ABSTRACT
Anti-AMP scFv were prepared by cloning variable region genes (VH and VL) from hybridoma cell line which secretes antibodies against AMP, and phoA gene was cloned from Escherichia coli strain K12 chromosomal DNA. The resulting scFv and phoA gene were inserted into the expression vector pBV220 to express the scFv–AP fusion protein in E. coli strain BL21. The purified scFv-AP fusion protein was used to develop an ic-CLEIA method for detection of AMP. The limit of detection (IC15) was 0.11 ± 0.004 ng/mL and the IC50 was 0.75 ± 0.04 ng/mL, respectively. The assay was 3 times as sensitive as the corresponding ic-ELISA based on the same fusion protein. IC-CLEIA was used to analyse AMP spiked milk samples, and the validation was confirmed by HPLC. Good correlation between ic-CLEIA and HPLC data was obtained (R2 = 0.9934). It indicated that the developed method can apply to effectively monitor food safety.
1. Introduction
Ampicillin is one of the most important antibiotics commonly used in veterinary medicine to treat bacterial infections. The presence of ampicillin in the food chain can adversely affect the health of human consumers (e.g. by inducing allergic reactions and dysbacterioses) (Hasegawa et al., Citation2003; Khan, Mohd, Bano, Siddiqi, & Asiri, Citation2015; Ubukata et al., Citation2001). In order to prevent the negative impact of ampicillin residues on human health and the entire ecosystem, Governmental agencies have set limitations on the levels of residues in recent years in most countries of the world. Therefore, the need for an efficient detection method to monitor its overuse in animal production is urgent (Byzova, Zvereva, Zherdev, & Dzantiev, Citation2011; Sun et al., Citation2016). Several traditional methods for detection of ampicillin, such as HPLC (Lauback, Rice, Bleiberg, Muhammad, & Hanna, Citation2006; TolhurstA, Negrusz, Libelt, Woods, & Levine, Citation1996), electrochemical (Wang et al. Citation2017a), LC–MS (Hamamoto & Mizuno, Citation2015) and immunoassays (Cliquet, Goddeeris, Okerman, & Cox, Citation2007; Peng et al., Citation2013), have been reported. The instrumental methods are sensitive, but they are not suitable for screening a large number of samples. In comparison, immunoassays such as enzyme-linked immunosorbent assay (ELISA) are rapid, cost-effective, high-throughput, and in some cases capable of working on-site or remotely online. It is a simple tool for detection and quantification of low-molecular-weight contaminants.
A few antibodies specific for ampicillin have been developed over the yeas. However, these studies used either polyclonal antibody (pAb) (Sun, Liu, & Qu, Citation2017; Wang, Zhang, Ni, Zhang, & Shen, Citation2014) or a monoclonal antibody (mAb) (Hu, Huang, Jiang, Fang, & Yang, Citation2010; Liu, Zhao, & Wang, Citation2013), the required polyclonal antibodies (pAb) and monoclonal antibodies (mAb) used in ELISA always involve some problems such as low specificity (Li, Liu, Wang, Chai, & Zhang, Citation2011; Shi, Cheng, Yan, Wu, & Liu, Citation2014), uneven quality for pAb or long period, high cost, complex procedure for mAb production. Recently, advances in recombinant DNA technology have provided an alternative approach by allowing the engineering of recombinant antibodies (RAbs) with desirable affinity and specificity (Fields et al., Citation2013; Maragos, Li, & Chen, Citation2012; Wang et al. 2017). It has several advantages over conventional antibody production, including the low cost and mass-produced by fermentation in Escherichia coli (He et al., Citation2017). More importantly, its single-chain makes it easy to fuse with other proteins, resulting in the formation of antibody molecules with two or more desired functions. To shorten the analysis time and avoid the use of chemically conjugated secondary antibodies, the analyte is directly detected with scFv fused with an enzyme, such as alkaline phosphatase (AP),by means of a biotechnology method to yield a homogeneous and stable bifunctional antibody. RAbs fused with AP are good reagents for immunoassays, particularly for chemiluminescent enzyme immunoassays (CLEIA).
In this work, a broad-specificity recombinant single-chain Fv antibody (scFv) against AMP was produced by a simple method. The scFv gene and AP gene (cloned from Escherichia coli strain K12 chromosomal DNA) were inserted into the expression vector pBV220 to prepare a bifunctional fusion protein (scFv−AP). Using the purified anti-AMP scFv-AP fusion protein, we developed a CLEIA protocol for detection of AMP in milk, and satisfactory results were obtained.
