–11.06%. It can be concluded that the immunoassay presented good accuracy.
Table 3. Determination of BDE-47 in spiked samples.
Determination of BDE-47 in marine fish samples and recovery test
The BA-IPCR method was applied to detect BDE-47 in several marine fish samples collected from the East China Sea. The average BDE-47 concentrations found in codfish, yellow croaker, pomfret and Spanish mackerel were 3.20, 14.52, 2.10 and 6.64 ng g−1 (these data were calculated according to ), respectively. BDE-47 concentrations of yellow croakers were obviously higher than those of other fishes; this may be because the fat content of this type of fish is high, which can contribute to the absorption and accumulation of BDE-47.
Table 4. Determination of BDE-47 by the BA-IPCR and GC–MS.
The marine fish samples were also tested using GC–MS to evaluate the accuracy of the immunoassay. Comparing the results of the BA-IPCR assay and the GC–MS analysis (), a correlation coefficient of 0.9893 was observed (y = 0.8739x + 0.1209). This indicated that the BA-IPCR assay was accurate and reliable. However, the detection results of the BA-IPCR assay were slightly higher than those of GC–MS method; this may be caused by the cross-reactivity between pAb-BDE-47 and BDE-47 analogues in the samples, which failed to be analysed by GC–MS method and contributed to the BA-IPCR-derived concentrations. As high-throughput detection can be achieved with the 96-well PCR plate, the BA-IPCR method has a batch testing mode for detecting BDE-47. Therefore, the analysis of BDE-47 in 16 samples only took about 6 h (excluding the incubation time [10 h] during the coating process), while the GC–MS method required about 48 h (each analysis needed about 30 min, and 6 replicates were tested for each practical sample). Therefore, this immunoassay is more appropriate to test large quantities of food samples.
Comparison with the correlated methods
Compared with the sensitive biotin–streptavidin-based enzyme-linked immunosorbent assay (BA-ELISA, Sun & Zhuang, Citation2015), which has also been studied by our research team, the enzyme chromogenic reaction signal used in the BA-ELISA was replaced by fluorescent signal labelled on template DNA in rt-IPCR analysis. Multiple-fold magnification of fluorescent signal can be achieved with the amplification of template DNA. Based on the OVA-BDE-47 and pAb-BDE-47, we also tested BDE-47 standard solutions using the BA-ELISA. The linear range of BA-ELISA method was 0.026 µg L−1–21.78 µg L−1; the LOD was 0.0067 µg L−1. This suggests that the LOD of the proposed method is much lower than that of the BA-ELISA method. Therefore, the BA-IPCR method is more appropriate for detecting trace amount of BDE-47 in food samples.
In addition, compared with the electrochemical immunosensor assay (EIA) for detecting BDE-47 (Fan, Keum, Li, Shelver, & Guo, Citation2012, this immunoassay has a linear working range of 0.3 µg L−1–6.9 µg L−1; the LOD of 0.18 µg L−1), this new method is also considerably more sensitive. In addition, as high-throughput detection can be achieved at one time with the 96-well PCR plate, this immunoassay is more convenient and less time-consuming for monitoring a large number of samples. In comparison with the fluorescence enhancement-based label-free homogeneous immunoassay (FIA, Bettazzi et al., Citation2016, this immunoassay has a linear working range of 3.1 µg L−1–390 µg L−1; the LOD of 0.73 µg L−1), the fluorescent signal is much stronger with the amplification of DNA, and the LOD is also much lower. The signal of analytes, which is indirectly detected by real-time immuno-PCR, can be considerably enhanced; this imparts the immunoassay with high sensitivity. Finally, as described earlier, compared with the GC–MS method, the BA-IPCR has a batch testing mode; it is less time-consuming and is more suitable for detecting large amounts of BDE-47 samples.
Conclusions
In this study, two diverse BDE-47 antigens and pAb-BDE-47 were prepared. A highly sensitive real-time immuno-PCR assay using the biotin–streptavidin system was developed and used for the monitoring of BDE-47 in marine fish collected from the East China Sea. The proposed method was applied to detect BDE-47 with an LOD of 2.96 pg L−1; the linear range was from 10 pg L−1 to 100 ng L−1. The recovery of spiked samples ranged from 92.97% to 107.19%, and the CV was from 6.93 to 11.06%. The BDE-47 data for several marine fish samples were consistent with those obtained by using GC–MS, which indicated that this immunoassay was accurate. Therefore, the proposed BA-IPCR method is sensitive, rapid, and reliable, and it is a useful option for detecting trace amounts of BDE-47 in the food samples.
Disclosure statement
No potential conflict of interest was reported by the authors.
