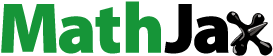
ABSTRACT
The flunixin residues in foods of animal origin could pose hazards for human health. In this paper, a broad-selectivity polyclonal antibody was obtained using 5-hydroxyflunixin coupled with bovine serum albumin as the immunogen. The semi-inhibitory concentration (IC50) of the polyclonal antibody was 1.43 ng/mL for flunixin and 0.29 ng/mL for 5-hydroxyflunixin, respectively, which were far below the maximum residue limits set by the United States, the European Union and China. The limits of detection were calculated to be 2.98 μg/kg for flunixin in bovine muscle and 0.78 μg/L for 5-hydroxyflunixin in milk. In spike and recovery tests, the average recovery ranged from 83% to 105%, with a satisfying coefficient of variance ranging from 5.8% to 11.3%. Furthermore, the ELISA method was validated by a well-established LC-MS/MS method. These results proved that the established method is suitable for flunixin and 5-hydroxyflunixin residue analysis.
Introduction
Flunixin, (2-[[2-methyl-3-(trifluoromethyl)phenyl]amino]-3-pyridinecarboxylic acid, ), a nonsteroidal anti-inflammatory drug (NSAID), is widely used for the treatment of some diseases in animals, such as acute inflammation, mastitis, metritis, lack of lactation, musculoskeletal disorders, abdominal pain and sow endometritis because of its excellent analgesic and antipyretic effects (Anderson, Smith, Shanks, Davis, & Gustafsson, Citation1986; Cook, Meyer, Campbell, & Blikslager, Citation2009; Levionnois, Fosse, & Ranheim, Citation2018). It can inhibit cyclooxygenase synthesis and reduce inflammation and pain within 15 min of drug treatment (Huber, Arnholdt, Möstl, Gelfert, & Drillich, Citation2013; Yazar, Er, Uney, Altunok, & Elmas, Citation2007). The analgesic and antipyretic effects usually occur 1–2 h after treatment, and the maximum effect is maintained for between 2 and 16 h (Aké-López, Segura-Correa, & Quintal-Franco, Citation2005; Levionnois et al., Citation2018). Since October 2004, flunixin has been approved by the Food and Drug Administration for lactation and has been widely used in the United States, France, Switzerland, Germany and the United Kingdom (Shelver, Schneider, & Smith, Citation2016). In addition, flunixin has been approved by the Chinese Ministry of Agriculture for veterinary use in animal husbandry since 2007.
Flunixin is easily metabolised and eliminated in vivo, and approximately 90% of the original drug is excreted via urine and feces 24 h after drug treatment (Levionnois et al., Citation2018). The main metabolites of flunixin are 4-hydroxyflunixin, 5-hydroxyflunixin and 2-methylhydroxyl (Kissell et al., Citation2012; Lin et al., Citation2018). Among these compounds, 5-hydroxyflunixin () is identified as the marker residue in bovine milk, while flunixin is identified in bovine muscle (Jedziniak et al., Citation2013; Ngoh et al., Citation2003; Smith et al., Citation2015).
During the last two decades, many studies proved that flunixin and other NSAID residues in foods of animal origin could pose potential risks to human health such as gastrointestinal damage, hematopoietic and renal systemic toxicity, hepatotoxicity, and aseptic meningitis (Kissell, Baynes, Riviere, & Smith, Citation2013; Schulz, Anderson, Coetzee, White, & Miesner, Citation2011). To reduce these toxic effects, the European Union, the United States, Canada, Japan and China identified maximum residue limits (MRLs) or tolerances for flunixin and 5-hydroxyflunixin () in the range of 6 μg/kg–60 μg/kg in animal tissues and milk (Zhu et al., Citation2013).
Table 1. The MRLs set by different countries for flunixin residues in foods of animal origin.
To prevent potential hazards to human health, various analytical techniques have been used to detect flunixin and other NSAID residues, i.e. high-performance liquid chromatography coupled with UV and mass spectrometry detectors (Asea, Patterson, Korsrud, Dowling, & Boison, Citation2001; Jedziniak, Szprengier-Juszkiewicz, & Olejnik, Citation2009; Liu et al., Citation2015; Peng et al., Citation2013; van Pamel & Daeseleire, Citation2015). Although these methods are very accurate and sensitive, they are costly and require complex sample pretreatment steps and trained personnel (Mukunzi, Suryoprabowo, Song, Liu, & Kuang, Citation2018; Qu et al., Citation2019; Wang et al., Citation2018; Zeng, Jiang, Liu, Song, & Kuang, Citation2018). Immunoassays are superior to chromatographic methods in screening tests for their simplicity, sensitivity, and high-throughput capabilities (Chen et al., Citation2017; Jiang et al., Citation2017; Kong et al., Citation2017; Wang, Luo, Chen, Huang, & Jiang, Citation2016). Recently, some immunoassays for detecting residues of flunixin and other NSAIDs have been reported (Lin et al., Citation2018). However, to the best of our knowledge, enzyme-linked immunosorbent assay (ELISA) methods for simultaneously detecting flunixin and 5-hydroxyflunixin residues have not been reported.