2. Materials and methods
2.1. Materials
Standard ampicillin, the related penicillins and the antibacterial AP mAb were purchased from Sigma Co. (St. Louis, MO, USA). GoScript reverse transcription system was purchased from Promega Co. (Madison. WI, USA). Escherichia coli strains JM109 was purchased from Solarbio Co. (Beijing, China). The pBV220 vector used for soluble protein expression was purchased from Miaolin bio Co. (Wuhan, China). The oligonucleotide primers were synthesized by Shanghai Sangon Biological Engineering Technology & Services Co. (Shanghai, China) and used for polymerase chain reaction (PCR) cloning of the variable genes. Restriction enzymes EcoRI and BamHI were purchased from Takara Co. (Dalian, China). Pfu DNA polymerase and T4 DNA ligase were provided by TransGen Biotechnology Co. (Beijing, China). DNA gel extraction kit, The RNeasy Midi kit, Oligotex mRNA Mini Kit and QIA quick PCR Purification Kit were purchased from Qigen company (Duesseldorf, Germany). Hybridoma cell 9B4C7E, which secretes anti-AMP mAb, was established in our laboratory previously (Hybridoma cell 9B4C7E were from the fusion of splenocyte and myeloma, the specificity of its secretion mAb is 1.54 ± 0.08 ng/mL). Other chemicals and reagents were obtained from Sigma (St. Louis, MO, USA).
2.2. Cloning of variable region genes and the scFv gene assembly
Total RNA was isolated from a hybridoma secreting an anti-AMP antibody using RNeasy mini kit. After reverse transcription from RNA using a reverse transcription PCR kit, the first cDNA was synthesized as a template, and the VL and VH genes were amplified by separate PCR primers (VH-back:5′-CAGGTGCAGCTGCAGGAG-3′,VH-forward:5′-ACGGTCACCGTCTCCTCA-3′.
VL-BACK:5′-GACATTGAGCTCACCCAGTCTCCA-3′,VL-Forward:5′-CCAAGCTGGAAATCAAACGGT-3′). The PCR protocol involved an initial denaturation at 94°C for 5 min, followed by 25 cycles at 94°C for 1 min, 55°C for 1 min, and 72°C for 1 min, with a final extension at 72°C for 10 min. After purification and quantification, the equal VH and VL DNAs were spliced together by overlap extension to generate the scFv gene with a (Gly4Ser)3 linker peptide. The PCR protocol for the overlap extension step involved an initial denaturation at 94 °C for 5 min, followed by 10 cycles at 94°C for 45 s, 50°C for 1 min, and 72°C for 1 min, with a final extension at 72°C for 10 min., Finally, the gene for the scFv fragment containing the EcoRI and BamHI sites was amplified using the primers VL–forward–BamHI and VH–back–EcoRI with the same PCR protocol as used for cloning of VL and VH genes. The amplification fragments were purified with an agarose gel DNA purification kit (He et al. Citation2016a, Citation2016b).
2.3. Construction of a plasmid encoding the scFv–AP fusion protein
The recombinant plasmid encoding the scFv−AP fusion protein (pBV220–scFv–phoA) is shown in . The purified scFv PCR product and pBV220 vector were both digested using EcoRI and BamHI restriction digestion enzymes. After the ligation reaction, the recombinants were transformed into E. coli strain JM109 competent cells, and positive colonies were used for restriction enzyme analysis. The phoA genes were amplified with PCR primers AP-back (5′-GAGGGATCCCGGACACCAGAAATGCC-3′) and AP-forward (5′-GCGGTCGACTTATTTCAGCCCCAGAGCG-3′). After amplification, the products were purified with an agarose gel DNA purification kit and digested with BamHI and SalI restriction enzymes, and vector pBV220-scFv was precut with the same enzymes. The digested products were purified again and then ligated using T4 DNA ligation enzyme at 16°C for 16 h. The ligation product was introduced into JM109 competent cells and grown at 37°C on selective LB-Agar plates containing 100 μg/mL ampicillin. Positive clones were characterized through BamHI and SalI enzyme digestions and sequence analysis (Ossysek, Uchański, Kulesza, Bzowska, & Klaus, Citation2015; Zhang, He, Zhao, Wang, & Jin, Citation2016).