Additional information
Funding
References
- Ahn, K. C., Gee, S. J., Tsai, H. J., Bennett, D., Nishioka, M. G., Blum, A., … Hammock, B. D. (2009). Immunoassasy for monitoring environmental and human exposure to the polybrominated diphenyl ether BDE-47. Environmental Science & Technology, 43(20), 7784–7790. doi: https://doi.org/10.1021/es9009037
- Bettazzi, F., Martellini, T., Shelver, W. L., Cincinelli, A., Lanciotti, E., & Palchetti, I. (2016). Development of an electrochemical immunoassay for the detection of polybrominated diphenyl ethers (PBDEs). Electroanalysis, 28(8), 1817–1823. doi: https://doi.org/10.1002/elan.201600127
- Bradman, A., Castorina, R., Sjödin, A., Fenster, L., Jones, R. S., Harley, K. G., … Eskenazi, B. (2012). Factors associated with serum polybrominated diphenyl ether (PBDE) levels among school-age children in the CHAMACOS cohort. Environmental Science & Technology, 46(13), 7373–7381. doi: https://doi.org/10.1021/es3003487
- Castorina, R., Bradman, A., Sjödin, A., Fenster, L., Jones, R. S., Harley, K., … Eskenazi, B. (2011). Determinants of serum polybrominated diphenyl ether (PBDE) levels among pregnant women in the CHAMACOS cohort. Environmental Science & Technology, 45(15), 6553–6560. doi: https://doi.org/10.1021/es104295m
- Chen, L. Y., Wei, H. P., Guo, Y. C., Cui, Z. Q., Zhang, Z. P., & Zhang, X. E. (2009). Gold nanoparticle enhanced immuno-PCR for ultrasensitive detection of Hantaan virus nucleo-capsid protein. Journal of Immunological Methods, 346, 64–70. doi: https://doi.org/10.1016/j.jim.2009.05.007
- Cloutier, P. L., Fortin, F., Groleau, P. E., Brousseau, P., Fournier, M., & Desrosiers, M. (2017). QuEChERS extraction for multi-residue analysis of PCBs, PAHs, PBDEs and PCDD/Fs in biological samples. Talanta, 165, 332–338. doi: https://doi.org/10.1016/j.talanta.2016.12.080
- Covaci, A., Harrad, S., Abdallah, M. A. E., Ali, N., Law, R. J., Herzke, D., & de Wit, C. A. (2011). Novel brominated flame retardants: A review of their analysis, environmental fate and behaviour. Environment International, 37, 532–556. doi: https://doi.org/10.1016/j.envint.2010.11.007
- Fan, Z. Y., Keum, Y. S., Li, Q. X., Shelver, W. L., & Guo, L. H. (2012). Development of indirect competitive fluorescence immunoassay for 2,2′,4,4′-tetrabromodiphenyl ether using DNA/dye conjugate as antibody multiple labels. Journal of Environmental Sciences, 24(7), 1334–1340. doi: https://doi.org/10.1016/S1001-0742(11)60929-7
- Gong, Y., Wen, S., Zheng, C., Peng, X., Li, Y., Hu, D., & Peng, L. (2015). Potential risk assessment of polybrominated diphenyl ethers (PBDEs) by consuming animal-derived foods collected from interior areas of China. Environmental Science & Pollution Research International, 22(11), 8349–8358. doi: https://doi.org/10.1007/s11356-014-3940-2
- Goodrow, M. H., & Hammock, B. D. (1998). Hapten design for compound-selective antibodies: ELISAs for environmentally deleterious small molecules. Analytica Chimica Acta, 376(1), 83–91. doi: https://doi.org/10.1016/S0003-2670(98)00433-4
- Hites, R. A. (2004). Polybrominated diphenyl ethers in the environment and in people: A meta-analysis of concentrations. Environmental Science & Technology, 38, 945–956. doi: https://doi.org/10.1021/es035082g
- Ionas, A. C., Ballesteros Gómez, A., Uchida, N., Suzuki, G., Kajiwara, N., Takata, K., … Covaci, A. (2015). Comprehensive characterisation of flame retardants in textile furnishings by ambient high resolution mass spectrometry, gas chromatography-mass spectrometry and environmental forensic microscopy. Environmental Research, 142, 712–719. doi: https://doi.org/10.1016/j.envres.2015.09.012
- Król, S., Zabiegała, B., & Namieśnik, J. (2012). PBDEs in environmental samples: Sampling and analysis. Talanta, 93(93), 1–17. doi: https://doi.org/10.1016/j.talanta.2012.01.048
- Lacorte, S., Ikonomou, M. G., & Fischer, M. (2010). A comprehensive gas chromatography coupled to high resolution mass spectrometry based method for the determination of polybrominated diphenyl ethers and their hydroxylated and methoxylated metabolites in environmental samples. Journal of Chromatography A, 1217(3), 337–347. doi: https://doi.org/10.1016/j.chroma.2009.11.024
- La Guardia, M. J., Hale, R. C., & Harvey, E. (2006). Detailed polybrominated diphenyl ether (PBDE) congener composition of the widely used penta-, octa-, and deca-PBDE technical flame-retardant mixtures. Environmental Science & Technology, 40(20), 6247–6254. doi: https://doi.org/10.1021/es060630m
