ABSTRACT
Peanut is ranked among the eight major food allergens. Ara h 2 is its major allergen, recognized by more than 90% of serum IgE from peanut-allergic patients. Cross-linking catalysed by microbial transglutaminase (MTGase) is proved to be feasible to decrease the allergenicity of Ara h 2. The crosslinking reaction occurred both inter- and intra-molecular could gain products with different molecular weights. In this study, after MTGase catalysed crosslinking, the products were separated into low molecular weight part of Ara h 2 (LP-Ara h 2) and high molecular weight part of Ara h 2 (HP-Ara h 2), and the structure, digestibility, IgG and IgE binding capability of each part were analysed. Compared with LP-Ara h 2, HP-Ara h 2 was found to have looser structure, higher digestion rate and corresponding lower immunogenicity. By controlling the reaction condition to get different products, the protein desensitization processes would get a better result.
Introduction
Peanut is listed among the eight major food allergens ranked by the Food and Agriculture Organization. Peanut allergy is common and typically permanent, moreover, allergy to peanuts and other tree nuts account for the majority of fatal and near-fatal anaphylactic reactions (Celakovska, Ettlerova, Ettler, Vaneckova, & Bukac, Citation2011; Husain & Schwartz, Citation2012; Offermann et al., Citation2015; Peng, Song, Liu, Kuang, & Xu, Citation2015; Sampson, Citation2002). At present, 17 peanut allergens are officially registered at the Allergen Nomenclature Sub-Committee of the International Union of Immunological Societies (http://www.allergen.org). Ara h 2 is considered a major allergen among them, being recognized by serum immunoglobulin E (IgE) from over 90% of peanut-allergic patients (Barre, Borges, Culerrier, & Rouge, Citation2005; Chen et al., Citation2016; Li, Kulis, Pons, Zhong, & Burks, Citation2011; Stanley et al., Citation1997).
At present, strictly avoiding peanuts remains the most reliable treatment for allergic patients because oral immunotherapy is not ready for clinical use (Sampson, Citation2013; Tian, Rao, Tao, & Xue, Citation2018; Wasserman et al., Citation2018). Developing processes for structural improvement, nutrient controlled delivery and energy intake has recently become increasingly important (Schmitt, Nesbit, Hurlburt, Cheng, & Maleki, Citation2010; Wu et al., Citation2016). Peanut-based food ingredients can also be modified using both chemical and biological manipulations, including acidification, heating, proteolysis, high-pressure microfluidisation, irradiation, enzyme-catalysed cross-linking and so on (Cabanillas et al., Citation2012; Hu, Zhao, Sun, Zhao, & Ren, Citation2011; Jamdar et al., Citation2010; Liu, Zhao, Zhao, Ren, & Yang, Citation2012; Luo et al., Citation2013; Maleki, Chung, Champagne, & Raufman, Citation2000; Peng et al., Citation2014). Cross-linking reaction is a new process that can reduce the allergenicity of Ara h 2 without damaging its nutritional quality (Gerrard, Citation2002; Kamath et al., Citation2014). The modifications of proteins by enzymatic and chemical reagents have been widely examined and have been powerful tools to improve the functional properties of macromolecules (Bae, Shin, Kim, Kim, & Shon, Citation2013; Plundrich et al., Citation2014).
Microbial transglutaminase (MTGase) has been extensively used to improve the textural and functional properties of protein (Djoullah, Djemaoune, Husson, & Saurel, Citation2015; Dube, Schafer, Neidhart, & Carle, Citation2007; Sorrentino et al., Citation2012). This enzyme can catalyse acyl transfer reactions to accomplish the crosslinking reaction (Gharibzahedi & Chronakis, Citation2018; Jong, & Koppelman, & J, Citation2002; Motoki & Seguro, Citation1998). Moreover, Wu et al found that reduced Ara h 2 can be crosslinked more easily than untreated Ara h 2 because of its looser structure, after reduction and cross-linking, Ara h 2 was able to possess the properties of low allergenicity (Wu, Lian, et al., Citation2017). In a further study (Wu, Zhao, et al., Citation2017), it was found that intramolecular cross-linking and deamidation would occur simultaneously in addition to intermolecular crosslinking, obtaining different reaction products, while the contribution of each product on immunoreactivity remained unclear.
