ABSTRACT
To develop new immunostimulatory ingredients, green tea polysaccharides were obtained by pectinase-assisted extraction. The present study investigated the immune stimulating and protective ability of polysaccharides from pectinase digests of green tea (PPGT) against infectious disease and the mechanisms involved. Macrophages stimulated with PPGT exhibited dose-dependent increases in nitric oxide, tumour necrosis factor-α (TNF-α) production and phagocytosis. PPGT increased phosphorylation of mitogen-activated protein (MAP) kinase, nuclear factor-kappaB (NFκB) and signal transducers and activators of transcription (STATs) through toll-like receptor 4 (TLR4) activation. The survival of mice infected with a lethal dose (1 × 107 CFU) of S. typhimurium was significantly extended by PPGT administration. Furthermore, PPGT induced production of cytokines (interleukin-12, interferon-γ, and TNF-α) in mice infected with a sub-lethal dose (1 × 105 CFU) of S. typhimurium. Furthermore, PPGT was significantly promoted natural killer cell and cytotoxic T lymphocyte activity. The above results lead to conclude that PPGT have a potent immune stimulating effect on immune cells via TLR4 pathway.
Introduction
Green tea prepared from Camellia sinensis is a popular beverage throughout Asia and is recognized as a herbal remedy (Dekant, Fujii, Shibata, Morita, & Shimotoyodome, Citation2017; Saeed et al., Citation2017). Catechin polyphenols, such as epicatechin, epigallocatechin (EGC), epicatechin-3-gallate, and epigallocatechin-3-gallate (EGCG), are the major components responsible for the beneficial effects of green tea (Du et al., Citation2016; Monobe, Ema, Tokuda, & Maeda-Yamamoto, Citation2010). Moreover, green tea polysaccharides have been reported to possess immunological, anti-radiation, anticarcinogenic, anti-HIV, antioxidant, and anti-fatigue and hypoglycemic properties (Du et al., Citation2016; Lu et al., Citation2017; Lu, Zhao, Sun, Yang, & Yang, Citation2013).
Recently, more evidence suggest that numerous polysaccharides isolated from various sources have immunostimulatory effects are appearing. Although most of such data derive from in vitro experiments or oral administration of polysaccharides to experimental animals (Pan et al., Citation2017; Shu et al., Citation2017; Zhang et al., Citation2018). The extraction of polysaccharides by hot water results in relatively poor yields. In order to increase the yield and physiological activity of polysaccharides, researchers have adjusted variables such as temperature and pH and employed enzymes in the extraction process (Baik et al., Citation2015; Zhai et al., Citation2018), but these methods remain suboptimal.
It is well known that the plant cell wall comprises a three-dimensional dynamic network composed of cellulose, pectins, crosslinking glycans, and proteins (Nagai et al., Citation2017). Pectinase may be used to cleave pectin and pectic compounds. Pectinase was the cell wall may be broken down, decreasing mass transfer resistance (Barman, Sit, Badwaik, & Deka, Citation2014). Moreover, the reaction is mild and the products stable, and pectinase can improve extraction efficiency and shorten extraction time (Barman et al., Citation2014; Palaniyandi, Suh, & Yang, Citation2017; Zhai et al., Citation2018). Here, polysaccharides from pectinase digests of green tea (PPGT) was performed, using the optimal temperature and time identified from previous studies of pectinase treatment. The immunostimulatory activity and the underlying signalling mechanism were investigated. In addition, the effect of polysaccharides from PPGT on host defence was examined by its administration to mice and subsequent challenge with the pathogen S. typhimurium.
Materials and methods
Chemical and reagents
Roswell Park Memorial Institute medium 1640 (RPMI) and foetal bovine serum (FBS) were obtained from GIBCO (Grand Island, NY, USA). Nitric oxide (NO) detection kit was obtained from INTRON Biotechnology (Sungnam, Korea). Trizol was obtained from Invitrogen (Carlsbad, CA, USA). Interleukin (IL)-6, IL-12, interferon-gamma (IFN-γ) and tumour necrosis factor-alpha (TNF-α) were obtained from R&D Systems (Minneapolis, MN, USA). Penicillin, streptomycin, neutral red, 3-(4,5-dimethylthiazol-2-yl)-2,5-diphenyltetrazolium bromide (MTT), lipopolysaccharide (LPS), and all other chemicals were obtained from Sigma-Aldrich (St. Louis, MO, USA).
Cell culture and animal care
RAW264.7 cells were routinely cultured in Dulbecco’s Modified Eagle Medium (DMEM; Sigma-Aldrich) supplemented with 10% heat-inactivated FBS, 100 U/mL penicillin, and 100 μg/mL of streptomycin. Cells were grown at 37°C in a humidified 5% CO2 incubator. S. typhimurium was grown on LB broth at 37°C under aerobic conditions. All experiments were performed in accordance with the guidelines for animal experimentation of Wonkwang University and were approved by the university’s institutional animal care and use committee (Approval No. WKU17-21).
Preparation of standardized PPGT
PPGT was supplied by BTC Corporation and used for experiments. For extraction, 1 kg of ground tea leaves was added to 10 L water and heated twice at 90°C for 2–4 h. 0.5% pectinase (26,000 1 PGU/mL, v/w) based on the dry weight of green tea leaves was added to the filtrate and the mixture was incubated at 40°C for 30 min. The enzyme was then inactivated by heating the mixture at 90°C for 1 h. The product was concentrated to 10° Brix at 60°C in a vacuum evaporator and dried in a freeze-dryer after solvent precipitation (FD8505, Ilshin Corp., Seoul, Republic of Korea) to yield PPGT (7.12%, w/w). For standardization, EGC + EGCG content was estimated as a maker of PPGT. The EGC + EGCG content was 14.24 mg/g.