In this paper, we want to establish a rapid screening method for simultaneously detecting the residues of the parent drug and its hydroxyl metabolites (marker residues). In this study, flunixin was coupled with a carrier protein, and polyclonal antibodies were obtained after immunisation of rabbits with synthesised immunogens. Based on the produced antibody, we established an indirect competitive ELISA (icELISA) for the simultaneous detection of flunixin and 5-hydroxyflunixin residues. In addition, to establish a rapid and simple screening method, efforts were made to simplify time-consuming sample preparation. This established icELISA method was validated by the liquid chromatography tandem mass spectrometry (LC-MS/MS) method, and the results indicate that this icELISA method is suitable for the detection of flunixin and 5-hydroxyflunixin in bovine muscle and milk.
Material and methods
Chemicals
Flunixin (99%) was purchased from Dr. Ehrenstorfer GmbH (Augsburg, Germany). 5-hydroxyflunixin (98%) was purchased from Witega Laboratorien Berlin-Adlershof GmbH (Berlin, Germany). Bovine serum albumin (BSA), ovalbumin (OVA), N-hydroxysuccinimide (NHS), 1-ethyl-3-(3-dimethylaminopropyl) carbodiimide (EDC), Freund’s complete and incomplete adjuvant, horseradish peroxidase, and 3,3′,5,5′-tetramethyl-benzidine (TMB) substrate were obtained from Sigma-Aldrich (St. Louis, MO, USA). Horseradish peroxidase-conjugated goat-anti-rabbit IgG was obtained from Jackson ImmunoResearch Laboratories, Inc. (West Grove, PA, USA). In addition, 96-well polystyrene microtiter plates were obtained from Costar Inc. (Milpitas, CA, USA).
Apparatus
A multimode microplate reader was obtained from BioTek Instruments Inc. (Winooski, VT, USA). A 30A ultrahigh-performance liquid chromatography system (Shimadzu, Kyoto, Japan) and an API 4000+ tandem mass spectrometer (AB SCIEX, USA) were used for final validation.
Antigen preparation
Because flunixin and 5-hydroxyflunixin are haptens, the immunogen was prepared by conjugating flunixin and 5-hydroxyflunixin with BSA through the carbodiimide method according to the methods in our previous studies (Jiang et al., Citation2015). Briefly, flunixin (11 mg) or 5-hydroxyflunixin (11 mg) was dissolved in 800 μL of N,N-dimethylformamide, and then NHS (8 mg) and EDC (13 mg) were added to the mixture. Afterwards, the reaction mixture was added to a BSA solution (10 mg dissolved in 4 mL of borate buffer) and reacted at room temperature for another 12 h. The hapten-protein conjugates were then dialysed against 0.01 M phosphate-buffered saline (PBS) for 3 days at 4°C. Similarly, the coating antigen was prepared using this method, and the only difference is that the coating antigen was conjugated with OVA.
Polyclonal antibody production
New Zealand white rabbits were immunised at a dosage of 1.0 mg/kg body weight and with the same volume of Freund’s complete adjuvant. Every two weeks, the rabbits were administered boost immunizations with the same dosage of immunogen emulsified in Freund’s incomplete adjuvant. The blood was collected by ear vein after each injection by subcutaneous multipoint injection, and then the antibody titre was determined by indirect ELISA. The antiserum was prepared by centrifugation of the whole blood, and the polyclonal antibody was purified from antiserum by chromatography.
Optimisation of the ELISA method
The icELISA approach can be described as follows: 96-well microtiter plates were coated with the coating antigen (100 μL/well) at a dilution of 1:2000 in PBS, and then the plates were incubated at 4°C overnight. Then, the plates were coated with 3% skim milk for 1 h at 25°C. After blocking, 50 μL of standard solutions and 50 μL of diluted polyclonal antibody solutions were added to each well, and the plates were incubated for 30 min. Then, diluted horseradish peroxidase-conjugated goat-anti-rabbit IgG was added to each well, and the plates were incubated for 30 min at 25°C. After another three washes, 100 μL of TMB substrate was pipetted, and the absorbance was measured after 50 μL of 2 M H2SO4 was added to stop the enzyme reaction.
