ABSTRACT
In this study, we used 2-(diethexyphosphoryl)acetic acid as a common template molecule and Fe3O4@SiO2 as support material to prepare a superparamagnetic core/shell molecular imprinting polymer (MIP), Fe3O4@SiO2@MIP, which has multiple recognition sites and increased adsorption capacity. Then, a biomimetic fluorescence immunoassay method was developed for the simultaneous determination of methyl parathion, chlorpyrifos, and trichlorfon using the Fe3O4@SiO2@MIP as biomimetic antibody and quantum dots as label. The factors that influenced the performance of this method were optimized. Under optimal conditions the limits of detection of the method were 0.21 ± 0.021, 0.44 ± 0.069 and 0.32 ± 0.033 μg/L for methyl parathion, chlorpyrifos, and trichlorfon, respectively. To evaluate the accuracy of the method, apple and orange samples spiked with methyl parathion, chlorpyrifos, and trichlorfon were analysed, and the recoveries ranged from 73.1% to 119.3%. Finally, this method was applied to detect three pesticides in pear, carrot, kiwifruit, and banana samples, and the results indicated a good correlation with those obtained from gas chromatography method.
Introduction
Organophosphorus pesticides are commonly used in agriculture because of their high insecticidal efficiency, low cost and high selectivity. Nevertheless, their residues can enter the human body through the atmosphere, water, soil, and food, and cause various chronic or acute diseases (Pan et al., Citation2016). Therefore, organophosphorus pesticides are major organic pollutants found in foodstuff. Regulatory authorities have stipulated that any pesticides in or on food must be within established maximum residue limits as determined via monitoring programmes (Thanhdong, Yu, Daemyung, & Gaeho, Citation2008). To satisfy this requirement, rapid and sensitive methods for multi-residue pesticide analysis are urgently needed (Liu, Ge, et al., Citation2012).
Current methods for the determination of multi-residue pesticide are usually based on gas chromatography coupled to mass spectrometry (GC-MS) (Cheng et al., Citation2017; Fernandes, Subramanian, Mateus, Domingues, & Deleruematos, Citation2012; Kolberg, Prestes, Adaime, & Zanella, Citation2011; Taghani, Goudarzi, Bagherian, Chamjangali, & Amin, Citation2018), GC with flame photometric detection (GC-FPD) (Farajzadeh, Mogaddam, Aghdam, Nouri, & Bamorrowat, Citation2016; Ronald Erney, Citation2015; Yang et al., Citation2012), liquid chromatography coupled with tandem mass spectrometry (Harshit, Charmy, & Nrupesh, Citation2017; Hu et al., Citation2017). However, these methods require expensive equipment and lengthy analytical times. Hence it is important to develop and explore alternative technologies that are rapid, affordable, and simple.
Immunoassays are an alternative pesticide assay because of their simplicity and cost-effectiveness (He & Ming-Tao, Citation2012). Most immunosorbent assay methods are normally used for the single pesticide detection or independent detection of a variety of pesticides. However, agricultural products contain many organophosphorus pesticides simultaneously. Therefore, current work in pesticide immunoassays focuses on multi-analyte immunoassays.
One of the most popular strategies for achievement of multi-analyte immunoassays is the synthesis of antibodies with affinity for a variety of organophosphorus pesticides (Liang, Xie, Wang, Gui, & Zhu, Citation2013; Liu et al., Citation2012). However, most common antibodies are obtained using immunogenic animals, which is complicates acquisition (Wang, Li, & Liu, Citation2010). Furthermore, the biological antibodies are sensitive and unstable to the temperature and pH. Therefore, their application is limited. To overcome this drawback, the preparation of molecularly imprinted polymer (MIP) that can selectively recognize multiple pesticides and has good stability to be used as a biomimetic antibody is an alternative method (Hu, Shen, Zhu, & Jiang, Citation2010; Song, Zhang, Wang, & Wang, Citation2016; Tang, Fang, Wang, Sun, & Qian, Citation2013).
Fluorescence immunoassay technology, one of the most commonly used immunoassay formats, has the advantages of high specificity and sensitivity, and good practicability (Hu et al., Citation2016). Quantum dots (QD) are photoluminescent semiconductor nanocrystals with excellent fluorescence characteristics, including narrow, symmetric, bright, and photostable fluorescent emission (He, Lu, et al., Citation2011; He, Zhong, et al., Citation2011). As a result, it has been increasingly used as a fluorescent label in fluorescence immunoassays (FIA) (Hou et al., Citation2016; Tao, Yong, Zhu, & Lei, Citation2016; Zhang et al., Citation2017). Furthermore, FIA can simultaneously determine multiple organophosphorus pesticides based on QDs via their unique emission wavelengths.