HPLC estimation of EGC + EGCG
PPGT was dissolved in HPLC-grade methanol to a concentration of 5 mg/mL and filtered through a 0.45-μm syringe filter. The reference standard comprised 0.5 mg EGC and EGCG in 1 mL HPLC-grade methanol. The HPLC system consisted of a model 515 pump and model 717 autosampler (Waters, Beverly, MA, USA). Reverse-phase separation was performed at room temperature using a YMC Triart C18 ExRS column (ID of 4.6 mm × 250 mm, particle size of 5 μm). The mobile phase included water containing 0.1% Phosphoric acid and ACN containing 0.1% Acetic acid. The flow rate was maintained at 1 mL/min and the peak was detected at 206 nm. The marker compound was identified by comparing the UV spectrum and retention time ().
Antioxidant activity
To ascertain the antioxidant activity of the green tea extracts, a DPPH radical scavenging assay was performed using the method described by Klouwen (Citation1962). The inhibition rate was calculated as follows: (1 – A/B) × 100, where A is the absorbance of the sample and B is the absorbance of the control. A superoxide radical scavenging assay was also performed using a SOD Assay Kit-WST (Dojindo, Tokyo, Japan) according to the manufacturer’s instructions.
Cell viability assay
RAW264.7 were then cultured for 24 h in 96-well culture plates at 2.5 × 104 cells/well in the presence or absence of 10, 30, or 100 μg/mL PPGT or 1 μg/mL LPS as a positive control for 24 h. MTT solution was added to each well and the plate was incubated for another 4 h before completion of the cell viability assay.
NO assay
RAW264.7 (1 × 105 cells/well) were incubated in a 96-well plate with 10, 30, or 100 μg/mL PPGT for 24 h. NO was measured by determining the concentration of nitrite, its stable oxidative metabolite, using a microplate assay according to a previously described method (Chang et al., Citation2015).
Measurement of cytokine levels
TNF-α, IL-6, IL-12, and IFN-γ production were measured by the sandwich ELISA method according to the manufacturer’s instructions. ELISA kits for IL-6 (DY406), IL-12 (DY419), and TNF-α (DY410) were provided by R&D Systems and that for IFN-γ (558258) was supplied by BD PharMingen (San Jose, CA, USA).
Peritoneal macrophage phagocytosis assay
Phagocytosis assays were performed as described by Han et al. (Citation2009). The fluorescence intensity (in relative fluorescence units) associated with intracellular bacteria was determined using a spectrofluorometer (Infinite F200, Tecan, Zurich, Switzerland).
Toll-like receptor 4 (TLR4) gene knockdown and quantitative reverse-transcription (RT)-polymerase chain reaction (PCR)
RAW264.7 cells were transfected using Lipofectamine by adding to the culture medium TLR4 siRNA (siRNA ID 110005, Thermo Fisher Scientific, Waltham, MA, USA) or nonspecific siRNA (AM4611, Thermo Fisher Scientific) combined with Opti-MEM. For control cultures, only Opti-MEM was added to the medium. After 24 h, the cells were treated for a further 24 h with PPGT or LPS, before being collected for RNA isolation. For amplification of TLR4 (Mm00445273) and iNOS (Mm00440485), TaqMan Gene Expression Assay (Applied Biosystems, Foster City, CA, USA) primers and probes were used. Expression levels of target genes were normalized to those of the housekeeping gene GAPDH. Relative expression levels and PCR efficiency were evaluated. Data analysis was performed with SDS 2.1.1 software (Applied Biosystems).
MAPK, NFkB, and STAT-1 protein expression analysis
Treated cells were lysed by adding RIPA buffer directly to the plate. After isolation, the concentration of protein in the samples was determined using a Micro BCA Assay Kit. The membrane was blocked with 5% skim milk and sequentially incubated with phospho P38, phospho JNK, phospho ERK, phospho NF-κB, phospho STAT1 and GAPDH antibodies at 4°C overnight. Immunoreactive bands were visualized using horseradish peroxidase-conjugated secondary antibodies and ECL detection reagent. Images were captured using a FluorChem E system (ProteinSimple, Santa Clara, CA, USA).
MAPK and NFκB inhibitor assay
The cells were pre-incubated with the MAPK inhibitors SB202190 (10 μM), SP600125 (10 μM), and PD98059 (25 μM) and ammonium pyrrolidine-dithio-carbamate (PDTC; 50 μM) for 1 h before PPGT treatment, before being collected for RNA isolation. For Amplification of iNOS (Mm00440485), TNF-ɑ (Mm00443258) and GAPDH (Mm99999915) was performed using TaqMan Gene Expression Assay primers and probes. Data analysis was performed with SDS 2.1.1 software. Expression of each target gene was normalized to that of the housekeeping gene GAPDH. Relative expression levels and PCR efficiency were evaluated.
Animals and experimental design
Male ICR mice (6 weeks old and weighing 25–27 g) were purchased from Orient Bio (Iksan, Republic of Korea) and randomly divided into two sets. The first set comprised seven groups, each of 10 mice, as follows: normal, S. typhimurium infection, S. typhimurium infection 50 mg/kg β-glucan(positive control) and S. typhimurium infection with 10, 30, 100, and 300 mg/kg/day PPGT. The second set also comprised seven groups, with 10 mice in each group. The mice were divided into six groups: normal, S. typhimurium infection, S. typhimurium infection 50 mg/kg β-glucan(positive control) and S. typhimurium infection with 10, 30, 100, and 300 mg/kg/day PPGT. Mice in the normal and S. typhimurium infection groups were administered an equivalent volume of distilled water instead of PPGT. PPGT or β-glucan was dissolved in sterile double-distilled water and administered for 12 consecutive days. After 5 days of PPGT treatment, mice of the first and second set were intraperitoneally injected with a lethal (1 × 107 CFU/mouse) and sublethal (1 × 105 CFU/mouse) dose, respectively, of a bacterial suspension to induce peritonitis. The challenged mice were given a standard diet and water for 7 days and their survival and health were monitored twice per day for the duration of the experiment. At the end of the experiment, the mice were anaesthetized with ether, and blood samples were taken from the central vein.