In this study, MTGase was applied to crosslink peanut allergen Ara h 2. After that, the reaction products were separated into two parts, low molecular weight part (LP-Ara h 2) and high molecular weight part (HP-Ara h 2). Then the structure, immunoreactivity and digestibility change of each part of Ara h 2 products were explored to find the contribution of each part.
Materials and methods
Materials
Peanuts were purchased from the local supermarket (Jiangxi, China). DEAE-Sepharose fast flow resin (16 × 250 mm) was purchased from Amersham Biosciences (Freiburg, Germany), and the 96-well microtiter plate was purchased from Nunc (Roskilde, Denmark). MTGase was purchased from YiMing (Zhejiang, China). Anilinonaphthalene Sulfonate (ANS), horseradish peroxidase-conjugated goat anti-rabbit IgG, Tween 20, pepsin and pancreatin were all obtained from Sigma (St Louis, MO, USA). All other chemicals were purchased from ShengGong (Shanghai, China). Table S1 provides the detailed information of the patients whose sera formed the serum pool.
Ara h 2 and enzymatic catalysed crosslinking
Ara h 2 was purified from crude peanut extraction using anion exchange chromatography according to the method of Hu (Hu, Chen, et al., Citation2011). Briefly, unshelled peanuts were powdered and then defatted by stirring in acetone. Peanut protein extract was obtained by mixing the defatted powder with 50 mmol L−1 Tris-HCl (pH 7.2) at a powder/solvent ratio of 1: 5 (w/v) for 10 h of stirring at 4°C. The ionic salts in the supernatant were removed by dialysis. The resulting crude extract was loaded onto DEAE-Sepharose Fast Flow Resin equilibrated with 50 mmol L−1 Tris-HCl (pH 7.2). Then the column was washed with the same buffer until the peak appeared, proteins were eluted successively with 500 mL of 0–0.2 mmol L−1 NaCl gradient in 50 mmol L−1 Tris-HCl and this fraction contained Ara h 2 was collected. After concentration, Ara h 2 (1 mg mL−1, pH 7.5) was reduced by dithiothreitol (DTT) (2.5 mg mL−1) at 50°C for 1 h, and then MTGase was added at a ratio of 30 units per gram of protein. The reaction was carried out at 50°C water bath for 5 h, ended by cold storage at −20°C, the reduced and cross-linked Ara h 2 (RC-Ara h 2) was stored at −20°C until use. And native Ara h 2 was treated under the same condition as RC-Ara h 2, except reduction treatment (MTG-Ara h 2), was used as control.
Separation of cross-linked products
After crosslinked reaction, RC-Ara h 2 was divided into two parts by a Millipore ultrafiltration tube (cutoff level: 50 kD). After centrifuged for 10 min (3000 rpm, 4°C) in the tube, the protein remained in the residue and left in solution were collected to be HP-Ara h 2 and LP-Ara h 2, respectively. Both parts were stored in PBS at −20°C till use.
Circular dichroism analysis
To define the secondary structure of four samples, namely, native Ara h 2, MTG-Ara h 2, LP-Ara h 2 and HP-Ara h 2 were characterized by circular dichroism (CD) spectroscopy using a J810 spectropolarimeter (JASCO, Groß-Umstadt, Germany) in solutions of 0.15 mg mL−1 (Hu, Chen, et al., Citation2011). The contents of different secondary structures were calculated using secondary structure software (http://dichroweb.cryst.bbk.ac.uk/html/home.shtml).
UV absorption spectral analysis
The UV absorption spectral analysis was performed using a UV-2450 spectrophotometer (Shimadzu, Kyoto, Japan). The UV absorption spectra were scanned from 200 to 420 nm (Hu, Chen, et al., Citation2011). The concentrations of these samples were all 0.15 mg mL−1.
Determination of protein surface hydrophobicity
Surface hydrophobicity was determined using the hydrophobic fluorescence probe ANS, measurements were performed according to the method of Takagi (Takagi, Akashi, & Yasumatsu, Citation1979). A 30 µL aliquot of 5 mmol L−1 ANS solution was added to 3 mL of each protein sample solution. The concentration of the four samples was 0.15 mg mL−1. The relative fluorescence was measured with an F-4500 fluorescence spectrophotometer. The excitation wavelength was 390 nm and the emission wavelength was from 400 to 650 nm at a scanning speed of 10 nm min−1.