2.4. Expression and purification of the scFv–AP fusion protein
The recombinant plasmid (scFv–pBV220–phoA) was extracted and transformed into E. coli BL21 competent cells. The transformed colony was then cultured in in LB (Luria–Bertani) medium supplemented with 100 μg/mL ampicillin at 37°C until the OD600 reached approximately 0.8−1.0. The promoter was induced by adding 2 mmol/L of IPTG. The culture was further incubated at 25°C with shaking at 180 r/min overnight, followed by centrifugation at 10,000 r/min for 10 min at 4°C, and the scFv–AP present in the periplasm was extracted using the cold osmotic shock method. The recombinant proteins were purified using a Ni-NTA agarose resin column, according to the manufacturer’s instructions. Subsequently, the fusion protein was detected in both SDS−PAGE.
2.5. Development of ic-ELISA and ic-CLEIA protocols using AP difunctional gene
2.5.1. Ic-ELISA protocol
5 μg of AMP-OVA in coating buffer (35 mmol/L NaHCO3, 15 mmol/L Na2CO3; pH 9.6) were coated into 96 microplates wells overnight at 4°C. The plate was washed three times with PBST (PBS containing 0.1% Tween-20), and then blocked with 5% skim milk in PBS buffer (200 μL/well) for 1 h at 37°C. After washing four times with PBST solution, 50 μL scFv–AP diluted with PBS and 50 μL AMP (0, 0.25, 0.5, 1.5, 3, 8, 20 µg/mL) were added to each well at 37°C for 1 h. After the plates were washed four times with PBST, 100 µL of AP–substrate (10 mmol/L MgCL2, 50 mmol/L ZnCl2, 1 mmol/L p-nitrophenyl phosphate, 1 mol/L Tris–HCl; pH 8.0) was added to each well. The reaction was stopped using 3 mol/L NaOH after the mixture was incubated at 37°C for 30 min. Absorbance values were measured at 405 nm using an ELISA plate reader.
2.5.2. Ic-CLEIA protocol
7 μg of AMP-OVA in coating buffer (35 mmol/L NaHCO3, 15 mmol/L Na2CO3; pH 9.6) were coated into 96 microplates wells overnight at 4°C. The plate was washed three times with PBST (PBS containing 0.1% Tween-20), and then blocked with 5% skim milk in PBS buffer (200 μL/well) for 1 h at 37°C. After washing four times with PBST solution, 50 μL scFv–AP diluted with PBS and 50 μL AMP (0.05, 0.15, 0.3, 1.5, 3.5, 10 ng/mL) were added to each well at 37°C for 1 h. After the plates were washed four times with PBST. 100 µL of AP–CLEIA substrate (50 mmol/L carbonate buffer containing 1 mmol/L MgCl2 and 0.67 mmol/L AMPPD) was added to each well. The chemiluminescent signal was measured with the multilabel counter after 30 min incubation at room temperature in the dark.
2.6. Specificity characterizations of soluble scFv–AP
The specificity of ic-CLEIA was evaluated based on the scFv–AP fusion protein by performing competitive assays using several compounds structurally related to ampicillin as competitors, and the obtained IC50 values were used to calculate cross-reactivity using the formula:
2.7. Analyses of spiked samples
The milk samples obtained from a local Yonghui supermarket were chosen to evaluate the performance of ic-CLEIA for detection of AMP. AMP-free milk samples were centrifuged at 5000 r/min for 5 min to remove the fat and precipitate, and the supernates were spiked with an ampicillin standard solution (5, 10 or 20 ng/mL). The spiked samples were allowed to stand at 4°C for 30 min. The samples were transferred to a clean test tube and diluted with 10 volumes of PBS. The diluted solution was analysed directly by ic-CLEIA. Also, the matrix effects determination was run according to the procedure described above using pure extract to dilute the AMP. The percentage of recovery was calculated as follows:
2.8. Validation study of ic-CLEIA
A validation study of ic-CLEIA was performed by high-performance liquid chromatography (HPLC). The milk samples obtained from a local Yonghui supermarket were confirmed to be free of AMP by HPLC, and then were spiked with ampicillin (5, 10 or 20 ng/mL). Liquid-liquid extraction and further solid phase clean up were used to eliminate the matrix effects and enrich the ampicillin. The extract was divided into two equal aliquots, one was used for ic-CLEIA analysis, the other was used for HPLC analysis. The extraction and HPLC procedures were described as follows:
Briefly, the milk samples were homogenized using acetonitrile (CAN). Potassium borohydride serving as a reductive agent was added to the crude extract. Followed by liquid-liquid extraction with dichloromethane, the lower organic layer was then collected and dried. The residue was redissolved in ACN and loaded to the PRS solid phase extraction columns for further cleanup.