Natural killer cell and cytotoxic T lymphocyte activity assay
Splenocytes were collected from infected mice and used as effector cells at a concentration of 1 × 106 cells/well. YAC-1 cells (as a natural killer (NK) cell target) and CT26 cells (as a cytotoxic T lymphocyte (CTL) target) were seeded in RPMI 1640 complete medium in a 96-well, flat-bottom microtiter plate at 2 × 104 cells/well to give an effector:target ratio of 50:1. The plates were incubated for 24 h at 37°C in an atmosphere containing 5% CO2. MTT solution was added to each well and the plate was incubated for another 4 h before completion of the MTT assay. The percentage of target cells killed (% lysis) was calculated as ((ODT – (ODS – ODE))/ODT) × 100, where ODT is the OD of the target cell control, ODS is the OD of the test sample, and ODE is the OD of the effector cell control.
Statistical analysis
Data are expressed as means ± SD and were examined for statistically significant differences by analysis of variance and Student’s t-test. p-Values < .05 were considered statistically significant.
Results
PPGT antioxidant activity
Hydroxyl radical scavenging increased with PPGT concentration, being 24.5%, 64.7%, and 92.4% at concentrations of 10, 30, and 100 μg/mL, respectively, 50 μM vitamin C (positive control), was recorded as 72.1%. The scavenging activity of PPGT at 100 μg/mL was significantly higher than that of vitamin C ((A)). PPGT was also tested for superoxide radical scavenging activity, which was found to range from 8.3% to 59.4%. A superoxide radical scavenging value of 60.9% was recorded for the positive control, trolox 500 μg/mL. PPGT at 100 μg/mL had an effect similar in degree to that of the positive control ((B)).
Effect of PPGT on macrophage activation
There was no cytotoxicity of PPGT at the experimental concentration ((A)). NO production was 2.99 ± 0.38 μM in the untreated group and 19.8 ± 7.05 μM in the 1 μg/mL LPS group (the positive control). TNF-α secretion was 0.40 ± 0.02 ng/mL in the untreated group and 2.34 ± 0.15 ng/mL in the LPS group. The PPGT associated NO and TNF-α were significantly increased to 2.5–5.6 fold and 2.6–4.6 fold of untreated group, respectively ((B,C)).
Figure 3. Effect of PPGT on macrophage activation. RAW264.7 cells were treated with 10, 30, or 100 μg/mL PPGT or 1 μg/mL LPS for 24 h, after which, cell viability (A), NO (B) and TNF-α (C) secretion was measured. The values shown are means ± SD. **p < .01, ***p < .001 compared with the untreated (UN) group.
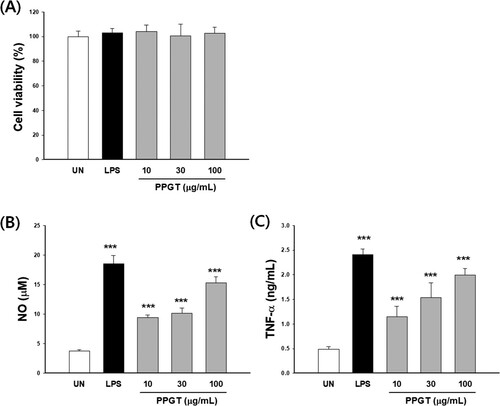
To determine the effect of PPGT on the phagocytic activity of macrophages, the uptake of FITC-labeled E. coli cells by PPGT-treated and untreated macrophages were compared. Phagocytosis of E. coli was significantly increased 2.8–3.9 fold treated with PPGT compared to the untreated group, demonstrating that PPGT enhances macrophage phagocytosis ().
Figure 4. Effect of PPGT on phagocytosis. RAW264.7 cells were treated with 10, 30, or 100 μg/mL PPGT or 1 μg/mL LPS (as a positive control) for 24 h, before being incubated with FITC-labeled E. coli for 2 h. Phagocyosed bacteria was measured with a fluorescence microplate reader. The values shown are means ± SD. **p < .01, ***p < .001 compared with the untreated (UN) group.
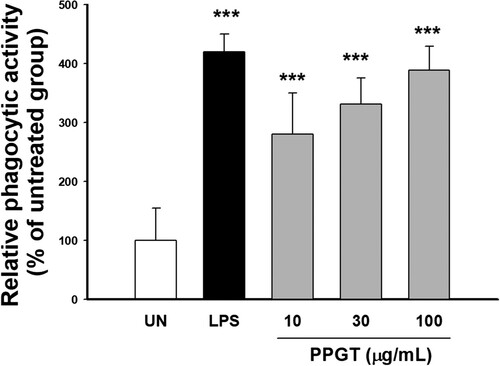
Effect of PPGT on mechanisms of macrophage stimulation
Knockdown of TLR4 was performed by transfection of TLR4 siRNA into macrophages, resulting in a 56.8% reduction of TLR4 gene expression compared to the nonspecific siRNA treatment (data not shown). Expression of iNOS mRNA increased 1.31, 5.51, and 6.38 fold following treatment with 10, 30, and 100 mg/mL PPGT the compared to nonspecific siRNA. In TLR4-knockdown, iNOS expression was 1.00, 1.20, and 2.13 fold that in the untreated group after administration respectively. iNOS expression was significantly inhibited in the TLR4-knockdown group than the nonspecific siRNA group at PPGT concentrations of 30 and 100 mg/mL ((A)).
Figure 5. Effect of TLR4 knockdown on PPGT-induced iNOS expression. RAW264.7 cells were transfected with 100 nM TLR4 siRNA using Lipofectamine. After 24 h, the cells were treated with 10, 30, or 100 µg/mL PPGT or 10 µg/mL LPS for a further 24 h, and subsequently collected for RNA isolation. iNOS gene expression was determined by quantitative RT-PCR. The values shown are means ± SD. *p < .05, **p < .01, ***p < .001 compared with the nonspecific siRNA group.