Simulated gastric fluid
The four Ara h 2 samples were digested by pepsin according to Koppelman (Koppelman, Hefle, Taylor, & de Jong, Citation2010). The enzymatic activity of the reaction system was 1000 U mL−1. Aliquots (20 μL) were taken at 0 s and 30 s, as well as at 1, 2, 5, 10, 20, 40 and 80 min, during the digestion. The digestion reactions were stopped by adding 0.2 mol L−1 NaHCO3. The digested samples were frozen and stored at −20°C until analysed by Tricine-SDS-PAGE and ELISA.
Simulated intestinal fluid
After digested by pepsin for 80 min, the samples were subsequently digested by pancreatin according to US Pharmacopoeia (Maleki et al., Citation2003). Aliquots (20 μL) were taken at 0 s, as well as at 1, 2, 5, 10, 20, 40 and 80 min, during the digestion. The reaction was stopped by heating at 100°C for 5 min. The samples were then frozen and stored at −20°C before analysed by Tricine-SDS-PAGE and ELISA.
Tricine-SDS-PAGE and digestion rate calculation
The gel was prepared by overlaying a polymerized separating gel (16.5% and 10%) directly with a 4% sample (stacking) gel. Electrophoresis was performed at a constant voltage of 30 V in the 4% sample (stacking) gel for 1 h and 100 V in the separating gel for 3 h. For the digestion rate, the intensity of the bands corresponding to the undigested protein was regarded as 100%, and after a digestion period, the decreased intensity of these bands in the formerly fixed reaction time was inferred to be the digestion rate of that protein.
Antigenicity assessment
To assess the antigenicity of treated Ara h 2, specific-peanut IgG binding experiments were performed via inhibition ELISA (White et al., Citation2013; Wu et al., Citation2016). The rabbit antiserum was diluted to 1: 1500 in PBS, horseradish peroxidase-conjugated goat anti-rabbit IgG was diluted to 1: 5000 in PBS. The absorbance at 490 nm was read using a Bio-Rad 1860 microplate reader. The inhibition rate was calculated using the following equation: % inhibition = 1−B/B0 × 100, where B and B0 are the absorbance values of the well with and without the inhibitor, respectively. IC50 is the analyte concentration that causes a 50% inhibition of antibody binding.
Potential allergenicity assessment
The IgE binding experiments were also performed, via inhibition ELISA, to estimate the potential allergenicity of treated Ara h 2 (White et al., Citation2013; Wu et al., Citation2016). The concentration of protein used to coat the plate was 20 μg mL−1, human sera was diluted to 1: 50 in PBS, and biotin-labeled goat anti-human IgE was used to be the second antibody, horseradish peroxidase-conjugated streptavidin was added to react with biotin to expand the chromogenic reaction.
Statistical analysis
All data were processed in Origin (version 6.0) and reported as the average and standard deviation, unless otherwise stated. The bivariate Pearson correlation was analysed in SPSS (Chicago, IL, USA). Differences were considered significant and very significant when the p-values were less than 0.05 and 0.01, respectively.
Results
Separation of Ara h 2 products
After centrifuged (3000 rpm, 4°C, 10 min), high molecular weight part of Ara h 2 (HP-Ara h 2) remained in the residue, which contained dimer Ara h 2, tripolymer Ara h 2 and higher polymer of Ara h 2. While low molecular weight part of Ara h 2 (LP-Ara h 2) which contained monomer Ara h 2, including intermolecular cross-linked Ara h 2 and deamidated proteins, left in solution ().
Advanced structure characterization
The secondary structure of Ara h 2 samples was characterized by far-UV CD spectroscopy. The result showed that MTG-Ara h 2 had similar secondary structure with native Ara h 2, but crosslinking treatment after reduction could obviously change the secondary structure of Ara h 2 ((A)). The change of secondary structure was mainly shown on the content of α-helix, β-sheet and random coil of LP-Ara h 2 and HP-Ara h 2. For HP-Ara h 2, the α-helix content decreased to 12.3%, while the random coil increased to 36.7% (). The crosslinking with reducing treatment together significantly changed the secondary structure of Ara h 2 but crosslinking treatment alone, or intramolecular crosslinking and deamination, had little influence.
Figure 2. The advanced structure of proteins. A, CD spectrum; B, UV spectrum. NA means native Ara h 2; MTG-A means MTG-Ara h 2; LP-A means low molecular weight polymer of Ara h 2; HP-A means high molecular weight polymer of Ara h 2.