Superparamagnetic core/shell MIPs have attracted extensive attention recently because of their high affinity, selectivity, and stability (Fan, Xu, Xu, Zhang, & Zhu, Citation2015; Tanhaei, Ayati, Lahtinen, & Sillanpää, Citation2015). In this study, 2-(diethexyphosphoryl)acetic acid (DPA) which possessing functional groups that are common to most organophosphorus pesticides was used as a template molecule, Fe3O4@SiO2 was used as support material, and a superparamagnetic core/shell MIP of Fe3O4@SiO2@MIP was synthesized. Using Fe3O4@SiO2@MIP as a biomimetic antibody and QDs with different emission wavelengths as labels, a biomimetic fluorescence immunoassay (BFIA) method that can simultaneously detect methyl parathion, chlorpyrifos, and trichlorfon was developed. The factors affecting the performance of this method were optimized in detail, and the selectivity and applicability of this method were evaluated.
Material and methods
Reagents and chemicals
The apple and orange samples were purchased from a local supermarket (Tai’an, China), and the pear, carrot, kiwifruit, and banana samples were purchased randomly from a market in Tai’an (Shandong, China).
The 2-(diethexyphosphoryl)acetic acid (DPA) was supplied by Bide Pharmatech Ltd. (Shanghai, China). Ferric chloride (FeCl3·6H2O) and iron (II) chloride tetrahydrate (FeCl2·4H2O) were obtained from Tianjin Bodi Chemical Co., Ltd. (Tianjin, China). The 3-aminopropyl triethoxysilane (APTES) and tetraethoxysilane (TEOS) were from Macklin Biochemical Co., Ltd. (Shanghai, China). The QDs (excitation wavelength 380 nm, emission wavelength 525, 585, and 625 nm) were from Jiayuan Quantum Dots Co., Ltd. (Wuhan, China). The analytical standards for methyl parathion (99.6%), chlorpyrifos (99%), and trichlorfon (99%) were supplied by the Institute for the Control of Agrochemicals of Ministry of Agriculture (Beijing, China). Ultrapure water was from an Aike ultrapure water instrument (Tangshi Kangning Technology Company, Chengdu, China) and used throughout the experiments.
Apparatus
UV spectra were recorded using a UV/Vis spectrophotometer (Metash Instruments Co., Ltd., Shanghai, China). FT-IR spectra (4000–500 cm−1) in KBr were obtained from a Vector 22 spectrometer (Bruker, Karlsruhe, Germany). The structure and size of Fe3O4@SiO2@MIP were determined by Transmission Electron Microscope (TEM; JEOL Model JEM-1010, Japan). Fluorescent measurements were done using a Lumina fluorescence spectrometer (Thermo Fisher Scientific, Shanghai, China).
A Shimadzu 2010 gas chromatograph (GC) equipped with a flame photometric detector (Shimadzu, Kyoto, Japan) was used to determine the pesticides in the pear, carrot, kiwifruit, and banana samples. The separation was conducted on an RTX-1701 capillary column (30 m × 250 μm i.d. × 0.1-μm film thickness). Nitrogen was used as the carrier gas at a constant flow rate of 1.00 mL min−1, and the injection volume was 1.00 μL. The injection port temperature was 180°C in split mode with a split ratio of 2:1. The detector temperature was held constant at 250°C. The makeup flow rate was 30.00 mL/min.
Synthesis of haptens
Methyl parathion hapten
The methyl parathion hapten was synthesized according to previous reports (Wang, Ma, Yu, & He, Citation2000). First, 4.00 g of methyl parathion was dissolved in 40 mL of ether, and then extracted with 20 mL of 0.1 mol/L cold Na2CO3 solution for three times. After the water phase was removed, 40 mL of acetic acid/concentrated hydrochloric acid (9/1, V/V) and 8.00 g of zinc powder were added. After stirring for 50 min, the ether layer was extracted twice with 25 mL of ultrapure water and dried over anhydrous sodium sulphate, and then placed in the dark for 12 h to get a yellowish-brown oily matter. Next, 0.5 mol/L hydrochloric acid was added dropwise to the oily solution until the pH value of the solution was 1.0–2.0. The solution was extracted using n-hexane after filtering the insoluble. An orange-yellow oily matter was obtained when the pH of the water layer was adjusted to 10.0–11.0 using a 2.5 mol/L NaOH solution. The mixture was extracted with chloroform for three times, and the organic extract was then dried over anhydrous sodium sulphate. Finally, the brown oily material was obtained using reduced pressure distillation.