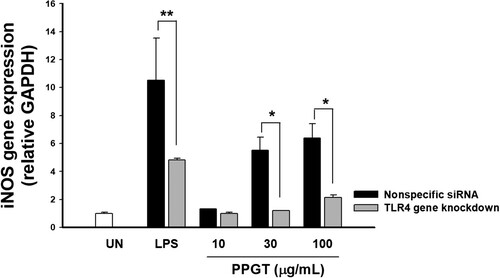
PPGT induced phosphorylation of p38, ERK, JNK, IKK, STAT-1 in macrophages in a dose-dependent fashion ((A)). To confirm the association between PPGT and MAPK and NFκB activation, the effects of several inhibitors (SB203580 for p38, SP600125 for JNK, and PD98059 for ERK) on PPGT-treated macrophages were investigated. PPGT-induced iNOS and TNF-α expression were significantly decreased after treatment with inhibitors of MAPK and NFκB activation ((B,C)).
Figure 6. Effect of PPGT on the downstream of TLR4 signalling pathway. (A) Cytosolic protein extracts were prepared and analysed by western blotting using phospho-ERK, phospho-JNK, phospho-p38, phospho-IKK, phospho-STAT1 antibodies. (B,C) Cells were pretreated for 2 h with SB203580 (a p38 inhibitor), SP600125 (a JNK inhibitor), PD98059 (an ERK inhibitor), or PDTC before exposure to PPGT. iNOS and TNF-α gene expression was determined by quantitative RT-PCR. The values shown are means ± SD. *p < .05, **p < .01, ***p < .001 compared with the untreated (UN) group. #p < .05, ##p < .01, ###p < .001 compared with the PPGT-only group.
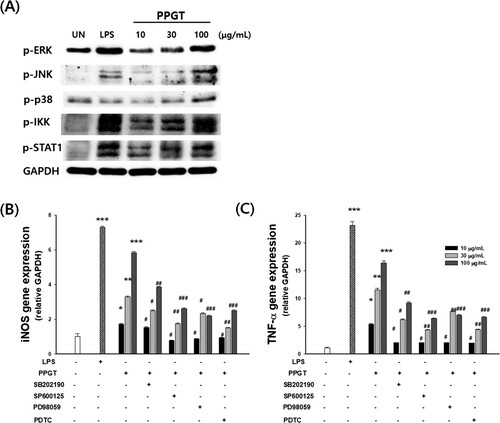
Effect of PPGT on the survival of lethal dose of S. typhimurium-infected mice
shows that only 10% of mice administered lethal dose of S. typhimurium were alive by day 7. In contrast, the survival rate in the β-glucan positive control group was 50% at the same time point. Of the mice treated with 10, 30, 100, or 300 mg/kg PPGT along with S. typhimurium, 20%, 30%, 40%, and 40% remained alive, respectively, on day 7. These observations demonstrate the dose-dependent protective influence of PPGT against the lethal effects of S. typhimurium on mice.
Figure 7. Effect of PPGT on the survival in mice challenged with lethal dose of S. typhimurium. Mice were orally administered 10, 30, 100, or 300 mg/kg PPGT for 12 days and subsequently challenged with a lethal dose of a bacterial suspension (1 × 107 CFU/mice) by intraperitoneal injection to induce peritonitis. The challenged mice were kept for 7 days on a standard diet with water and their survival was monitored twice per day throughout the experimental period.
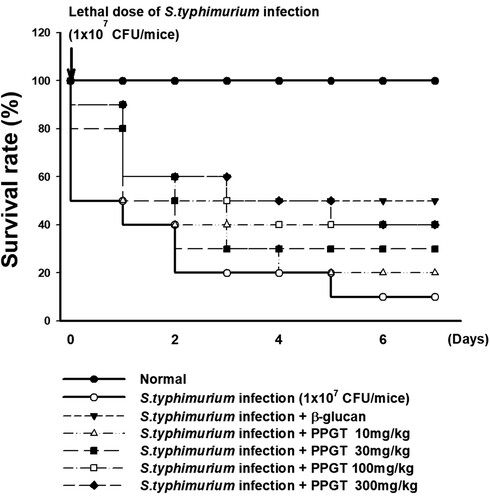
Effect of PPGT on the body weight of sub-lethal dose of S. typhimurium-infected mice
Average body weight across all groups before the start of the experiment was 32.4 ± 0.60 g. Prior to infection with S. typhimurium, no significant difference in body weight was noted between the PPGT and positive control groups. Weight loss was observed in all groups following S. typhimurium (1 × 105 CFU/mouse) infection. The body weight of mice administered only S. typhimurium was 12.2% lower on day 2 than the pre-infection weight (day 0), and had increased by day 7 to be 5.3% lower than that on day 0. In the PPGT groups, recovery of weight was observed from day 4 post infection. At the end of the experiment, there was no significant difference between the groups ().
Figure 8. Effect of PPGT on the body weight in mice challenged with sub-lethal dose of S. typhimurium. Mice were orally administered 10, 30, 100, or 300 mg/kg PPGT for 12 days and subsequently challenged with a sub-lethal dose of a bacterial suspension (1 × 105 CFU/mice) by intraperitoneal injection to induce peritonitis. Body weight was recorded daily.
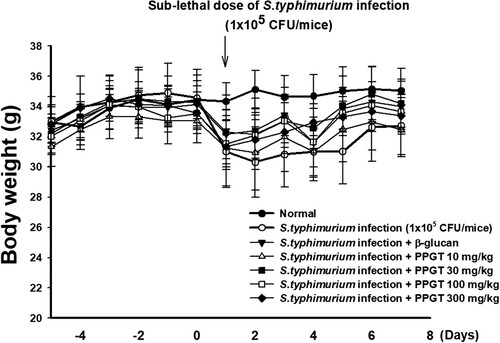
Effects of PPGT on cytokine production in sub-lethal dose of S. typhimurium-infected mice
IL-6 secretion did not show any significant difference PPGT ((A)). IL-12 secretion was increased by 21% in the infected the compared S. typhimurium-infected group. IL-12 secretion was 19 – 45% higher than that in the normal group ((B)). Concerning secretion of TNF-α, mice treated with only S. typhimurium demonstrated an increase of 84.6% compared to those in the normal group. TNF-α secretions were 106%, 115%, 133%, and 142% higher in 10, 30, 100, and 300 mg/kg PPGT groups, respectively, than the normal group ((C)). The increase resulting from the highest dose was statistically significant. Secretion of IFN-γ was 46.9% higher in the S. typhimurium-only group than the normal group. IFN-γ secretion was significantly increased in the 30, 100, and 300 mg/kg PPGT+ S. typhimurium groups, by 95%, 141%, and 154%, respectively ((D)).