Curve fitting, sensitivity and selectivity determination
Standards were run in triplicate wells, and average absorbance values of the standards were plotted against the analyte concentration on a logarithmic scale. Competition curves were fitted to a four parameter logistic equation from which IC50 values were calculated (He et al., Citation2017; Jiang et al., Citation2013).Where A = the absorbance at the high asymptote of the curve, B = the slope at the inflection point of the sigmoid, C = the concentration of analyte resulting in 50% inhibition of tracer binding, D = the absorbance at the low asymptote of the curve and X = the calibration concentration.
To determine the selectivity, a cross-reactivity study was carried out under optimum conditions. The cross-reactivity of the antibody against flunixin, 5-hybroxyflunixin and other cross-reactants was calculated using the IC50 values in the unit of pmol/mL according to the following equation (Jiang et al., Citation2016):
Real sample analysis
Bovine muscle and milk samples were purchased from local supermarkets and stored in a refrigerator (−20°C) until used. The flunixin and 5-hydroxyflunixin concentrations of each sample were quantified by LC-MS/MS before spike and recovery tests (General Administration of Quality Supervision, Citation2008). For sample extraction, the samples were thawed, and 1.0 ± 0.01 g of bovine muscle sample or 1.0 ± 0.01 mL of milk was combined with 4.0 mL of 0.5% hydrochloric acid (HCl) in acetonitrile. Samples were sonicated for 5 min and then centrifuged for 10 min at 5000 g. Then, 2 mL of the supernatant was collected and evaporated to dryness under a stream of nitrogen at 55°C. After reconstitution in 500 μL of assay buffer, the mixture was tested by the established icELISA.
Assay validation
A validation of the icELISA method was carried out according to the related content of the Commission Decision 2002/657/EC (European Commission, Citation2002). The limit of detection (LOD) was based on the average value of 20 blank samples plus three times the standard deviation (SD). The accuracy and precision of the method were represented by the recovery and coefficient of variation (CV), respectively. Concentrations of the tested compounds in naturally contaminated or spiked samples were confirmed by LC-MS/MS and then detected with the established ELISA for the final validation.
Results and discussion
Antigen synthesis and characterisation of the polyclonal antibody
The aim of this paper was to develop immunoassays for the rapid detection of flunixin and 5-hydroxyflunixin residues in foods of animal origin. The design of immunogens is critical for the success of rapid immunoassays. Flunixin and 5-hydroxyflunixin are small molecule haptens without immunogenicity (molecule weight <1000 Da), and they need to be coupled to carrier proteins to elicit a specific immune response in animals. The carboxyl, imido and hydroxyl groups in the molecular structure of flunixin and 5-hydroxyflunixin could be used for the synthesis of immunogens. The successful coupling of hapten to carrier protein was determined by matrix-assisted laser desorption/ionization time of flight mass spectrometry according to our previous studies, and the results showed qualitative differences between the carrier protein and the conjugate, which indicated the successful attachment of the hapten to the carrier protein.
The icELISA described in this paper was used to characterise the performance of the polyclonal antibody. In this study, rabbits immunised with flunixin-BSA and 5-hydroxyfluinxin-BSA produced antibodies. However, the antibody from rabbits immunised with 5-hydroxyfluinxin-BSA had better performance and also recognised flunixin. The synthesised coating antigens and the produced antibody were characterised by homologous and heterologous formats to select their best combinations, and the flunixin-OVA was selected as the coating antigen. When the dilution of antiserum was 1:2000 (after the third immunisation), the absorbance at 450 nm ranged between 1.5 and 2.0. Furthermore, the inhibition rate was > 50% when using 10 ng/mL flunixin as a standard. All of these characteristics indicated polyclonal antibodies would be suitable for detecting target analytes.
Optimisation of the icELISA
Suitable assay conditions are important for improving the sensitivity of icELISA. For this purpose, several key factors affecting the assay performance were studied as follows.