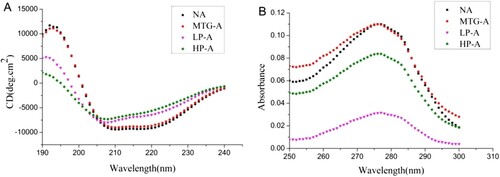
Table 1. Percentage of conformational unit in protein secondary structures.
In the UV spectrum, the absorption peak of MTG-Ara h 2 had little difference from that of native Ara h 2 at approximately 280 nm ((B)). But the absorption of HP-Ara h 2 and LP-Ara h 2 were much lower, LP-Ara h 2 had a lowest UV absorption, which indicated that structure of LP-Ara h 2 got a most compact tertiary structure.
Changes on surface hydrophobicity of Ara h 2
The ANS-induced fluorescence spectra of Ara h 2 samples were shown in . It can be seen that LP-Ara h 2 exhibited the highest fluorescence intensity, while that of MTG-Ara h 2 and HP-Ara h 2 increased slightly. The result indicated that the more hydrophobic groups exposed on the surface of LP-Ara h 2.
Assessment digestibility
As shown in , native Ara h 2 and MTG-Ara h 2 were hard to be digested by pepsin. The digestion rates of HP-Ara h 2 and LP-Ara h 2 were different: almost 50% HP-Ara h 2 could be digested into smaller peptides in 5 minutes, while about 70% LP-Ara h 2 remained on the gel after 20 minutes of pepsin digestion. That is, compared with LP-Ara h 2, HP-Ara h 2 were more easily to be digested by pepsin.
Figure 4. The digestion rate of gastric digested products. NA means native Ara h 2; MTG-A means MTG-Ara h 2; LP-A means low molecular weight polymer of Ara h 2; HP-A means high molecular weight polymer of Ara h 2.
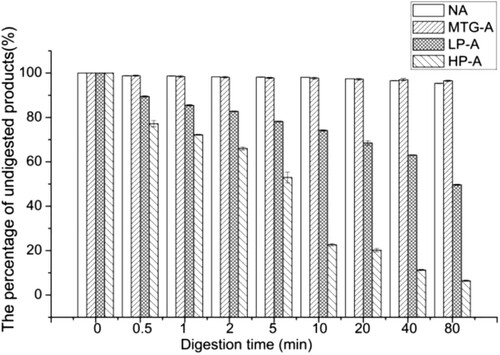
showed that native Ara h 2 and MTG-Ara h 2 could be digested by pancreatin gradually. Compared with them, the HP-Ara h 2 was evidently more sensitive to SIF digestion. It could be digested totally at the moment of contacting with pancreatin. There remained a 13 kDa fragment for LP-Ara h 2 at 1 minute, which would disappear 2 minutes later.
Assessment of antigenicity and potential allergenicity
The antigenicity of treated Ara h 2 and their digested products was assessed by indirect competitive inhibition ELISA. As shown in (A), the IC50 of native Ara h 2 and MTG-Ara h 2 had no significant difference. The IC50 of LP-Ara h 2 was 2 μg mL−1, while that of HP-Ara h 2 was 9 μg mL−1, indicating that the antigenicity of HP-Ara h 2 decreased obviously.
Figure 6. IgG (A) and IgE (B) binding capacity of treated Ara h 2. NA means native Ara h 2; MTG-A means MTG-Ara h 2; LP-A means low molecular weight polymer of Ara h 2; HP-A means high molecular weight polymer of Ara h 2. ** very significant differences (P < .01).
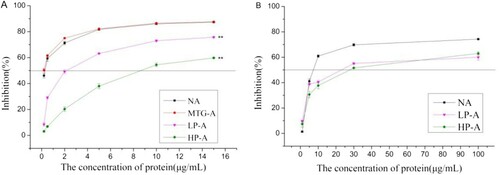
The potential allergenicity of treated Ara h 2 was assessed by inhibition ELISA. The IC50 of native Ara h 2 was 8 μg mL−1, while that of LP-Ara h 2 was 22 μg mL−1 and of HP-Ara h 2 was 27 μg mL−1 ((B)). This result implied that reducing and crosslinking treatment could significantly decrease the allergenicity of Ara h 2 and high polymer product exhibited a lower potential allergenicity.