Figure 9. Effect of PPGT on the production of cytokines in mice challenged with sub-lethal dose of S. typhimurium. Mice were orally administered 10, 30, 100, or 300 mg/kg PPGT for 12 days and subsequently challenged with a sub-lethal dose of a bacterial suspension (1 × 105 CFU/mice) by intraperitoneal injection to induce peritonitis. At the end of the experiment, the mice were anesthetized using ether. Serum was then collected from blood samples to measure levels of (A) IL-6, (B) IL-12, (C) IFN-γ, and (D) TNF-α. The values shown are means ± SD. *p < .05 compared with the normal group.
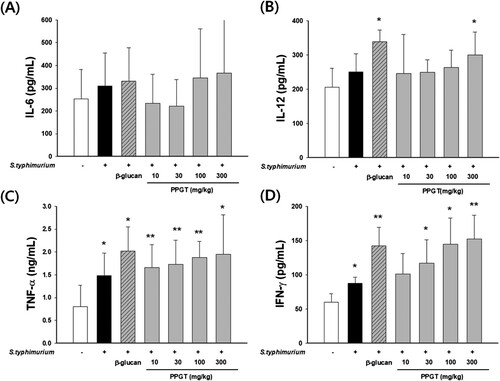
Effects of PPGT on NK cell and CTL activity in sub-lethal dose of S. typhimurium-infected mice
A difference of 24.6% was noted between NK cell activity in the S. typhimurium-only group (56.4 ± 3.67%) and that in the normal group (31.8 ± 2.7%). NK cell activity also significantly differed, by 19.3%, between the β-glucan positive control group (67.3 ± 4.48%) and the S. typhimurium-only group. PPGT did not show any significant effect on NK cell activity ((A)).
Figure 10. Effects of PPGT on NK cell and CTL activity in sub-lethal dose of S. typhimurium-infected mice. Splenocytes were collected from sub-lethal dose of S. typhimurium-infected mice as effector cells. YAC-1 and CT26 cells were used as NK cell and CTL targets, respectively, at an effector:target ratio of 50:1. (A) NK cell and (B) CTL activity was measured. The values shown are means ± SD. **<.01, ***p < .001 compared with the S. typhimurium-only group.
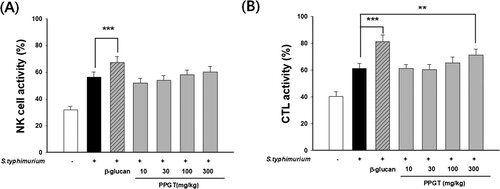
CTL activity was higher in mice administered only S. typhimurium (61.0 ± 4.0%) than in those of the normal group (40.3 ± 3.4%), with a difference of 20.7% being recorded. CTL activity significantly differed, by 20%, between the β-glucan-treated positive control group (81.0 ± 5.3%) and the S. typhimurium-only group. A dose-dependent increase in CTL activity was observed in relation to PPGT treatment, with increases of 10.0% being noted in the 300 mg/kg PPGT+ S. typhimurium groups, respectively, and the difference associated with the amount of these doses was statistically significant ((B)).
Discussion
One approach to the effective release of bioactive substances from protein and carbohydrate sources is enzymatic hydrolysis. Indeed, this method is widely applied to enhance the functional, nutritional, and pharmacological properties of proteins and carbohydrates (Kim et al., Citation2011; Thitiratsakul & Anprung, Citation2014). In addition, several studies have reported that enzymatic hydrolysates of plants exert biological effects, including antioxidant and anticoagulant activity and inhibition of enzymes and cell proliferation (Ridley, O’Neill, & Mohnen, Citation2001; Zhai et al., Citation2018). Pectinase and cellulase treatment significantly increased yield and soluble solid content (Baik et al., Citation2015; Ridley et al., Citation2001; Zhai et al., Citation2018). In the present study, attempts were made to enhance the polysaccharide content and increased the radical scavenging and immunostimulatory activity of green tea extract involving a pectin-hydrolysing enzyme.
Polysaccharides have been reported as act as effective free radical scavengers and antioxidants, playing a critical role in protecting organisms against oxidative damage (Liao et al., Citation2017; Liu & Huang, Citation2017; Lu et al., Citation2013). Our results indicate that PPGT has a strong hydroxyl and superoxide radical scavenging capacity.
Activation of macrophages is a key event for initiating and propagating defensive reactions against pathogens. Phagocytosis is the first step in the macrophage response to invading microorganisms, and activated macrophages further function in antigen processing and presentation, and antibody-dependent cell-mediated cytotoxicity (Deng, Fu, Shang, Chen, & Xu, Citation2018; Fang et al., Citation2015; Wang et al., Citation2018; Wang, Wakeham, Harkness, & Xing, Citation1999). We observed that PPGT enhances phagocytic activity in macrophage. Macrophage also induces the production of numerous cytokine or mediators, such as TNF-α, interferons, interleukins, NO, and other reactive oxygen species. NO can diffuse freely through cells and is synthesized from l-arginine by NOS, which contributes to killing microorganisms and tumour cells through activated macrophages and mediates a variety of biological functions as an intracellular messenger molecule (Fonseca et al., Citation2003). The roles of TNF-α include activation and chemotaxis of leukocytes and induction of the expression of adhesion molecules (Parameswaran & Patial, Citation2010). PPGT was significantly increased the release of TNF-α and NO produced by macrophages. Therefore, PPGT-mediated phagocytosis and production of NO and cytokines contribute to its immunostimulatory.