Chlorpyrifos hapten
The chlorpyrifos hapten was prepared via a previous method. Briefly, 1.06 g of 3-mercaptopropionic acid (10 mmol) was dissolved in 50 mL ethanol. After 1.42 g of KOH and 50 mL of anhydrous ethanol containing 3.51 g of chlorpyrifos were added, the mixture was then heated and refluxed for 1 h. Next, the mixed solution was filtered, and then concentrated by reduced pressure distillation. The aqueous layer was adjusted to pH 4.0 after adding 50 mL of 5% NaHCO3 and extracting with n-hexane (3×50 mL). After that, the solution was extracted three times with 80, 50, and 50 mL of dichloromethane, respectively. Then, all dichloromethane solutions were pooled and dehydrated using an anhydrous sodium sulphate column. After being concentrated in a vacuum, the residue was studied with column chromatography [n-hexane/tetrahydrofuran/acetic acid, 75/25/1 (v/v/v)]. Fractions showing only one spot on TLC (Rf = 0.41, same solvent mixture) were pooled and concentrated. Finally, light yellow crystals were obtained after recrystallization.
Trichlorfon hapten
The preparation of trichlorfon hapten was followed by our previous study (Jiang, Wu, Xu, Qiao, & Xu, Citation2017). Firstly, 5.66 g of trichlorfon (22 mmol) and 2.00 g of succinic anhydride (20 mmol) were dissolved in 5 mL of anhydrous pyridine. After magnetic stirring for 10 min, the sample was placed in the dark for 18 h, and a yellowish-brown material was formed. This solution was then purged with nitrogen. Next, 50 mL of ultrapure water was added, and the pH was changed to 8.0–9.0 with saturated NaHCO3. The organic phase was evaporated to dryness under reduced pressure after extraction with 30 mL of ethyl acetate for three times, and the light yellow oily product was obtained.
Preparation of QD-labelled haptens
Methyl parathion, chlorpyrifos and trichlorfon haptens were labelled using QDs with different emission wavelengths (525, 585, and 625 nm, respectively). The procedure used our previous method (Jiang et al., Citation2017).
Preparation of Fe3O4@SiO2@MIP
Superparamagnetic Fe3O4 nanoparticles were prepared with a modified co-precipitation method (Fan et al., Citation2015). Briefly, FeCl2·4H2O (0.01 mol) and FeCl3·6H2O (0.02 mol) were dissolved in 150 mL water under nitrogen with vigorous stirring at room temperature. The solution was then gradually heated to 60°C. After 40 mL of sodium hydroxide solution (2.0 mol/L) was added, the mixture was stirred at 60°C for 150 min and then cooled to room temperature. Finally, the black precipitate was collected with a magnet and washed with ethanol and ultrapure water until the pH of the wash solution was 7.0.
The superparamagnetic Fe3O4 nanoparticles were coated with SiO2 as follows: First, 1.00 g of above Fe3O4 magnetic nanoparticles were dissolved in 150 mL ethanol and 30 mL ultrapure water. When 2 mL of ammonium hydroxide and 4 mL of TEOS were added sequentially, the mixture was stirred at 60°C for 6 h. The resultant precipitate was collected by filtration and washed with ultrapure water and ethanol for six times. Finally, the Fe3O4@SiO2 material was dried in a vacuum oven at 60°C for 24 h.
The DPA was grafted to the surface of the Fe3O4@SiO2 particles via the following procedure. Briefly, 0.20 g of DPA (1 mmol) was dissolved in 5 mL of tetrahydrofuran, followed by 0.47 mL of APTES (2 mmol). After stirring for 20 min, 60.00 mg of Fe3O4@SiO2 and 1.18 mL of TEOS (6 mmol) were added, and the mixture was stirred for another 30 min. When 0.3 mL of ammonium hydroxide (0.1 mol/L) was added, the mixed solution was incubated at 60 °C for 10 h. The product was filtered and washed with methanol to remove the unreacted reagents and template molecules and then dried in a vacuum oven at 60°C for 12 h. The product was treated by Soxhlet extraction with 150 mL of methanol/acetic acid (4/1, V/V) for 24 h and then with 150 mL methanol for 12 h to remove DPA. The imprinted polymer was then dried under vacuum at 60°C for 12 h, and the Fe3O4@SiO2@MIP was obtained (). For comparison, a non-molecularly imprinted polymer (NIP), Fe3O4@SiO2@NIP, was synthesized using the above procedure except without a template molecule.