Discussion
In this study, the products of reduction and crosslinking treatment were separated into two parts, LP-Ara h 2 and HP-Ara h 2, and their conformation and immunoreactivity were studied separately.
The native Ara h 2 was hardly to cross-linked with each other (Clare, Gharst, & Sanders, Citation2007), and only one intramolecular crosslinking peptide was identified in the product (Wu, Zhao, et al., Citation2017). The degree of cross-linking was too low to effect the conformation of Ara h 2. The second and tertiary structures of MTG-Ara h 2 were similar to that of native Ara h 2, while deamination slightly increased the surface hydrophobicity of MTG-Ara h 2. For the similar structure, MTG-Ara h 2 was also difficult to be digested as native Ara h 2 (Koppelman et al., Citation2010; Sen et al., Citation2002), so were their antigenicity (Wu, Zhao, et al., Citation2017).
The immunogenicity of Ara h 2 would decreased significantly when they were reduced before crosslinking treatment (Wu, Lian, et al., Citation2017) because of the structure change (Hu, Zhao, et al., Citation2011; Maleki et al., Citation2003). The possible cross-linked peptides contained phenylalanine and tryptophan (Wu, Lian, et al., Citation2017), and this reaction might mask these aromatic amino acids, reducing the UV absorption peaks of LP-Ara h 2 and HP-Ara h 2. The reduction and crosslinking treatment also unfolded Ara h 2, exposed the hydrophobic amino acid residues inside, which enhanced its surface hydrophobicity. The content of α-helical structure decreased, which was caused by not only the unfolding of protein, but also the involvement of K52 and K142 in the α-helix area (Hu, Gao, Luo, & Chen, Citation2009). As a result, the epitopes of IgG and IgE located at α-helix could be destroyed, which influenced the immunogenicity (Cabanillas et al., Citation2012; Hu, Chen, et al., Citation2011; Luo et al., Citation2013).
Between the cross-linked products, the surface hydrophobicity of LP-Ara h 2 was obviously higher than that of HP-Ara h 2, probably due to deamidation which could alter the solubility, weaken the aggregation of the protein, leading to the enhancement of the surface hydrophobicity (Wu, Zhao, et al., Citation2017). However, LP-Ara h 2 had a higher α-helix content than HP-Ara h 2 and lower UV absorbance than HP-Ara h 2, which may because the protein folded again after intramolecular cross-linking, forming a more compact structure (Djoullah, Krechiche, Husson, & Saurel, Citation2016). The intramolecular cross-linking could also mask the digestive enzyme sites and allergen epitopes, which caused the gastrointestinal digestion rate and immunogenicity of LP-Ara h 2 weaker than HP-Ara h 2. In addition, HP-Ara h 2 was the polymer composed of multiple monomers which would cover more allergen epitopes during its formation, that’s why the IgG and IgE binding capacity of HP-Ara h 2 were the lowest.
In the products, HP-Ara h 2 had a looser structure, and was more easily to decompose in gastrointestinal digestion. The previous study has found that high protein concentration benefited intermolecular cross-links (Wu, Zhao, et al., Citation2017). By increasing the concentration of protein, the reaction would produce more high polymer; the immunogenicity of the products will be greatly reduced. This would be a promising idea for desensitization of allergens by processing.
Conclusions
The crosslinking reaction occurred both inter- and intra-molecular could obtain products with different molecular weights. Compared with LP-Ara h 2, HP-Ara h 2 had unordered loose structure, higher digestion rate and corresponding lower antigenicity and potential allergenicity. By controlling the reaction condition, the protein desensitization processes would get better result.
Supplemental Material
Download MS Word (14.4 KB)Disclosure statement
No potential conflict of interest was reported by the authors.