Optimisation of working concentrations of antibody and antigen: Competition experiments were carried out by checkerboard titration gradient dilution to determine the suitable concentrations of coating antigen and antibody. As shown in , the most suitable dilution ratio for coating antigen and antibody was 1:2000 and 1:2000 when a compromise was made between the Amax value (absorbance of assay buffer, ranging from 1.5 to 2.0) and the highest sensitivity (the absorbance at 10 ng/mL flunixin/absorbance of assay buffer).
Table 2. Optimisation of dilutions for coating antigen and antibody using checkerboard titration. The data shown in the table are absorbance values.
pH effect: Unsuitable pH conditions affect the binding between the antigen and antibody. To determine the optimum pH of the assay buffer, calibration curves were prepared in PBS buffers with different pH values between 5.0 and 9.0. Considering the Amax value and the sensitivity of the assay (IC50), a slightly acidic pH (pH 6.5) is preferred for assay buffer ().
Figure 2. Effects of pH (A), ionic strength (B) of assay buffer, and acetonitrile tolerance (C) on the assay performance of icELISA.
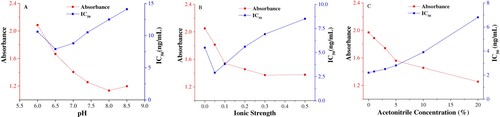
Ionic strength effect: Previous studies indicated that increasing the NaCl concentration improves the sensitivity by weakening the nonspecific binding of the antibody. To evaluate the effect of ionic strength, immunoassay performance was investigated in PBS with NaCl concentrations ranging from 0 to 0.5 M. As shown in , a NaCl concentration of 0.05 M was optimal when compromising between the Amax value and sensitivity. (IC50 exhibited a minimum value, and at the same time, the Amax/IC50 showed the maximum value).
Organic solvent effect: In this paper, acetonitrile was used to extract the target analytes from food matrices, and thus, the acetonitrile residues in the sample extract may affect the assay performance. Calibration curves were performed with PBS buffer containing different proportions of acetonitrile. As shown in , the assay sensitivity and absorbance decreased with increasing acetonitrile concentration. After the assay buffer with different acetonitrile amounts was tested, 0% acetonitrile matched the sample extract calibration curve and was used in the established ELISA.
Curve fitting and sensitivity and selectivity determination
In this study, an icELISA method was established, and the standard solutions were 5-hydroxyflunixin diluted in PBS at concentrations of 0.03–24.3 ng/mL. Whether the established method could be applied practically depends on its repeatability. Herein, repeatability was investigated by five tests at every analyte concentration. The results showed that the CVs of absorbance at six different concentrations levels were in the range of 3.5%-6.8%, which indicated a good repeatability of icELISA.
To assess sensitivity and selectivity, IC50 and cross-reactivity (CR%) values were determined by the established icELISA. A concentration series of standard solutions was prepared and measured in the optimised assay conditions. The standard calibration curves are shown in , and the IC50 value was calculated to be 1.43 ng/mL for flunixin and 0.29 ng/mL for 5-hydroxyflunixin. The quantification linear range was as broad as 0.13–68.7 ng/mL for flunixin, whereas the linear working range was determined to range from 0.076 ng/mL to 16.3 ng/mL for 5-hydroxyflunixin. The IC50 values obtained in this work were much lower than those in some previous reports, and the results indicated that the icELISA fabricated in this work displayed better performance.
Figure 3. Calibration curves of icELISA for flunixin and 5-hydroxyflunixin residue analysis. The data are average values of triplicate samples (average ± SD).
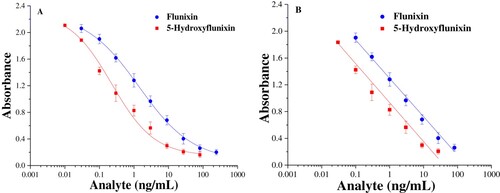
The selectivity of the icELISA was assessed by the cross-reactivity against the analogs compared with flunixin, including 5-hydroxyflunixin, and other related NSAID analytes under the same experimental conditions. gives the IC50 values of other structurally related analytes and their cross-reactivity values. When the cross-reactivity with 5-hydroxyflunixin was set as 100%, the cross-reactivity with flunixin was calculated to be 20.3%. However, there was a small amount of cross-reactivity (< 0.1%) with the other related NSAID compounds because they are not structurally similar to the target haptens. The results suggested that these analogs should not interfere with flunixin and 5-hydroxyflunixin detection.
Table 3. IC50 and cross-reactivity values using 5-hydroxyflunixin as a standard.