Static adsorption and dynamic adsorption test
To evaluate the binding capacity of the Fe3O4@SiO2@MIP, 20 mg of MIP or NIP was added to 10 mL of methyl parathion, chlorpyrifos, or trichlorfon standard solution (10-60 mg/L) in a methanol/water mixture solution (1/9, V/V). The mixture was vigorously shaken for 4 h at room temperature with a horizontal shaker and then centrifuged at 8000 rpm for 40 min. The absorbance of the supernatant solutions was determined using a UV/Vis spectrophotometer, and the adsorption capacity was calculated.
The uptake kinetics of Fe3O4@SiO2@MIP toward methyl parathion, chlorpyrifos, or trichlorfon were also investigated. Here, 20.00 mg of MIP was added to 10 mL of standard solution at 30 mg/L concentration with three pesticides. The mixtures were shaken for 5, 30, 60, 90, 120, 180, and 240 min followed by adsorption capacity measurement.
BFIA protocol
The procedure of the BFIA was as follows: 1 mL of different QD-labelled haptens and 3 mL of methyl parathion, chlorpyrifos and trichlorfon mixture standard solutions (0.000033–33.33 mg/L) or 3 mL of sample extract were added into a round bottom flask and mixed. Then, 5.00 mg of Fe3O4@SiO2@MIP was added. After shaking for 120 min and centrifuging (8000 r/min) for 15 min, the fluorescence values of the supernatant were measured at λex of 380 nm and λem of 525, 585, and 625 nm. The inhibition rates were calculated respectively.
Sample preparation
To test the feasibility, apple and orange samples which spiked with standard solution were detected by the BFIA method, and they were detected to be free of methyl parathion, chlorpyrifos and trichlorfon by GC prior to spiking. Here, 50.00 g of the apple and orange samples were sliced and homogenized. Then, 5.00 g of each was separately weighed and spiked with 5 mL of the mixed standard solutions of methyl parathion, chlorpyrifos and trichlorfon (5, 25 and 50 mg/L). After incubation at the room temperature for 4 h, the spiked samples were ultrasonicated with 3 × 15 mL methanol/water solution (3/17, V/V) for 30 min, respectively. The extracts were diluted with methanol/water solution (3/17, V/V) to 50 mL and used for the BFIA procedure after filtering with a 0.22-μm filter membrane.
To detect the contents of three pesticides in the apple, orange, pear, carrot, kiwifruit, and banana samples, each sample was prepared similar to the above procedure except for the addition of pesticides. The resulting extracts were studied with BFIA to measure the three pesticides.
Gas chromatography analysis
To identify the accuracy of the BFIA method, the pear, carrot, kiwifruit, and banana samples were also determined with gas chromatography (GC) according to Chinese agricultural standard (NY/T 761-2008). Briefly, 25.00 g of the pear, carrot, kiwifruit, and banana sample homogenate were mixed separately with 50 mL of acetonitrile solutions, and the extracts were pooled into a 100 mL cylinder containing 5–7 g of NaCl and shaken for 1 min. After standing at room temperature for 30 min, 10 mL of the acetonitrile layer was transferred to a beaker. The solution was condensed to dryness at 80°C under a gentle flow of nitrogen, and then accurately redissolved with 5 mL of acetone solution. Finally, the extract was transferred to GC for analysis after filtering with a 0.22-μm filter membrane.
Data analysis
The ANOVA (SAS Institute, Cary, NC, U.S.A) test was used to evaluate the differences between the results obtained with BFIA and GC methods.
Results and discussion
UV scanning of haptens
The abilities of methyl parathion, chlorpyrifos, trichlorfon and their haptens were studied with UV spectrophotometer at 190–400 nm (Figure S1). The three pesticides have characteristic absorption peaks at 275, 230, and 189 nm, respectively, while their haptens have characteristic absorption peaks at 234, 258, and 257 nm, respectively, suggesting that the three pesticide haptens were synthesized successfully.