3. Results and discussion
3.1. Cloning of VH, VL genes and assembly of the scFv genes
The VH and VL (heavy chain and light chain) genes were amplified using PCR technique from the hybridoma cell line 9B4C7E. The VH and VL gene amplified products formed bands at expected about 370 and 340 bp size on an agarose gel respectively ((A)). The VL and VH genes were amplified to generate the enzyme site and linker overhang and then assembled by splicing overlap extension PCR to generate the scFv genes. PCR assembly and amplification of scFv fragment produced an expected about 760 bp size ((B)). The results indicated that the scFv genes were successfully generated.
3.2. Construction of a plasmid of pBV220–scFv–phoa
To obtain the scFv–AP fusion protein, the pBV220–scFv–phoA plasmid was constructed after inserting the PCR product of the AP coding sequence into pBV220-scFv vector between the BamHI and SalI sites. The recombinant plasmid pBV220–scFv–phoA was then identified using PCR and restriction enzyme digestion (). PCR analysis using plasmid pBV220–scFv–phoA as a template obtained a band of the expected 1400 bp size (, lane 3). Digestion of the recombinant plasmid using BamHI or SalI shown a single band with the expected 5700 bp size (, lanes 4 and 5), and digestion using BamHI and SalI shown two bands of 4300 and 1400 bp (, lane 2). The nucleotide sequence of recombinant scFv-phoA was shown in Fig S1. As expected, The results of sequence were correct. All these indicated that recombinant plasmid pBV220–scFv–phoA had been successfully generated.
Figure 3. The recombinant plasmid pBV220–scFv–phoA identification using PCR and the digestion on agarose gel electrophoresis. Lane M1, DNA Marker 2000; lane M2, k-HindIII digest DNA Marker 23130; lane 1, purification phoA gene; lane 2, BamHI and SalI digestion product of plasmid pBV220–scFv–phoA; lane 3, PCR product of plasmid pBV220–scFv–phoA; lane 4, BamHI digestion product of plasmid pBV220–scFv–phoA; lane 5, SalI digestion product of plasmid pBV220–scFv–phoA.
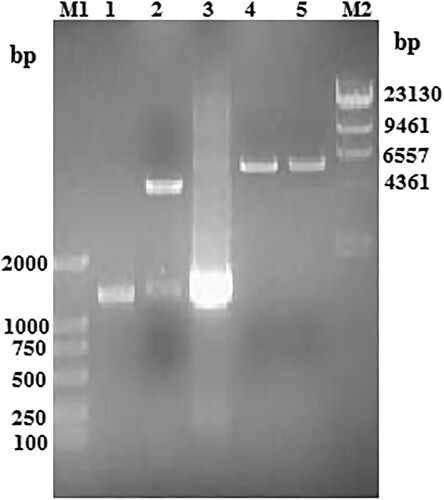
3.3. Expression of soluble scFv–AP fusion protein
In order to obtain the target protein, The positive recombinant plasmid pBV220–scFv–phoA was used to transform E. coli BL21 competent cells. When the OD600 reached 0.8–1.0, Protein expression was induced by the addition of IPTG and the culture grown overnight at 25°C, and then bacterial extraction was prepared using means of cold osmotic shock (Dong et al., Citation2012). To confirm the expression of the target protein, SDS–PAGE analysis was performed for fusion Protein in , a soluble protein of ∼75 kDa was expressed as expected by SDS–PAGE analysis. Judging from the molecular-weight, this band was the target fusion protein scFv–AP.