- Lee, H. J., & Kim, G. B. (2015). An overview of polybrominated diphenyl ethers (PBDEs) in the marine environment. Ocean Science Journal, 50(2), 119–142. doi: https://doi.org/10.1007/s12601-015-0010-8
- Lind, K., & Kubista, M. (2005). Development and evaluation of three real-time immuno-PCR assemblages for quantification of PSA. Journal of Immunological Methods, 304(1), 107–116. doi: https://doi.org/10.1016/j.jim.2005.06.015
- Liu, Y. P., Li, J. G., Zhao, Y. F., Wen, S., Huang, F. F., & Wu, Y. N. (2011). Polybrominated diphenyl ethers (PBDEs) and indicator polychlorinated biphenyls (PCBs) in marine fish from four areas of China. Chemosphere, 83(2), 168–174. doi: https://doi.org/10.1016/j.chemosphere.2010.12.045
- Martellini, T., Diletti, G., Scortichini, G., Lolini, M., Lanciotti, E., Katsoyiannis, A., & Cincinelli, A. (2016). Occurrence of polybrominated diphenyl ethers (PBDEs) in foodstuffs in Italy and implications for human exposure. Food and Chemical Toxicology, 89, 32–38. doi: https://doi.org/10.1016/j.fct.2015.12.026
- Niemeyer, C. M., Adler, M., & Wacker, R. (2005). Immuno-PCR: High sensitivity detection of proteins by nucleic acid amplification. Trends in Biotechnology, 23(4), 208–216. doi: https://doi.org/10.1016/j.tibtech.2005.02.006
- Sano, T., Smith, C. L., & Cantor, C. R. (1992). Immuno-PCR: Very sensitive antigen detec-tion by means of specific antibody-DNA conjugates. Science, 258, 120–122. doi: https://doi.org/10.1126/science.1439758
- Shang, X. H., Dong, G. X., Zhang, H. X., Zhang, L., Yu, X., Li, J., … Wu, Y. N. (2016). Polybrominated diphenyl ethers (PBDEs) and indicator polychlorinated biphenyls (PCBs) in various marine fish from Zhoushan fishery, China. Food Control, 67, 240–246. doi: https://doi.org/10.1016/j.foodcont.2016.03.008
- Shelver, W. L., Keum, Y. S., Kim, H. J., Rutherford, D., Hakk, H. H., Bergman, Å., Li, Q. X. (2005). Hapten syntheses and antibody generation for the development of a polybrominated flame retardant ELISAII. Journal of Agricultural and Food Chemistry, 53(10), 3840–3847. doi: https://doi.org/10.1021/jf047863m
- Shelver, W. L., Parrotta, C. D., Slawecki, R., Li, Q. X., Ikonomou, M. G., Barcelo, D., … Rubio, F. M. (2008). Development of a magnetic particle immunoassay for polybrominated diphenyl ethers and application to environmental and food matrices. Chemosphere, 73(1 Suppl), S18–S23. doi: https://doi.org/10.1016/j.chemosphere.2007.01.088
- Stapleton, H. M., Eagle, S., Sjödin, A., & Webster, T. F. (2012). Serum PBDEs in a North Carolina toddler cohort: Associations with handwipes, house dust, and socioeconomic variables. Environmental Health Perspectives, 120(7), 1049–1054. doi: https://doi.org/10.1289/ehp.1104802
- Sun, R. Y., & Zhuang, H. S. (2015). Biotin-streptavidin enzyme-linked immunosorbent assay for the determination of dibutyl phthalate in beverages and drinking water using a specific polyclonal antibody. Food Analytical Methods, 8, 1990–1999. doi: https://doi.org/10.1007/s12161-014-0085-3
- Tanaka, K., Takada, H., Yamashita, R., Mizukawa, K., Fukuwaka, M., & Watanuki, Y. (2015). Facilitated leaching of additive-derived PBDEs from plastic by seabirds’ stomach oil and accumulation in tissues. Environmental Science & Technology, 49(19), 11799–11807. doi: https://doi.org/10.1021/acs.est.5b01376
- Thorenz, U. R., Musa Bandowe, B. A., Sobocka, J., & Wilcke, W. (2010). Method optimization to measure polybrominated diphenyl ether (PBDE) concentrations in soils of Bratislava, Slovakia. Environmental Pollution, 158(6), 2208–2217. doi: https://doi.org/10.1016/j.envpol.2010.02.021
- Troisi, G. M., & Borjesson, L. (2005). Development of an immunoassay for the determination of polyaromatic hydrocarbons in plasma samples from oiled seabirds. Environmental Science & Technology, 39, 3748–3755. doi: https://doi.org/10.1021/es048935t
- Wang, D., & Li, Q. X. (2010). Application of mass spectrometry in the analysis of polybrominated diphenyl ethers. Mass Spectrometry Reviews, 29(5), 737–775. doi: https://doi.org/10.1002/mas.20263
- Xu, P. X., Xiao, H. S., & Ju, G. (1999). A simplification of the immuno-PCR assay. Progress in Biochemistry and Biophysics, 26(4), 396–398.
- Zhuang, Y., Gao, X., Gao, A., & Fan, M. (2012). A biotin-streptavidin amplified enzyme-linked immunosorbent assay with improved sensitivity for rapid detection of ractopamine in muscular tissue. Food Analytical Methods, 5(5), 1214–1220. doi: https://doi.org/10.1007/s12161-012-9363-0