ORCID
Zhihua Wu http://orcid.org/0000-0001-5988-3643
Additional information
Funding
References
- Bae, M. J., Shin, H. S., Kim, E. K., Kim, J., & Shon, D. H. (2013). Oral administration of chitin and chitosan prevents peanut-induced anaphylaxis in a murine food allergy model. International Journal of Biological Macromolecules, 61, 164–168. doi: https://doi.org/10.1016/j.ijbiomac.2013.06.017
- Barre, A., Borges, J. P., Culerrier, R., & Rouge, P. (2005). Homology modelling of the major peanut allergen Ara h 2 and surface mapping of IgE-binding epitopes. Immunology Letters, 100(2), 153–158. doi: https://doi.org/10.1016/j.imlet.2005.03.014
- Cabanillas, B., Maleki, S. J., Rodriguez, J., Burbano, C., Muzquiz, M., Jimenez, M. A., … Crespo, J. F. (2012). Heat and pressure treatments effects on peanut allergenicity. Food Chemistry, 132(1), 360–366. doi: https://doi.org/10.1016/j.foodchem.2011.10.093
- Celakovska, J., Ettlerova, K., Ettler, K., Vaneckova, J., & Bukac, J. (2011). The peanut allergy in patients suffering from atopic dermatitis. Food and Agricultural Immunology, 22(3), 195–204. doi: https://doi.org/10.1080/09540105.2010.549214
- Chen, X., Negi, S. S., Liao, S., Gao, V., Braun, W., & Dreskin, S. C. (2016). Conformational IgE epitopes of peanut allergens Ara h 2 and Ara h 6. Clinical and Experimental Allergy, 46(8), 1120–1128. doi: https://doi.org/10.1111/cea.12764
- Clare, D. A., Gharst, G., & Sanders, T. H. (2007). Transglutaminase polymerization of peanut proteins. Journal of Agricultural and Food Chemistry, 55(2), 432–438. doi: https://doi.org/10.1021/jf062309t
- Djoullah, A., Djemaoune, Y., Husson, F., & Saurel, R. (2015). Native-state pea albumin and globulin behavior upon transglutaminase treatment. Process Biochemistry, 50(8), 1284–1292. doi: https://doi.org/10.1016/j.procbio.2015.04.021
- Djoullah, A., Krechiche, G., Husson, F., & Saurel, R. (2016). Size measuring techniques as tool to monitor pea proteins intramolecular crosslinking by transglutaminase treatment. Food Chemistry, 190, 197–200. doi: https://doi.org/10.1016/j.foodchem.2015.05.091
- Dube, M., Schafer, C., Neidhart, S., & Carle, R. (2007). Texturisation and modification of vegetable proteins for food applications using microbial transglutaminase. European Food Research and Technology, 225(2), 287–299. doi: https://doi.org/10.1007/s00217-006-0401-2
- Gerrard, J. A. (2002). Protein-protein crosslinking in food: Methods, consequences, applications. Trends in Food Science & Technology, 13(12), 391–399. doi: https://doi.org/10.1016/S0924-2244(02)00257-1
- Gharibzahedi, S. M. T., & Chronakis, I. S. (2018). Crosslinking of milk proteins by microbial transglutaminase: Utilization in functional yogurt products. Food Chemistry, 245, 620–632. doi: https://doi.org/10.1016/j.foodchem.2017.10.138
- Hu, C. Q., Chen, H. B., Gao, J. Y., Luo, C. P., Ma, X. J., & Tong, P. (2011). High-pressure microfluidisation-induced changes in the antigenicity and conformation of allergen Ara h 2 purified from Chinese peanut. Journal of the Science of Food and Agriculture, 91(7), 1304–1309. doi: https://doi.org/10.1002/jsfa.4318
- Hu, C. Q., Gao, J. Y., Luo, C. P., & Chen, H. B. (2009). Prediction of second structure and B cell epitopes of peanut allergen Ara h 2.02. Food Science, 21, 13–15. doi: https://doi.org/10.3321/j.issn:1002-6630.2009.21.001
- Hu, X., Zhao, M. M., Sun, W. Z., Zhao, G. L., & Ren, J. Y. (2011). Effects of microfluidization treatment and transglutaminase cross-linking on physicochemical, functional, and conformational properties of peanut protein isolate. Journal of Agricultural and Food Chemistry, 59(16), 8886–8894. doi: https://doi.org/10.1021/jf201781z