3.4. Comparison of ic-CLEIA and ic-ELISA using scFv-AP
To further determine the purified anti–AMP scFv–AP especially not only its bifunctionality but also its sensitivity to detect AMP, an ic-ELISA and ic-CLEIA were both developed. The result was showed in . The average concentration of AMP required for 50% inhibition of binding (IC50) and LOD (IC15) of ic-CLEIA were 0.75 ± 0.04 (n = 3) and 0.11 ± 0.004 ng/mL (n = 3), respectively, but the IC50 and IC15 of ic-ELISA were 1.92 ± 0.06 ng/mL and 0.18 ± 0.03 ng/mL, respectively. The sensitivity of ic-CLEIA was about three times that of ic-ELISA, and The LOD (IC15) was two times that of ic-ELISA. What’s more, the sensitivity is higher than the previously reported ELISA using an anti-AMP polyclonal antibody and monoclonal antibodies by comparion of IC50 (Li et al., Citation2011; Ma, Zhao, Qiu, Qin, & Yang, Citation2013; Shi et al., Citation2014). Cross-reactivity was performed using AMP analogs based on ic-CLEIA to determine the specificity of obtained fusion protein (). The cross-reactivity values of scFv–AP with other analogs were below 4%. This indicated that the fusion protein was no cross-reactivity with AMP analogs.
Figure 5. AMP standard curves obtained obtained with the scFv–AP fusion protein in ic-CLEIA and ic-ELISA under optimized conditions (n = 3).
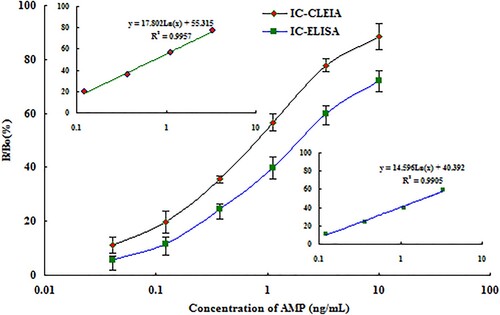
Table 1. Cross-reactivity of the scFv–AP fusion protein with other AMP analogs using ic-CLEIA.
Compared with traditional pAb or mAb, the approach using gene fusion to prepare antibody is cheap and reproducible (with the ability to store bacterial cells indefinitely), and it produces a homogeneous target protein in contrast to traditional antibody production operation. The production of an expressed protein can be adapted to industrial scale and be rapidly purified using affinity chromatography. Moreover, The developed ic-CLEIA not only can avid to use enzyme-labeled secondary antibody and reduced one washing step compared with the traditional ic-ELISA, but also can save time. These results strongly suggest this expression system has an advantage for the production of scFv–AP fusion protein used in a rapid detection immunoassay.
3.5. Analyses of spiked samples
According to the data, the matrix effects of milk extract samples were almost eliminated after extract diluted 1:10. The developed ic−CLEIA was applied to analyze milk samples spiked with three concentrations of AMP (5, 10 or 20 ng/mL). As shown in , the mean recoveries of AMP were in the desirable range of 99–104.4% for milk samples. Coefficients of variation (CV) ranging from 7.74–13.8% were obtained. These results demonstrate that the developed ic-CLEIA method can be used in the determination of AMP residues in food samples.
Table 2. Recovery of AMP from spiked milk samples using method of ic-CLEIA (n = 3).
3.6. Validation study of ic-CLEIA
Milk samples were spiked with AMP at 5, 10, 20 ng/mL, respectively. After sample extraction and clean-up on a solid-phase extraction column, the purified extract was analyzed by HPLC and ic-CLEIA. The analytical results obtained with the two methods for the same extract were shown in . Good correlations (R2 = 0.9934) were obtained for the samples. The results indicate that the developed ic-CLEIA can be used as a new immunoassay detection method for AMP in food samples.
4. Conclusion
In this study, the anti–AMP scFv and AP genes were successfully cloned and then plasmid pBV220–scFv–phoA was constructed. After induction, The anti–AMP scFv–AP recombinant fusion protein was expressed in E. coli strain BL21. On basis of the scFv–AP antibodies. We developed a new immunoassay method (ic-CLEIA) for AMP. Compared with a traditonal immunoassay (ic-ELISA), the developed ic-CLEIA method showed many advantages in detection AMP in food samples. In brief, as an alternative to pAb or mAb, the recombinant scFv–AP fusion protein could be used to rapidly and effectively guarantee food safety using ic-CLEIA method.
Supplemental Material
Download ()Disclosure statement
No potential conflict of interest was reported by the authors.