Real sample analysis
In published analytical methods, flunixin and 5-hydroxyflunixin residues have mostly been determined by the use of LC-MS/MS. In the sample pretreatment procedures, acid or enzyme hydrolysis is a critical part for the release of tissue-bound residues into solution because of the high (99%) protein binding of flunixin (Horii, Ikenaga, Shimoda, & Kokue, Citation2004). In previous studies (Lin et al., Citation2018), the samples were directly diluted or extracted for the analysis of flunixin residues in foods of animal origin. This method may be used for satisfactorily detecting artificially spiked samples; however, high protein binding increases the potential risk of false negative rates. In this paper, different approaches in the extraction step were applied. First, the samples were extracted and then diluted by assay buffer for analysis. As shown in A and B, the calibration curves built directly from bovine muscle and milk extracts did not match the calibration curves built in assay buffer. Considering the high protein binding, the spiked bovine muscle and milk samples were acidified with HCl for the precipitation of proteins, and the supernatant was extracted with acetonitrile in the presence of sodium chloride to simultaneously extract flunixin and its main metabolite 5-hydroxyflunixin from bovine muscle and milk. The extraction efficiency was investigated using the incurred sample containing flunixin and 5-hydroxyflunixin that had been taken 12 h after injection. For this purpose, four samples were taken, and three of them were acidified with 200 µL of 1 N, 3 N, and 6 N HCl. The samples were left to stand for 12 h and then analyzed according to the procedure. The results from acidified samples were compared to the results from a blank sample extract spiked with the target analytes, whose value was regarded as 100%. As presented in C, the recovery values meet the requirements of spike and recovery tests when the samples were acidified using 3N HCl in the sample extract procedures.
Assay validation
In this paper, LOD, accuracy, repeatability and reproducibility were investigated for assay validation. Based on the average values determined from blank samples plus three times the standard deviations, the LODs were calculated to be 2.98 μg/kg for flunixin in bovine muscle and 0.78 μg/L for 5-hydroxyflunixin in milk samples, which were lower than the MRLs set by the European Commission, United States and China. A spike and recovery test was carried out to assess the accuracy, repeatability and within-laboratory reproducibility. The blank samples were spiked at three concentration levels (5, 10, and 20 μg/kg for flunixin and 2, 5, and 10 μg/L for 5-hydroxyflunixin). After the sample pretreatments, the extract was tested by the established icELISA. As shown in , the average recoveries were in the range of 83%–94% for flunixin and 81%–105% for 5-hydroxyflunixin, which were within an acceptable range. Repeatability and within-laboratory reproducibility, both expressed as the CVs, were 5.8%–10.2% for flunixin and 6.8%–11.3% for 5-hydroxyflunixin, respectively. The results suggested that the established method was accurate for use in real sample analysis.
Table 4. Average recovery and CV values for flunixin and 5-hydroxyflunixin detection in bovine muscle and milk (n = 3).
To further evaluate the accuracy and precision of the established ELISA, a well-established LC-MS/MS method was used to test the same spiked concentrations of flunixin. As shown in , the naturally contaminated and artificially spiked samples were investigated by icELISA and LC-MS/MS. The results of icELISA showed few significant difference from those of LC-MS/MS, which indicates that icELISA could be applied for target analyte analysis with good effectiveness and applicability.
Figure 5. Correlation between icELISA and LC-MS/MS in detecting flunixin residues in bovine muscle (A) and 5-hydroxyflunixin residues in milk (B). The data are average values of triplicate samples (average ± SD).
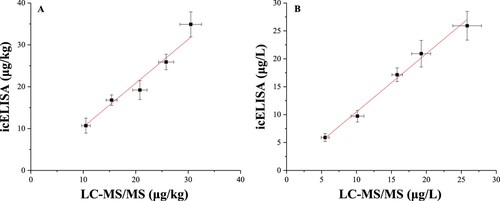
Flunixin has been widely used for disease treatment in animal husbandry because of its excellent analgesic and antipyretic effects. The flunixin and 5-hydroxyflunixin residues in foods of animal origin could pose hazards for human health. In this paper, we developed an icELISA for the rapid screening of flunixin residue in bovine muscle and 5-hydroxyflunixin residue in milk. The produced antibody in this study could simultaneously recognise flunixin (IC50: 1.43 ng/mL) and 5-hydroxyflunixin (IC50: 0.29 ng/mL), and the calculated LODs of the developed icELISA were 2.98 μg/kg for flunixin in bovine muscle and 0.78 μg/L for 5-hydroxyflunixin in milk. In 2018, Lin et al successfully produced a sensitive anti-flunixin meglumine monoclonal antibody 2H4, and the produced antibody had an IC50 of 0.29 ng/mL, a LOD (IC10) of 0.1 ng/mL, and a linear range of 0.08664 ng/mL – 0.97226 ng/mL. Based on the developed antibody and icELISA, Lin et al. developed an effective and portable immunochromatographic strip assay with a cutoff value of 0.29 ng/mL flunixin meglumine in milk (Lin et al., Citation2018). Compared with previous research (Lin et al., Citation2018), we used 5-hydroxyflunixin to synthesise immunogens, and the produced antibody showed a satisfying cross-reactivity (20.3%) with is parent drug flunixin. Based on the produced antibody, we established an icELISA for the simultaneous screening of flunixin residue in bovine muscle and 5-hydroxyflunixin residue in milk before confirmation by a mass spectrometry method.