Characterization of Fe3O4@SiO2@MIP
The FT-IR spectra of (A) DPA, (B) Fe3O4@SiO2@MIP before extraction, (C) Fe3O4@SiO2@MIP after extraction, and (D) Fe3O4@SiO2@NIP are compared in . For Fe3O4@SiO2@MIP before extraction, the vibration band near 1574 cm−1 confirmed the presence of the N-H group from APTES (Liu, Fang, & Wang, Citation2014). For DPA and Fe3O4@SiO2@MIP before extraction, the features around 1725 and 1736 cm−1 indicated a C=O stretch from the COOH of DPA. The stretch shift in the C=O stretching peak was due to the interaction between the C=O of DPA and the N-H of APTES. However in (C), there was no vibration band for C=O group, which indicated that the template molecule of DPA had been removed from the imprinted polymer after extraction. In addition, the Fe-O stretching peak near 780 cm−1 and the Si-O-Si stretching peak near 1030 cm−1 were observed in (B–D), which verified that the surface of Fe3O4 particle was encapsulated by a SiO2 shell, and the MIP was successfully coated onto the surface of the Fe3O4@SiO2 (Fan et al., Citation2015). These results verified that Fe3O4@SiO2@MIP was synthesized.
Figure 2. FT-IR spectra of (A) DPA, (B) Fe3O4@SiO2@MIP before extraction, (C) Fe3O4@SiO2@MIP after extraction, and (D) Fe3O4@SiO2@NIP.
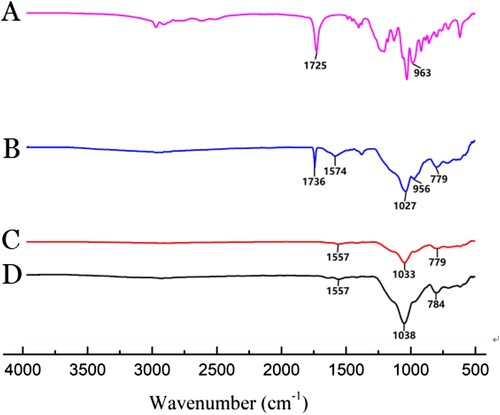
In this experiment, the morphology of Fe3O4@SiO2 (Figure S2A) and Fe3O4@SiO2@MIP (Figure S2B) was characterized by transmission electron microscopy (TEM). As shown in Figure S2B, a core/shell structure was formed in Fe3O4@SiO2@MIP. Thus the adsorption capacity of MIP can be improved. It was revealed that the dispersity of Fe3O4@SiO2 particle was not good. This might be the reason that Fe3O4@SiO2 particles as the cores were encapsulated in MIP shell. The similar phenomena were also found in another study (Fan et al., Citation2015).
The adsorption capacity (Q) of the MIP and NIP was investigated ((A)). The results showed that the adsorption capacity of MIP or NIP toward methyl parathion, chlorpyrifos, or trichlorfon increased as the initial concentrations of the pesticides increasing. However, the MIP adsorbed more pesticides than the NIP, and the adsorption capacities of the MIP toward methyl parathion, chlorpyrifos, and trichlorfon (33.08, 27.55 and 46.32 mg/g) were 1.72-fold higher than that of the NIP (14.97, 16.00 and 17.48 mg/g) at 60 mg/L concentration. This indicated that the MIP could specifically adsorb the three pesticides.
The uptake kinetics of MIP toward methyl parathion, chlorpyrifos, and trichlorfon at 30 mg/L were also examined ((B)). After shaking for 60 min, 82.6% (20.73 mg/g), 68.8% (15.56 mg/g) and 73.9% (18.34 mg/g) of binding were obtained for methyl parathion, chlorpyrifos, and trichlorfon, respectively. The adsorption was almost achieved equilibrium within 120 min. The rapid adsorption kinetics of the Fe3O4@SiO2@MIP is an obvious advantage for its application in BFIA procedure.
Development of BFIA standard curves
To improve the sensitivity of the BFIA method, the assay conditions (the concentrations of QD-haptens, the reaction solution, and the methanol content in the methanol/water mixture) were optimized. The results are presented in Figures S3, S4, and S5. Results showed that when the diluted concentration ratios of methyl parathion, chlorpyrifos, and trichlorfon hapten were 1/100, 1/200 and 1/200, and the methanol/water ration was 3/17 (V/V), higher inhibition rate and less spectra interference were observed.
Under the optimal conditions, the BFIA method was developed using MIP or NIP as the antibody. The fluorescence spectrums of QD-labelled haptens after competing reaction were shown in Figure S6. The standard curves were plotted in . The limits of detection (LOD, IC15) for the method were 0.21 ± 0.021, 0.44 ± 0.069 and 0.32 ± 0.033 μg/L for methyl parathion, chlorpyrifos, and trichlorfon, respectively. The sensitivities (IC50) of the method for these three pesticides were 1.83 ± 0.015, 2.66 ± 0.025, and 2.50 ± 0.039 mg/L. Compared with previous methods (), the results in this study demonstrate a relatively low LOD. Therefore, this method has advantage to be used as a tool for simultaneous determination of trace multiple organophosphorus pesticides.