Additional information
Funding
References
- Byzova, N. A., Zvereva, E. A., Zherdev, A. V., & Dzantiev, B. B. (2011). Immunochromatographic technique for express determination of ampicillin in milk and dairy products. Applied Biochemistry and Microbiology, 47, 685–693. doi.org/10.1134/S0003683811060032
- Cliquet, p., Goddeeris, B. M., Okerman, L., & Cox, E. (2007). Production of penicillin-specific polyclonal antibodies for a group-specific screening ELISA. Food and Agricultural Immunology, 18, 237–252. doi.org/10.1080/09540100701802908
- Dong, J. X., Li, Z. F., Lei, H. T., Sun, Y. M., Ducancel, F., Xu, Z. L., & Wang, H. (2012). Development of a single-chain variable fragment-alkaline phosphatase fusion protein and a sensitive direct competitive chemiluminescent enzyme immunoassay for detection of ractopamine in pork. Analytica Chimica Acta, 736, 85–91. doi.org/10.1016/j.aca.2012.05.033
- Fields, C., O’Connell, D., Xiao, S., Lee, G. U., Billiald, P., & Muzard, J. (2013). Creation of recombinant antigen-binding molecules derived from hybridomas secreting specific antibodies. Nature Protocols, 8, 1125–1148. doi.org/10.1038/nprot.2013.057
- Hamamoto, K. K., & Mizuno, Y. (2015). LC-MS/MS measurement of ampicillin residue in swine tissues at 5 days after in-feed administration. Journal of Veterinary Medical Science, 77, 1527–1529. doi.org/10.1292/jvms.14-0367
- Hasegawa, K., Yamamoto, K., Chiba, N., Kobayashi, R., Nagai, K., & Jacobs, M. R. (2003). Diversity of ampicillin-resistance genes in Haemophilus influenzae in Japan and the United States. Microbial Drug Resistance, 9, 39–46. doi.org/10.1089/107662903764736337
- He, X., Duan, C. F., Qi, R. H., Dong, J., Wang, G. N., Zhao, G. X., … Liu, J. (2017). Virtual mutation and directional evolution of anti-amoxicillin ScFv antibody for immunoassay of penicillins in milk. Analytical Biochemistry, 517, 9–17. doi.org/10.1016/j.ab.2016.10.020
- He, K., Zhang, X. Y., Du, X. J., & Wei, D. (2016a). Production of a soluble single-chain variable fragment antibody against okadaic acid and exploration of its specific binding. Analytical Biochemistry, 503, 21–27. doi.org/10.1016/j.ab.2015.12.020
- He, K., Zhang, X. Y., Zhao, R. P., Wang, L. X., Feng, T. T., & Wei, D. (2016b). An enzyme-linked immunosorbent assay and a gold-nanoparticle based immunochromatographic test for amatoxins using recombinant antibody. Microchimica Acta, 183, 2211–2219. doi.org/10.1007/s00604-016-1856-x
- Hu, K., Huang, X. Y., Jiang, Y. S., Fang, W., & Yang, X. L. (2010). Monoclonal antibody based enzyme-linked immunosorbent assay for the specific detection of ciprofloxacin and enrofloxacin residues in fishery products. Aquaculture, 310, 8–12. doi.org/10.1016/j.aquaculture.2010.08.008
- Khan, A. A. P., Mohd, A., Bano, S., Siddiqi, K. S., & Asiri, A. M. (2015). Spectrophotometric methods for the determination of ampicillin by potassium permanganate and 1-chloro-2,4-dinitrobenzene in pharmaceutical preparations. Arabian Journal Chemistry, 8, 255–263. doi.org/10.1016/j.arabjc.2012.04.033
- Lauback, R. G., Rice, J. J., Bleiberg, B., Muhammad, N., & Hanna, S. A. (2006). Specific high-performance liquid chromatographic determination of ampicillin in bulks, injectables, capsules, and oral suspensions by reverse-phase ion-pair chromatography. Food and Agricultural Immunology, 7, 1243–1265. doi.org/10.1080/01483918408074041
- Li, Q. M., Liu, Q. T., Wang, Y. B., Chai, S. J., & Zhang, G. P. (2011). Anti-ampicillin monoclonal antibodies and their cross-reactivity to penicillin antibiotics. Acta Agriculturae Boreali-Sinica, 26, 60–63. http://www.hbnxb.net/EN/article/searchArticle.do
- Liu, Y. Z., Zhao, G. X., & Wang, P. (2013). Production of the broad specific monoclonal antibody against sarafloxacin for rapid immunoscreening of 12 fluoroquinolones in meat. Journal of Environmental Science and Health, 48, 139–146. doi.org/10.1080/03601234.2013.727668