Conclusions
In summary, an icELISA was established to detect flunixin residue in bovine muscle and 5-hydroxyflunixin residue in milk before confirmation by a mass spectrometry method. Compared with traditional methods, several merits of the proposed assay were highlighted. First, the proposed polyclonal antibody could be used to detect flunixin and its marker residues. In addition, the sample pretreatment was more suitable than traditional methods for analyzing a large number of samples within 120 min. In real-sample tests, the results from the icELISA agreed well with those from the LC-MS/MS method. From a practical point of view, this icELISA method can be carried out in any routine laboratory without the requirement of special facilities.
Disclosure statement
No potential conflict of interest was reported by the authors.
Additional information
Funding
References
- Aké-López, R., Segura-Correa, J. C., & Quintal-Franco, J. (2005). Effect of flunixin meglumine on the corpus luteum and possible prevention of embryonic loss in Pelibuey ewes. Small Ruminant Research, 59(1), 83–87. doi: 10.1016/j.smallrumres.2004.11.008
- Anderson, K., Smith, A., Shanks, R., Davis, L., & Gustafsson, B. (1986). Efficacy of flunixin meglumine for the treatment of endotoxin-induced bovine mastitis. American Journal of Veterinary Research, 47(6), 1366–1372.
- Asea, P. A., Patterson, J. R., Korsrud, G. O., Dowling, P. M., & Boison, J. O. (2001). Determination of flunixin residues in bovine muscle tissue by liquid chromatography with UV detection. Journal of AOAC International, 84(3), 659–665.
- Chen, Y., Liu, L., Xie, Z., Zhu, J., Song, S., & Kuang, H. (2017). Gold immunochromatographic assay for trimethoprim in milk and honey samples based on a heterogenous monoclonal antibody. Food and Agricultural Immunology, 28(6), 1046–1057. doi: 10.1080/09540105.2017.1325843
- Cook, V. L., Meyer, C. T., Campbell, N. B., & Blikslager, A. T. (2009). Effect of firocoxib or flunixin meglumine on recovery of ischemic-injured equine jejunum. American Journal of Veterinary Research, 70(8), 992–1000. doi: 10.2460/ajvr.70.8.992
- European Commission. (2002). Commission Decision 2002/657/EC of 12 August 2002 implementing Council Directive 96/23/EC concerning the performance of analytical methods and the interpretation of results. Official Journal of European Communities, 221, 8–36.
- General Administration of Quality Supervision,Inspection and Quarantine of People’s Republic of China. (2008). Determination of residues of non-steroidal anti-inflammatory drugs in foodstuffs of animal origin for import and export-LC-MS/MS method (SN/T 2190–2008).
- He, J., Wu, N., Luo, P., Guo, P., Qu, J., Zhang, S., … Jiang, W. (2017). Development of a heterologous enzyme-linked immunosorbent assay for the detection of clindamycin and lincomycin residues in edible animal tissues. Meat Science, 125, 137–142. doi: 10.1016/j.meatsci.2016.11.024
- Horii, Y., Ikenaga, M., Shimoda, M., & Kokue, E. (2004). Pharmacokinetics of flunixin in the cat: Enterohepatic circulation and active transport mechanism in the liver. Journal of Veterinary Pharmacology and Therapeutics, 27(2), 65–69. doi: 10.1111/j.1365-2885.2004.00551.x
- Huber, J., Arnholdt, T., Möstl, E., Gelfert, C. C., & Drillich, M. (2013). Pain management with flunixin meglumine at dehorning of calves. Journal of Dairy Science, 96(1), 132–140. doi: 10.3168/jds.2012-5483
- Jedziniak, P., Olejnik, M., Szprengier-Juszkiewicz, T., Smulski, S., Kaczmarowski, M., & Żmudzki, J. (2013). Identification of flunixin glucuronide and depletion of flunixin and its marker residue in bovine milk. Journal of Veterinary Pharmacology and Therapeutics, 36(6), 571–575. doi: 10.1111/jvp.12035
- Jedziniak, P., Szprengier-Juszkiewicz, T., & Olejnik, M. (2009). Multi-residue screening method for the determination of non-steroidal anti-inflammatory drug residues in cow’s milk with HPLC-UV and its application to meloxicam residue depletion study. Bulletin of the Veterinary Institute in Pulawy, 53, 731–739.