Figure 4. The BFIA standard curves of three pesticides using the MIP or NIP as antibody at concentrations of 0.000033–33.33 mg/L in methanol/water solution.
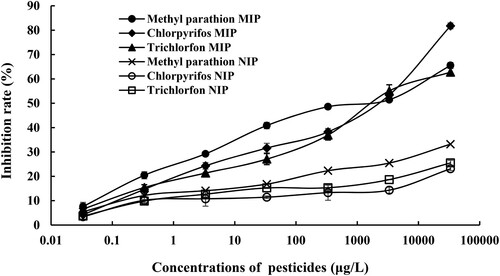
Table 1. Comparison of LOD of this method with other methods for the simultaneous determination of multiple organophosphorus pesticides.
Selectivity of the BFIA assay
To investigate the selectivity of BFIA method, two structural analogs of organophosphorus pesticides (moncrotophos and malathion) and two non-organophosphorus pesticides (atrazine and cyhalothrin) were applied to the BFIA procedure, and the competitive curves were then measured under optimized conditions (). Higher inhibition rate was obtained when the three pesticide haptens competed with the corresponding pesticides, while all of the inhibition rates for the structural analogs of moncrotophos and malathion and two non-organophosphorus pesticides were relatively lower. It was indicated that the BFIA method has a higher selectivity for organophosphorus pesticides. The results in also showed that the MIP as antibody had a higher inhibition rate for the three pesticides than the NIP. This further indicated that the MIP has advantage to be used as a recognition element in the BFIA method. Above results proved that the BFIA assay has good selectivity for the simultaneous determination of multiple organophosphorus pesticides.
Accuracy evaluation of the BFIA method
The accuracy and applicability of the developed BFIA method were also evaluated. The apple and orange samples spiked with methyl parathion, chlorpyrifos, and trichlorfon at levels of 0.5, 2.5 and 5 mg/L were analysed by this method (). Good recoveries between 79.1% and 119.3% were obtained.
Table 2. The recoveries for spiked pesticides in actual samples determination by the BFIA method.
Finally, the level of methyl parathion, chlorpyrifos, and trichlorfon in pear, carrot, kiwifruit, and banana samples were analysed by BFIA, and the results were compared to GC data. As seen in Table S1, there was no significant differences between the two methods (P > 0.05), which proved that the BFIA method has good accuracy and could be used for the simultaneous determination of multiple organophosphorus pesticide residues in agricultural products.
Conclusions
We used Fe3O4@SiO2@MIP as a biomimetic antibody and QDs as labels to develop a BFIA method that can simultaneously determinate methyl parathion, chlorpyrifos, and trichlorfon. With good sensitivity and accuracy, this study provides a tool for the simultaneous determination of multiple organophosphorus pesticide residues in agricultural products.
Supplemental Material
Download ()Disclosure statement
No potential conflict of interest was reported by the authors.
Additional information
Funding
References
- Cheng, Z., Dong, F., Xu, J., Liu, X., Wu, X., Chen, Z., … Zheng, Y. Q. (2017). Simultaneous determination of organophosphorus pesticides in fruits and vegetables using atmospheric pressure gas chromatography quadrupole-time-of-flight mass spectrometry. Food Chemistry, 231, 365–373.
- Fan, J. P., Xu, X. K., Xu, R., Zhang, X. H., & Zhu, J. H. (2015). Preparation and characterization of molecular imprinted polymer functionalized with core/shell magnetic particles (Fe3O4@SiO2@MIP) for the simultaneous recognition and enrichment of four taxoids in Taxus × media. Chemical Engineering Journal, 279, 567–577.
- Farajzadeh, M. A., Mogaddam, M. R. A., Aghdam, S. R., Nouri, N., & Bamorrowat, M. (2016). Application of elevated temperature-dispersive liquid-liquid microextraction for determination of organophosphorus pesticides residues in aqueous samples followed by gas chromatography-flame ionization detection. Food Chemistry, 212, 198–204.
- Fernandes, V. C., Subramanian, V., Mateus, N., Domingues, V. F., & Deleruematos, C. (2012). The development and optimization of a modified single-drop microextraction method for organochlorine pesticides determination by gas chromatography-tandem mass spectrometry. Microchimica Acta, 178(1-2), 195–202.