- Ma, L. P., Zhao, J. F., Qiu, Z. J., Qin, C. L., & Yang, M. L. (2013). Synthesis of ampicillin complete antigen and the preliminary optimization of indirect competitive ELISA conditions. Animal Husbandry and Feed Science, 34, 10–13. http://www.doc88.com/p-6901156224273.html
- Maragos, C. M., Li, L., & Chen, D. H. (2012). Production and characterization of a single chain variable fragment (scFv) against the mycotoxin deoxynivalenol. Food and Agricultural Immunology, 23, 51–67. doi.org/10.1080/09540105.2011.598921
- Ossysek, K., Uchański, T., Kulesza, M., Bzowska, M., & Klaus, T. (2015). A new expression vector facilitating production and functional analysis of scfv antibody fragments selected from tomlinson I+J phagemid libraries. Immunol.Lett, 167, 95–102. doi.org/10.1016/j.imlet.2015.07.005
- Peng, J., Cheng, G., Li, H., Wang, Y., Hao, H., Peng, D., … Yuan, Z. (2013). Development of a direct ELISA based on carboxy-terminal of penicillin-binding protein BlaR for the detection of β-lactam antibiotics in foods. Analytical and Bioanalytical Chemistry, 405, 8925–8933. doi.org/10.1007/s00216-013-7311-5
- Shi, L., Cheng, H., Yan, J. H., Wu, M., & Liu, J. Z. (2014). Synthesis of ampicillin artificial antigen and preparation of anti-ampicillin monoclonal antibodies. Animal Husbandry & Veterinary Medicine, 46, 15–18. https://wenku.baidu.com/view/d8c98f49376baf1ffc4fad73.html
- Sun, L. R., Jia, L. F., Xie, X., Xie, K. Z., Wang, J. F., Liu, J. Y., & Wang, J. Y. (2016). Quantitative analysis of amoxicillin, its major metabolites and ampicillin in eggs by liquid chromatography combined with electrospray ionization tandem mass spectrometry. Food Chemistry, 192, 313–318. doi.org/10.1016/j.foodchem.2015.07.028
- Sun, W. Y., Liu, W. Y., & Qu, L. B. (2017). Development of ELISA and immunochromatographic assay for ofloxacin. Chinese Chemical Letters, 18, 1107–1110. doi: 10.1016/j.cclet.2007.07.008
- TolhurstA, T. A., Negrusz, A., Libelt, B., Woods, E. F., & Levine, B. S. (1996). Determination of ampicillin in New Zealand white rabbit plasma using column switching technique and HPLC. Chromatographia, 42, 223–226. doi.org/10.1007/BF02269657
- Ubukata, K., Shibasaki, Y., Yamamoto, K., Chiba, N., Hasegawa, K., & Takeuchi, Y. (2001). Association of amino acid substitutions in penicillin-binding protein 3 with beta-lactam resistance in betalactamase-negative ampicillin-resistant Haemophilus influenzae. Antimicrobial Agents and Chemotherapy, 45, 1693–1699. doi.org/10.1128/AAC.00960-06 doi: 10.1128/AAC.45.6.1693-1699.2001
- Wang, B., Pang, M., Xie, X., Zhao, M., & Xie, K. (2017a). Quantitative analysis of amoxicillin, amoxicillin major metabolites, and ampicillin in chicken tissues via ultra-performance liquid chromatography-electrospray ionization tandem mass spectrometry. Food Analytical Methods, 10, 3292–3305. doi.org/10.1007/s12161-017-0900-8
- Wang, D. D., Yue, Y. H., Wu, G. M., Tian, Y., Liu, Y. L., Yu, J., … Zhang, G. L. (2017b). Preparation and characterization of a human scFv against the Clostridium perfringens type A alpha-toxin. Toxicon, 130, 79–86. doi.org/10.1016/j.toxicon.2017.02.021
- Wang, Z. H., Zhang, H. Y., Ni, H. J., Zhang, S. X., & Shen, J. Z. (2014). Development of a highly sensitive and specific immunoassay for enrofloxacin based on heterologous coating haptens. Anal.Chim.Acta, 820, 152–158. doi.org/10.1016/j.aca.2014.02.043
- Zhang, X. Y., He, K., Zhao, R. P., Wang, L. X., & Jin, Y. D. (2016). Cloning of scFv from hybridomas using a rational strategy: Application as a receptor to sensitive detection microcystin-LR in water. Chemosphere, 160, 230–236. doi: 10.1016/j.chemosphere.2016.06.084