- Jiang, W., Beier, R. C., Luo, P., Zhai, P., Wu, N., Lin, G., … Xu, G. (2016). Analysis of Pirlimycin residues in beef muscle, milk, and honey by a biotin–streptavidin-amplified enzyme-linked immunosorbent assay. Journal of Agricultural and Food Chemistry, 64(1), 364–370. doi: 10.1021/acs.jafc.5b05711
- Jiang, W., Beloglazova, N. V., Luo, P., Guo, P., Lin, G., & Wang, X. (2017). A dual-color quantum dots encoded frit-based immunoassay for visual detection of Aflatoxin M1 and Pirlimycin residues in milk. Journal of Agricultural and Food Chemistry, 65(8), 1822–1828. doi: 10.1021/acs.jafc.6b05337
- Jiang, W., Beloglazova, N. V., Wang, Z., Jiang, H., Wen, K., de Saeger, S., … Shen, J. (2015). Development of a multiplex flow-through immunoaffinity chromatography test for the on-site screening of 14 sulfonamide and 13 quinolone residues in milk. Biosensers & Bioelectronics, 66, 124–128. doi: 10.1016/j.bios.2014.11.004
- Jiang, W., Wang, Z., Nölke, G., Zhang, J., Niu, L., & Shen, J. (2013). Simultaneous determination of aflatoxin B1 and aflatoxin M1 in food matrices by enzyme-linked immunosorbent assay. Food Analytical Methods, 6, 767–774. doi: 10.1007/s12161-012-9484-5
- Kissell, L. W., Baynes, R. E., Riviere, J. E., & Smith, G. W. (2013). Occurrence of flunixin residues in bovine milk samples from the USA. Food Additives & Contaminants: Part A, 30(9), 1513–1516. doi: 10.1080/19440049.2013.803604
- Kissell, L. W., Smith, G. W., Leavens, T. L., Baynes, R. E., Wu, H., & Riviere, J. E. (2012). Plasma pharmacokinetics and milk residues of flunixin and 5-hydroxy flunixin following different routes of administration in dairy cattle. Journal of Dairy Science, 95(12), 7151–7157. doi: 10.3168/jds.2012-5754
- Kong, D., Liu, L., Song, S., Zheng, Q., Wu, X., & Kuang, H. (2017). Rapid detection of tenuazonic acid in cereal and fruit juice using a lateral-flow immunochromatographic assay strip. Food and Agricultural Immunology, 28(6), 1293–1303. doi: 10.1080/09540105.2017.1337085
- Levionnois, O. L., Fosse, T. K., & Ranheim, B. (2018). PK/PD modeling of flunixin meglumine in a kaolin-induced inflammation model in piglets. Journal of Veterinary Pharmacology and Therapeutics, 41(2), 314–323. doi: 10.1111/jvp.12468
- Lin, L., Jiang, W., Xu, L., Liu, L., Song, S., & Kuang, H. (2018). Development of IC-ELISA and immunochromatographic strip assay for the detection of flunixin meglumine in milk. Food & Agricultural Immunology, 29(1), 193–203. doi: 10.1080/09540105.2017.1364710
- Liu, Z.-Y., Yang, K., Chen, F.-H., Long, X.-M., Deng, Y.-B., Kuang, G.-W., & Sun, Z.-L. (2015). Development of a rapid method for the confirmatory analysis of flunixin residue in animal tissues using liquid chromatography–tandem mass spectrometry. Food Analytical Methods, 8(2), 352–362. doi: 10.1007/s12161-014-9901-z
- Mukunzi, D., Suryoprabowo, S., Song, S., Liu, L., & Kuang, H. (2018). Development of an indirect enzyme-linked immunosorbent assay and lateral-flow test strips for pefloxacin and its analogues in chicken muscle samples. Food and Agricultural Immunology, 29(1), 484–497. doi: 10.1080/09540105.2017.1406460
- Ngoh, M. A., Wislocki, P. G., Thompson, K., Katz, T., Weingarten, A., TerHune, T., & Hurshman, B. (2003). Residue depletion study and withdrawal period for flunixin-N-methyl glucamine in bovine milk following intravenous administration. Journal of Agricultural and Food Chemistry, 51(16), 4701–4707. doi: 10.1021/jf026219+