TLRs are innate immune sensors that recognize conserved microbial structures and signal expression of MHC proteins, co-stimulatory molecules, and inflammatory mediators by macrophages, neutrophils, dendritic cells, and other cell types. The binding of polysaccharides to TLR4 is a critical event in immune activation (Tewari et al., Citation2017). iNOS and TNF-α signalling pathway is activated by TLR4 and transduced via mitogen-activated protein kinases MAPKs (ERK, p38 and JNK), NFκB as well as a Janus tyrosine kinase (JAK) and signal transducers and activators of transcription (STATs) (Chang, Kim, Lee, Park, & Kim, Citation2015; Zhou et al., Citation2015). PPGT was found to increase the phosphorylation of ERK, JNK, p38, IKK, and STAT-1 in a dose-dependent manner. PPGT-induced iNOS expression was attenuated by knockdown of TLR4, therefore, immune activity resulting from PPGT administration may occur through activation of TLR4 signalling. Moreover, inhibition of MAPK and NFκB signalling by pretreatment with MAPK inhibitors and PDTC inhibited PPGT-induced increases in iNOS and TNF-α gene expression (Chang et al., Citation2015; Sun et al., Citation2013). These results suggest that PPGT-mediated macrophage activation largely depends on MAPK and NFκB signalling. Taken together, our findings demonstrate that PPGT may act as a macrophage activator and immune stimulator through TLR4 signalling.
We found that PPGT enhanced immune activity and resistance to experimental challenge with S. typhimurium. The protective effect of PPGT against this pathogen is likely to be mediated by potentiation of host defence, such as macrophage activation, rather than by indirect inhibition of bacterial growth.
IL-12 is produced primarily by monocytes, macrophages, and other antigen-presenting cells and is essential in responses to infectious diseases and cancer (Tong et al., Citation2015; Xing, Zganiacz, & Santosuosso, Citation2000). IL-12 acts synergistically with TNF and other proinflammatory cytokines to stimulate IFN-γ production, as well as NK cells and CD8+ cytotoxic T cells. Levels of the proinflammatory cytokine TNF-α in plasma directly correlate with superoxide generation by phagocytes and iNOS activity and, thus, NO levels (Wherry, Schreiber, & Unanue, Citation1991; Zwirner & Ziblat, Citation2017). The cytokines IL-12, IFN-γ, and TNF-α, which have vital roles in both innate and adaptive immunity and are responsible for Th1 polarization (Belardelli, Citation1995; Wang et al., Citation1999), were upregulated in the PPGT-treated groups, further indicating important immunoregulatory activity via activation of macrophages. However, Th2 cytokine production (IL-6) was unaffected. The immune system includes a population of lymphocyte-like cells that induce cytotoxicity in pathogens. NK lymphocytes contribute substantially to the inhibition of pathogens, and do not need to be primed by prior antigen recognition. By contrast, CTLs are activated upon recognition of antigens on the surface of immune cells (Chang et al., Citation2015; Gonzales et al., Citation2012; Mills, Lenz, & Ley, Citation2015). PPGT significantly increased NK cell and CTL activity in S. typhimurium-infected mice, suggesting that it can enhance the function of specific and nonspecific cytotoxic cells. Macrophages, NK cells, and NK T cells can produce IFN-γ during the innate immune response and have immunostimulatory properties, augmenting defence against pathogens (Dillon, Lee, Bramante, Barker, & Wilson, Citation2014; Rivera, Siracusa, Yap, & Gause, Citation2016). The increased resistance to pathogens conferred by PPGT may be explained by the observed increases in IL-12, TNF-α, and IFN-γ secretion by macrophages in mice treated with this extract.
Conclusion
PPGT exhibited antioxidant properties, stimulated cytokine production via TLR4, and enhanced macrophage defence against bacterial infection. Macrophage activation was induced through TLR4-mediated MAPK, NFκB and STAT1 signalling pathways, which initiated phagocytosis and the release of cytokines, TNF-α and effector molecules NO. The survival rate of mice infected with S. typhimurium was significantly increased when PPGT was administered. These results suggest that enhancement of innate immune and host defence activity by PPGT occur through activation of TLR4 signalling.
Acknowledgements
This research was supported by the Ministry of Trade, Industry & Energy (MOTIE) and the Korea Institute for Advancement of Technology (KIAT) through the Encouragement Program for The Industries of Economic Cooperation Region (R004933). SYK and TYK conceived and designed the experiments. BYC performed the experiments. BYC, SYK, and TYK analysed the data.
Disclosure statement
No potential conflict of interest was reported by the authors.