- Harshit, D., Charmy, K., & Nrupesh, P. (2017). Organophosphorus pesticides determination by novel HPLC and spectrophotometric method. Food Chemistry, 230, 448–453.
- He, Y., Lu, H., Su, Y., Sai, L., Hu, M., Fan, C., & Wang, L. (2011a). Ultra-photostable, non-cytotoxic, and highly fluorescent quantum nanospheres for long-term, high-specificity cell imaging. Biomaterials, 32(8), 2133–2140.
- He, J., & Ming-Tao, F. (2012). Multi-analyte immunoassay for pesticides: A review. Analytical Letters, 45(11), 1347–1364.
- He, Y., Zhong, Y., Peng, F., Wei, X., Su, Y., Lu, Y., … Lee, S. T. (2011b). One-pot microwave synthesis of water-dispersible, ultraphoto- and ph-stable, and highly fluorescent silicon quantum dots. Journal of the American Chemical Society, 133(36), 14192–14195.
- Hou, J. Y., Dong, G. J., Tian, Z. B., Lu, J. T., Wang, Q. Q., Ai, S. Y., & Wang, M. L. (2016). A sensitive fluorescent sensor for selective determination of dichlorvos based on the recovered fluorescence of carbon dots-Cu(II) system. Food Chemistry, 202, 81–87.
- Hu, T. T., Lu, C. M., Li, H., Zhang, Z. X., Zhao, Y. H., & Li, J. (2017). Determination of eleven organophosphorus pesticide residues in textiles by using HPLC-HRMS. Analytical Sciences the International Journal of the Japan Society for Analytical Chemistry, 33(9), 1027.
- Hu, Y., Shen, G., Zhu, H., & Jiang, G. (2010). A class-specific enzyme-linked immunosorbent assay based on magnetic particles for multiresidue organophosphorus pesticides. Journal of Agricultural and Food Chemistry, 58(5), 2801–2806.
- Hu, G., Sheng, W., Zhang, Y., Wang, J., Wu, X., & Wang, S. (2016). Upconversion nanoparticles and monodispersed magnetic polystyrene microsphere based fluorescence immunoassay for the detection of sulfaquinoxaline in animal-derived foods. Journal of Agricultural and Food Chemistry, 64(19), 3908–3915.
- Jiang, M. D., Wu, S., Xu, L. H., Qiao, X. G., & Xu, Z. X. (2017). Determination of trichlorfon residues in vegetables using a quantum dot-labeled biomimetic immunoassay method followed by capillary electrophoresis. Food and Agricultural Immunology, 28(6), 1242–1255.
- Kolberg, D. I., Prestes, O. D., Adaime, M. B., & Zanella, R. (2011). Development of a fast multiresidue method for the determination of pesticides in dry samples (wheat grains, flour and bran) using QuEChERS based method and GC-MS. Food Chemistry, 125(4), 1436–1442.
- Kou, T. T., Kun-Long, X. U., Zhang, L. Z., Yang, J., Wang, D. L., & Chen, Y. F. (2010). GC with flame photometric detection for the simultaneous determination of organophosphorus pesticide multiple residues in pu-erh tea. Food Science, 31(12), 165–168.
- Liang, D. Q., Ken-Ming, S. U., Zhang, J. M., & Guo, W. P. (2014). Determination of 23 organophosphorus pesticide residues in lentinus edodes by high performance liquid chromatography-tandem mass spectrometry. Food Science, 35(4), 159–162.
- Liang, X., Xie, R., Wang, C. M., Gui, W. J., & Zhu, G. N. (2013). Development of a broad-selective immunoassay for multi-residue determination of type ii pyrethroids in west lake water. Food and Agricultural Immunology, 24(1), 59–78.
- Liu, H., Fang, G., & Wang, S. (2014). Molecularly imprinted optosensing material based on hydrophobic CdSe quantum dots via a reverse microemulsion for specific recognition of ractopamine. Biosensors & Bioelectronics, 55(10), 127–132.
- Liu, B., Ge, Y., Zhang, Y., Song, Y., Lv, Y. Y., Wang, X. X., & Wang, S. (2012a). Production of the class-specific antibody and development of direct competitive ELISA for multi-residue detection of organophosphorus pesticides. Food and Agricultural Immunology, 23(2), 157–168.
- Liu, Y. H., Xie, R., Guo, Y. R., Zhu, G. N., & Tang, F. B. (2012b). Comparison of homologous and heterologous formats in nanocolloidal gold-based immunoassays for parathion residue determination. Journal of Environmental Science & Health Part B, 47(5), 475–483.