- Peng, T., Zhu, A.-L., Zhou, Y.-N., Hu, T., Yue, Z.-F., Chen, D.-D., … Jiang, H.-Y. (2013). Development of a simple method for simultaneous determination of nine subclasses of non-steroidal anti-inflammatory drugs in milk and dairy products by ultra-performance liquid chromatography with tandem mass spectrometry. Journal of Chromatography B, 933, 15–23. doi: 10.1016/j.jchromb.2013.06.025
- Qu, J., Xie, H., Zhang, S., Luo, P., Guo, P., Chen, X., … Jiang, W. (2019). Multiplex flow cytometric immunoassays for high-throughput screening of multiple mycotoxin residues in milk. Food Analytical Methods. doi: 10.1007/s12161-018-01412-4
- Schulz, K. L., Anderson, D. E., Coetzee, J. F., White, B. J., & Miesner, M. D. (2011). Effect of flunixin meglumine on the amelioration of lameness in dairy steers with amphotericin B-induced transient synovitis-arthritis. American Journal of Veterinary Research, 72(11), 1431–1438. doi: 10.2460/ajvr.72.11.1431
- Shelver, W. L., Schneider, M. J., & Smith, D. J. (2016). Distribution of flunixin residues in muscles of dairy cattle dosed with lipopolysaccharide or saline and treated with flunixin by intravenous or intramuscular injection. Journal of Agricultural and Food Chemistry, 64(1), 9697–9701. doi: 10.1021/acs.jafc.6b04792
- Smith, D. J., Shelver, W. L., Baynes, R. E., Tell, L., Gehring, R., Li, M., … Riviere, J. E. (2015). Excretory, secretory, and tissue residues after label and extra-label administration of flunixin meglumine to saline- or lipopolysaccharide-exposed dairy cows. Journal of Agricultural and Food Chemistry, 63(19), 4893–4901. doi: 10.1021/acs.jafc.5b01509
- van Pamel, E., & Daeseleire, E. (2015). A multiresidue liquid chromatographic/tandem mass spectrometric method for the detection and quantitation of 15 nonsteroidal anti-inflammatory drugs (NSAIDs) in bovine meat and milk. Analytical and Bioanalytical Chemistry, 407(15), 4485–4494. doi: 10.1007/s00216-015-8634-1
- Wang, J., Wang, Q., Zheng, Y., Peng, T., Yao, K., Xie, S., … Jiang, H. (2018). Development of a quantitative fluorescence-based lateral flow immunoassay for determination of chloramphenicol, thiamphenicol and florfenicol in milk. Food and Agricultural Immunology, 29(1), 56–66. doi: 10.1080/09540105.2017.1359498
- Wang, X., Luo, P., Chen, J., Huang, Y., & Jiang, W. (2016). Development of a quantitative immuno-affinity test column assay for on-site screening of clindamycin residues in milk. International Dairy Journal, 55, 59–63. doi: 10.1016/j.idairyj.2015.12.002
- Yazar, E., Er, A., Uney, K., Altunok, V., & Elmas, M. (2007). Effect of flunixin meglumine on cytokine levels in experimental endotoxemia in mice. Journal of Veterinary Medicine Series A, 54(7), 352–355. doi: 10.1111/j.1439-0442.2007.00959.x
- Zeng, L., Jiang, W., Liu, L., Song, S., & Kuang, H. (2018). Development of ic-ELISA and lateral-flow immunochromatographic strip for detection of vitamin B2 in an energy drink and vitamin tablets. Food and Agricultural Immunology, 29(1), 121–132. doi: 10.1080/09540105.2017.1360257
- Zhu, A.-L., Peng, T., Liu, L., Xia, X., Hu, T., Tao, X. Q., … Jiang, H. Y. (2013). Ultra-performance liquid chromatography–tandem mass spectrometry determination and depletion profile of flunixin residues in tissues after single oral administration in rabbits. Journal of Chromatography B, 934, 8–15. doi: 10.1016/j.jchromb.2013.07.002