References
- Baik, J. H., Shin, K. S., Park, Y., Yu, K. W., Suh, H. J., & Choi, H. S. (2015). Biotransformation of catechin and extraction of active polysaccharide from green tea leaves via simultaneous treatment with tannase and pectinase. Journal of the Science of Food and Agriculture, 95(11), 2337–2344. doi: https://doi.org/10.1002/jsfa.6955
- Barman, S., Sit, N., Badwaik, L. S., & Deka, S. C. (2014). Pectinase production by Aspergillus niger using banana (Musa balbisiana) peel as substrate and its effect on clarification of banana juice. Journal of Food Science and Technology, 52(6), 3579–3589. doi: https://doi.org/10.1007/s13197-014-1413-8
- Belardelli, F. (1995). Role of interferons and other cytokines in the regulation of the immune response. Apmis, 103(3), 161–179. doi: https://doi.org/10.1111/j.1699-0463.1995.tb01092.x
- Chang, B. Y., Kim, S. B., Lee, M. K., Park, H., & Kim, S. Y. (2015). Improved chemotherapeutic activity by Morus alba fruits through immune response of toll-like receptor 4. International Journal of Molecular Sciences, 16(10), 24139–24158. doi: https://doi.org/10.3390/ijms161024139
- Dekant, W., Fujii, K., Shibata, E., Morita, O., & Shimotoyodome, A. (2017). Safety assessment of green tea based beverages and dried green tea extracts as nutritional supplements. Toxicology Letters, 277, 104–108. doi: https://doi.org/10.1016/j.toxlet.2017.06.008
- Deng, C., Fu, H., Shang, J., Chen, J., & Xu, X. (2018). Dectin-1 mediates the immunoenhancement effect of the polysaccharide from Dictyophora indusiata. International Journal of Biological Macromolecules, 109, 369–374. doi: https://doi.org/10.1016/j.ijbiomac.2017.12.113
- Dillon, S. M., Lee, E. J., Bramante, J. M., Barker, E., & Wilson, C. C. (2014). The natural killer cell interferon-gamma response to bacteria is diminished in untreated HIV-1 infection and defects persist despite viral suppression. JAIDS Journal of Acquired Immune Deficiency Syndromes, 65(3), 259–267. doi: https://doi.org/10.1097/01.qai.0000435603.50598.2b
- Du, L. L., Fu, Q. Y., Xiang, L. P., Zheng, X. Q., Lu, J. L., Ye, J. H., … Liang, Y. R. (2016). Tea polysaccharides and their bioactivities. Molecules, 21(11). doi: https://doi.org/10.3390/molecules21111449
- Fang, Q., Wang, J. F., Zha, X. Q., Cui, S. H., Cao, L., & Luo, J. P. (2015). Immunomodulatory activity on macrophage of a purified polysaccharide extracted from Laminaria japonica. Carbohydrate Polymers, 134, 66–73. doi: https://doi.org/10.1016/j.carbpol.2015.07.070
- Fonseca, S. G., Romao, P. R., Figueiredo, F., Morais, R. H., Lima, H. C., Ferreira, S. H., & Cunha, F. Q. (2003). TNF-alpha mediates the induction of nitric oxide synthase in macrophages but not in neutrophils in experimental cutaneous leishmaniasis. European Journal of Immunology, 33(8), 2297–2306. doi: https://doi.org/10.1002/eji.200320335
- Gonzales, C. M., Williams, C. B., Calderon, V. E., Huante, M. B., Moen, S. T., Popov, V. L., … Endsley, J. J. (2012). Antibacterial role for natural killer cells in host defense to Bacillus anthracis. Infection and Immunity, 80(1), 234–242. doi: https://doi.org/10.1128/iai.05439-11
- Han, E. H., Choi, J. H., Hwang, Y. P., Park, H. J., Choi, C. Y., Chung, Y. C., … Jeong, H. G. (2009). Immunostimulatory activity of aqueous extract isolated from Prunella vulgaris. Food and Chemical Toxicology, 47(1), 62–69. doi: https://doi.org/10.1016/j.fct.2008.10.010
- Kim, H. Y., Jeong, H. S., Min, B. C., Ahn, S. H., Oh, S. C., Yoon, Y. H., … Yang, I. (2011). Antifungal efficacy of environmentally friendly wood preservatives formulated with enzymatic-hydrolyzed okara, copper, or boron salts. Environmental Toxicology and Chemistry, 30(6), 1297–1305. doi: https://doi.org/10.1002/etc.515
- Klouwen, H. M. (1962). Determination of the sulfhydryl content of thymus and liver using DPPH. Archives of Biochemistry and Biophysics, 99, 116–120. doi: https://doi.org/10.1016/0003-9861(62)90252-7
- Liao, B. Y., Zhu, D. Y., Thakur, K., Li, L., Zhang, J. G., & Wei, Z. J. (2017). Thermal and antioxidant properties of polysaccharides sequentially extracted from mulberry leaves (Morus alba L.). molecules, 22(12). doi: https://doi.org/10.3390/molecules22122271
- Liu, Y., & Huang, G. (2017). Antioxidant effects of polysaccharides from traditional Chinese medicines. Mini-Reviews in Medicinal Chemistry, doi: https://doi.org/10.2174/1389557518666171207160318
- Lu, L., Chai, L., Wang, W., Yuan, X., Li, S., & Cao, C. (2017). A selenium-enriched Ziyang green tea polysaccharide induces Bax-dependent mitochondrial apoptosis and inhibits TGF-beta1-stimulated collagen expression in human keloid fibroblasts via NG2 inactivation. Biological Trace Element Research, 176(2), 270–277. doi: https://doi.org/10.1007/s12011-016-0827-8
- Lu, X., Zhao, Y., Sun, Y., Yang, S., & Yang, X. (2013). Characterisation of polysaccharides from green tea of Huangshan Maofeng with antioxidant and hepatoprotective effects. Food Chemistry, 141(4), 3415–3423. doi: https://doi.org/10.1016/j.foodchem.2013.06.058
- Mills, C. D., Lenz, L. L., & Ley, K. (2015). Macrophages at the fork in the road to health or disease. Frontiers in Immunology, 6(59), doi: https://doi.org/10.3389/fimmu.2015.00059
- Monobe, M., Ema, K., Tokuda, Y., & Maeda-Yamamoto, M. (2010). Effect on the epigallocatechin gallate/epigallocatechin ratio in a green tea (Camellia sinensis L.) extract of different extraction temperatures and its effect on IgA production in mice. Bioscience, Biotechnology, and Biochemistry, 74(12), 2501–2503. doi: https://doi.org/10.1271/bbb.100498