- Husain, Z., & Schwartz, R. A. (2012). Peanut allergy: An increasingly common life-threatening disorder. Journal of the American Academy of Dermatology, 66(1), 136–143. doi: https://doi.org/10.1016/j.jaad.2011.02.031
- Jamdar, S. N., Rajalakshmi, V., Pednekar, M. D., Juan, F., Yardi, V., & Sharma, A. (2010). Influence of degree of hydrolysis on functional properties, antioxidant activity and ACE inhibitory activity of peanut protein hydrolysate. Food Chemistry, 121(1), 178–184. doi: https://doi.org/10.1016/j.foodchem.2009.12.027
- Jong, D. E., & Koppelman, G. A. H., & J, S. (2002). Transglutaminase catalyzed reactions: Impact on food applications. Journal of Food Science, 67(8), 2798–2806. doi: https://doi.org/10.1111/j.1365-2621.2002.tb08819.x
- Kamath, S. D., Rahman, A. M. A., Voskamp, A., Komoda, T., Rolland, J. M., O’Hehir, R. E., & Lopata, A. L. (2014). Effect of heat processing on antibody reactivity to allergen variants and fragments of black tiger prawn: A comprehensive allergenomic approach. Molecular Nutrition & Food Research, 58(5), 1144–1155. doi: https://doi.org/10.1002/mnfr.201300584
- Koppelman, S. J., Hefle, S. L., Taylor, S. L., & de Jong, G. A. H. (2010). Digestion of peanut allergens Ara h 1, Ara h 2, Ara h 3, and Ara h 6: A comparative in vitro study and partial characterization of digestion-resistant peptides. Molecular Nutrition & Food Research, 54(12), 1711–1721. doi: https://doi.org/10.1002/mnfr.201000011
- Li, Y. X., Kulis, M., Pons, L., Zhong, X. P., & Burks, A. W. (2011). Peanut allergen Ara h 2-specific T cells are activated via Ras-Erk MAP kinase pathway signalling and identified by CD154 expression. Food and Agricultural Immunology, 22(4), 335–344. doi: https://doi.org/10.1080/09540105.2011.579591
- Liu, Y., Zhao, G. L., Zhao, M. M., Ren, J. Y., & Yang, B. (2012). Improvement of functional properties of peanut protein isolate by conjugation with dextran through maillard reaction. Food Chemistry, 131(3), 901–906. doi: https://doi.org/10.1016/j.foodchem.2011.09.074
- Luo, C. P., Hu, C. Q., Gao, J. Y., Li, X., Wu, Z. H., Yang, A. S., & Chen, H. B. (2013). A potential practical approach to reduce Ara h 6 allergenicity by gamma irradiation. Food Chemistry, 136(3–4), 1141–1147. doi: https://doi.org/10.1016/j.foodchem.2012.09.091
- Maleki, S. J., Chung, S. Y., Champagne, E. T., & Raufman, J. P. (2000). The effects of roasting on the allergenic properties of peanut proteins. Journal of Allergy and Clinical Immunology, 106(4), 763–768. doi: https://doi.org/10.1067/mai.2000.109620
- Maleki, S. J., Viquez, O., Jacks, T., Dodo, H., Champagne, E. T., Chung, S. Y., & Landry, S. J. (2003). The major peanut allergen, Ara h 2, functions as a trypsin inhibitor, and roasting enhances this function. Journal of Allergy and Clinical Immunology, 112(1), 190–195. doi: https://doi.org/10.1067/mai.2003.1551
- Motoki, M., & Seguro, K. (1998). Transglutaminase and its use for food processing. Trends in Food Science & Technology, 9(5), 204–210. doi: https://doi.org/10.1016/S0924-2244(98)00038-7
- Offermann, L. R., Perdue, M. L., He, J. Z., Hurlburt, B. K., Maleki, S. J., & Chruszcz, M. (2015). Structural biology of peanut allergens. Journal of Contemporary Immunology, 2(1), 1–26. doi: https://doi.org/10.7726/jci.2015.1001
- Peng, J., Meng, X., Deng, X. F., Zhu, J. P., Kuang, H., & Xu, C. L. (2014). Development of a monoclonal antibody-based sandwich ELISA for the detection of ovalbumin in foods. Food and Agricultural Immunology, 25(1), 1–8. doi: https://doi.org/10.1080/09540105.2012.716398
- Peng, J., Song, S. S., Liu, L. Q., Kuang, H., & Xu, C. L. (2015). Development of sandwich ELISA and immunochromatographic strip for the detection of peanut allergen Ara h 2. Food Analytical Methods, 8(10), 2605–2611. doi: https://doi.org/10.1007/s12161-015-0163-1