- Pan, L. L., Sun, J. T., Li, Z. H., Yu, Z., Shen, X., & Zhu, L. Z. (2016). Organophosphate pesticide in agricultural soils from the Yangtze River Delta of China: Concentration, distribution, and risk assessment. Environmental Science & Pollution Research International, 25(1), 1–8.
- Ronald Erney, D. (2015). Determination of organophosphorus pesticides in whole/chocolate/skim-milk and infant formula using solid-phase extraction with capillary gas chromatography/flame photometric detection. Journal of Separation Science, 18(1), 59–62.
- Song, Y., Zhang, Y., Wang, S., & Wang, J. (2016). Determination of metolcarb residues by a biotin-streptavidin-amplified enzyme-linked immunosorbent assay in vegetables and edible fungus. Food & Agricultural Immunology, 27(2), 194–204.
- Taghani, A., Goudarzi, N., Bagherian, G., Chamjangali, M. A., & Amin, A. H. (2018). Application of nano-perlite as a new natural sorbent in preconcentration of three organophosphorus pesticides by microextraction in packed syringe coupled with gc-ms. Journal of Separation Science, 41(10), 2245.
- Tang, Y., Fang, G., Wang, S., Sun, J., & Qian, K. (2013). Rapid determination of metolcarb residues in foods using a biomimetic enzyme-linked immunosorbent assay employing a novel molecularly imprinted polymer film as artificial antibody. Journal of Aoac International, 96(2), 4553–4458.
- Tanhaei, B., Ayati, A., Lahtinen, M., & Sillanpää, M. (2015). Preparation and characterization of a novel chitosan/Al2O3/magnetite nanoparticles composite adsorbent for kinetic, thermodynamic and isotherm studies of methyl orange adsorption. Chemical Engineering Journal, 259, 1–10.
- Tao, L., Yong, X., Zhu, L., & Lei, Z. (2016). Rapid and sensitive detection of 3-amino-2-oxazolidinone using a quantum dot-based immunochromatographic fluorescent biosensor. Journal of Agricultural & Food Chemistry, 64(45), 8678–8683.
- Thanhdong, N., Yu, J. E., Daemyung, L., & Gaeho, L. (2008). A multiresidue method for the determination of 107 pesticides in cabbage and radish using QuEChERs sample preparation method and gas chromatography mass spectrometry. Food Chemistry, 110(1), 207–213.
- Wang, H., Li, G. H., Chen, T., Xue, X. P., & Zhang, Y. (2015). Immunoassay for multiple residues of organophosphorus pesticides using broad spectrum of monoclonal antibodies. Journal of Food Safety & Quality, 165(4), 163–199.
- Wang, C., Li, X., & Liu, Y. (2010). Development of a mab-based heterologous immunoassay for the broad-selective determination of organophosphorus pesticides. Journal of Agricultural and Food Chemistry, 58(9), 5658–5663.
- Wang, G., Ma, Z., Yu, T., & He, F. (2000). Synthesis and identification of methylparathion artificial antigen. Journal of Hygiene Research, 29(2), 69–70.
- Yang, L. X., Li, H. I., Zeng, F. G., Liu, Y. P., Li, R. F., Chen, H. J., … Wu, Y. N. (2012). Determination of 49 organophosphorus pesticide residues and their metabolites in fish, egg, and milk by dual gas chromatography–dual pulse flame photometric detection with gel permeation chromatography cleanup. Journal of Agricultural & Food Chemistry, 60(8), 1906.
- Zhang, C., Han, Y., Li, L., Deng, N., Bo, C., & Yuan, L. (2017). Development of quantum dots-labeled antibody fluorescence immunoassays for the detection of morphine. Journal of Agricultural & Food Chemistry, 65(6), 1290–1295.
- Zheng, C. H., Yang, L. G., Yao, Z. Y., Zhao, D. Y., & Yang, M. M. (2009). Application of electrospunpolymer nanofibers in the determination of five organophosphorus pesticides by HPLC. Journal of Instrumental Analysis, 28(8), 926–930.
- Zhu, F., Ruan, W. H., He, M. H., Zeng, F., Luan, T. G., Tong, Y. X., … OuYang, G. F. (2009). Application of solid-phase microextraction for the determination of organophosphorus pesticides in textiles by gas chromatography with mass spectrometry. Analytica Chimica Acta, 650(2), 202–206.