- Nagai, K., Ueno, Y., Tanaka, S., Hayashi, R., Shinagawa, K., & Chayama, K. (2017). Polysaccharides derived from Ganoderma lucidum fungus mycelia ameliorate indomethacin-induced small intestinal injury via induction of GM-CSF from macrophages. Cellular Immunology, 320, 20–28. doi: https://doi.org/10.1016/j.cellimm.2017.08.001
- Palaniyandi, S. A., Suh, J. W., & Yang, S. H. (2017). Preparation of ginseng extract with enhanced levels of ginsenosides Rg1 and Rb1 using high hydrostatic pressure and polysaccharide hydrolases. Pharmacognosy Magazine, 13(Suppl 1), S142–s147. doi: https://doi.org/10.4103/0973-1296.203992
- Pan, G., Xie, Z., Huang, S., Tai, Y., Cai, Q., Jiang, W., … Yuan, Y. (2017). Immune-enhancing effects of polysaccharides extracted from Lilium lancifolium Thunb. International Immunopharmacology, 52, 119–126. doi: https://doi.org/10.1016/j.intimp.2017.08.030
- Parameswaran, N., & Patial, S. (2010). Tumor necrosis factor-alpha signaling in macrophages. Critical Reviews in Eukaryotic Gene Expression, 20(2), 87–103. doi: https://doi.org/10.1615/CritRevEukarGeneExpr.v20.i2.10
- Ridley, B. L., O’Neill, M. A., & Mohnen, D. (2001). Pectins: Structure, biosynthesis, and oligogalacturonide-related signaling. Phytochemistry, 57(6), 929–967. doi: https://doi.org/10.1016/S0031-9422(01)00113-3
- Rivera, A., Siracusa, M. C., Yap, G. S., & Gause, W. C. (2016). Innate cell communication kick-starts pathogen-specific immunity. Nature Immunology, 17(4), 356–363. doi: https://doi.org/10.1038/ni.3375
- Saeed, M., Naveed, M., Arif, M., Kakar, M. U., Manzoor, R., Abd El-Hack, M. E., … Sun, C. (2017). Green tea (Camellia sinensis) and l-theanine: Medicinal values and beneficial applications in humans – a comprehensive review. Biomedicine & Pharmacotherapy, 95, 1260–1275. doi: https://doi.org/10.1016/j.biopha.2017.09.024
- Shu, Y., Liu, X. B., Ma, X. H., Gao, J., He, W., Cao, X. Y., & Chen, J. (2017). Immune response mechanism of mouse monocytes/macrophages treated with kappa-carrageenan polysaccharide. Environmental Toxicology and Pharmacology, 53, 191–198. doi: https://doi.org/10.1016/j.etap.2017.06.010
- Sun, P., Zhou, K., Wang, S., Li, P., Chen, S., Lin, G., … Wang, T. (2013). Involvement of MAPK/NF-kappaB signaling in the activation of the cholinergic anti-inflammatory pathway in experimental colitis by chronic vagus nerve stimulation. PLoS One, 8(8), e69424. doi: https://doi.org/10.1371/journal.pone.0069424
- Tewari, P., Mandal, P., Roy, R., Asthana, S., Dwivedi, P. D., Das, M., & Tripathi, A. (2017). A novel function of TLR4 in mediating the immunomodulatory effect of benzanthrone, an environmental pollutant. Toxicology Letters, 276, 69–84. doi: https://doi.org/10.1016/j.toxlet.2017.05.008
- Thitiratsakul, B., & Anprung, P. (2014). Prebiotic activity score and bioactive compounds in longan (Dimocarpus longan Lour.): Influence of pectinase in enzyme-assisted extraction. Journal of Food Science and Technology, 51(9), 1947–1955. doi: https://doi.org/10.1007/s13197-014-1263-4
- Tong, H., Mao, D., Zhai, M., Zhang, Z., Sun, G., & Jiang, G. (2015). Macrophage activation induced by the polysaccharides isolated from the roots of Sanguisorba officinalis. Pharmaceutical Biology, 53(10), 1511–1515. doi: https://doi.org/10.3109/13880209.2014.991834
- Wang, Y., Tian, Y., Shao, J., Shu, X., Jia, J., Ren, X., & Guan, Y. (2018). Macrophage immunomodulatory activity of the polysaccharide isolated from Collybia radicata mushroom. International Journal of Biological Macromolecules, 108, 300–306. doi: https://doi.org/10.1016/j.ijbiomac.2017.12.025
- Wang, J., Wakeham, J., Harkness, R., & Xing, Z. (1999). Macrophages are a significant source of type 1 cytokines during mycobacterial infection. Journal of Clinical Investigation, 103(7), 1023–1029. doi: https://doi.org/10.1172/jci6224
- Wherry, J. C., Schreiber, R. D., & Unanue, E. R. (1991). Regulation of gamma interferon production by natural killer cells in scid mice: Roles of tumor necrosis factor and bacterial stimuli. Infection and Immunity, 59(5), 1709–1715.
- Xing, Z., Zganiacz, A., & Santosuosso, M. (2000). Role of IL-12 in macrophage activation during intracellular infection: IL-12 and mycobacteria synergistically release TNF-alpha and nitric oxide from macrophages via IFN-gamma induction. Journal of Leukocyte Biology, 68(6), 897–902.
- Zhai, X., Zhu, C., Li, Y., Zhang, Y., Duan, Z., & Yang, X. (2018). Optimization for pectinase-assisted extraction of polysaccharides from pomegranate peel with chemical composition and antioxidant activity. International Journal of Biological Macromolecules, 109, 244–253. doi: https://doi.org/10.1016/j.ijbiomac.2017.12.064
- Zhang, J. H., Xin, H. L., Xu, Y. M., Shen, Y., He, Y. Q., Hsien, Y., … Du, J. (2018). Morinda officinalis How. – a comprehensive review of traditional uses, phytochemistry and pharmacology. Journal of Ethnopharmacology, 213, 230–255. doi: https://doi.org/10.1016/j.jep.2017.10.028
- Zhou, Y., Zhang, T., Wang, X., Wei, X., Chen, Y., Guo, L., … Wang, C. (2015). Curcumin modulates macrophage polarization through the inhibition of the toll-like receptor 4 expression and its signaling pathways. Cellular Physiology and Biochemistry, 36(2), 631–641. doi: https://doi.org/10.1159/000430126
- Zwirner, N. W., & Ziblat, A. (2017). Regulation of NK cell activation and effector functions by the IL-12 family of cytokines: The case of IL-27. Frontiers in Immunology, 8(25), doi: https://doi.org/10.3389/fimmu.2017.00025