- Plundrich, N. J., Kulis, M., White, B. L., Grace, M. H., Guo, R. S., Burks, A. W., … Lila, M. A. (2014). Novel strategy to create hypoallergenic peanut protein-polyphenol edible matrices for oral immunotherapy. Journal of Agricultural and Food Chemistry, 62(29), 7010–7021. doi: https://doi.org/10.1021/jf405773b
- Sampson, H. A. (2002). Clinical practice – peanut allergy. New England Journal of Medicine, 346(17), 1294–1299. doi: https://doi.org/10.1056/NEJMcp012667
- Sampson, H. A. (2013). Peanut oral immunotherapy: Is it ready for clinical practice? Journal of Allergy and Clinical Immunology-in Practice, 1(1), 15–21. doi: https://doi.org/10.1016/j.jaip.2012.10.009
- Schmitt, D. A., Nesbit, J. B., Hurlburt, B. K., Cheng, H. P., & Maleki, S. J. (2010). Processing can alter the properties of peanut extract preparations. Journal of Agricultural and Food Chemistry, 58(2), 1138–1143. doi: https://doi.org/10.1021/jf902694j
- Sen, M., Kopper, R., Pons, L., Abraham, E. C., Burks, A. W., & Bannon, G. A. (2002). Protein structure plays a critical role in peanut allergen stability and may determine immunodominant IgE-binding epitopes. Journal of Immunology, 169(2), 882–887. doi: https://doi.org/10.4049/jimmunol.169.2.882
- Sorrentino, A., Giosafatto, C. V. L., Sirangelo, I., De Simone, C., Di Pierro, P., Porta, R., & Mariniello, L. (2012). Higher susceptibility to amyloid fibril formation of the recombinant ovine prion protein modified by transglutaminase. Biochimica Et Biophysica Acta-Molecular Basis of Disease, 1822(10), 1509–1515. doi: https://doi.org/10.1016/j.bbadis.2012.06.003
- Stanley, J. S., King, N., Burks, A. W., Huang, S. K., Sampson, H., Cockrell, G., … Bannon, G. A. (1997). Identification and mutational analysis of the immunodominant IgE binding epitopes of the major peanut allergen Ara h 2. Archives of Biochemistry and Biophysics, 342(2), 244–253. doi: https://doi.org/10.1006/abbi.1997.9998
- Takagi, S., Akashi, M., & Yasumatsu, K. (1979). Determination of hydrostatic region in soybean globulin. Nippon Shokuhin Kogyo Gakkaishi, 26(3), 133–138. doi: https://doi.org/10.3136/nskkk1962.26.3_133
- Tian, Y., Rao, H., Tao, S., & Xue, W. T. (2018). Effect of boiling on the structure and immunoreactivity of recombinant peanut protein Ara h 1. Food and Agricultural Immunology. doi:10.1080/09540105.2018.1461812
- Wasserman, R. L., Hague, A. R., Pence, D. M., Sugerman, R. W., Silvers, S. K., Rolen, J. G., & Herbert, M. (2018). Real world experience with peanut oral immunotherapy: Lessons learned from 270 patients. The Journal of Allergy and Clinical Immunology. In Practice, doi: https://doi.org/10.1016/j.jaip.2018.05.023
- White, B. L., Gokce, E., Nepomuceno, A. I., Muddiman, D. C., Sanders, T. H., & Davis, J. P. (2013). Comparative proteomic analysis and IgE binding properties of peanut seed and testa (skin). Journal of Agricultural and Food Chemistry, 61(16), 3957–3968. doi: https://doi.org/10.1021/jf400184y
- Wu, Z. H., Lian, J., Han, Y. L., Zhou, N. L., Li, X., Yang, A. S., … Chen, H. B. (2016). Crosslinking of peanut allergen Ara h 2 by polyphenol oxidase: Digestibility and potential allergenicity assessment. Journal of the Science of Food and Agriculture, 96(10), 3567–3574. doi: https://doi.org/10.1002/jsfa.7542
- Wu, Z. H., Lian, J., Zhao, R. F., Li, K., Li, X., Yang, A. S., … Chen, H. B. (2017). Ara h 2 cross-linking catalyzed by MTGase decreases its allergenicity. Food & Function, 8(3), 1195–1203. doi: https://doi.org/10.1039/c6fo01365a
- Wu, Z., Zhao, R., Ren, L., Li, X., Yang, A., Tong, P., & Chen, H. (2017). Modification of the reaction system of Ara h 2 catalyzed by MTGase: Products and reaction conditions analysis. Journal of Food Processing and Preservation, e13422. doi: https://doi.org/10.1111/jfpp.13422