ABSTRACT
Here we demonstrate a noncompetitive idiometric nanobodies phage immunoassay for the determination of FB1, utilizing two types of anti-idiotypic nanobody, that is specific for different epitopes within the hypervariable region of the primary mAb. Three alphatype anti-idiotypic nanobodies were obtained from a naive nanobodies phage-display library. A noncompetitive idiometric immunoassay was established with a combination of betatype anti-idiotypic nanobody and phage-displayed alphatype anti-idiotypic nanobody. The half-maximal saturation of the signal value (SC50) was 0.68 ng/mL, with the limit of detection (LOD) was 0.19 ng/mL. When the noncompetitive assay was compared to the competitive ELISA (LOD = 3.41 ng/mL), an over 17-fold improvement in sensitivity was observed. A correlation (R2) was 0.988 between the data of the noncompetitive assay and LC–MS/MS for the determination of FB1 in cereal samples. The noncompetitive idiometric immunoassay would have a broad utility for monitoring small molecules in food and environment.
1. Introduction
Immunoassays can be classified as either competitive, in which the measured signal is associated with a limited amount of antibodies that failed to capture the analyte; or noncompetitive, in which the measurement of the signal is associated with antibody that binds the analyte (Li, Wu, Song, Zheng, & Kuang, Citation2019; Suryoprabowo et al., Citation2019). Theoretical study has showed that noncompetitive immunoassays are good sensitivity, precision, a wider working range, and comparability with competitive immunoassays (Gujral, Yoo, Bamdad, Suh, & Sunwoo, Citation2017). Thus, researchers have sought to develop noncompetitive immunoassays for the determination of small molecules. Despite these potential advantages, the noncompetitive immunoassay system is unsuitable for measuring small molecules with a single antigenic determinant (epitope) called haptens, such as toxins, vitamins, drugs, eicosanoids, and industrial chemicals (Akter, Vehniäinen, Kankaanpää, & Lamminmäki, Citation2017; Li et al., Citation2018). Several attempts have been made to overcome this difficulty, and several novel immunoassays that can noncompetitively detect haptens have been developed. These methodologies are based on chemical modification to the analytes (Carlomagno et al., Citation2014; Haasnoot & Verheijen, Citation2001), idiometric assays with anti-idiotype antibodies (Niwa et al., Citation2009), the anti-metatype antibody (Omi et al., Citation2015; Tsutsumi, Nagata, Yoshida, Harada, & Ueno, Citation2000), the split recombinant variable region fragments of the antibody (Chung, Makino, Dong, & Ueda, Citation2015) or other principles (Saha, Roy, & Dhar, Citation2013). Although these approaches have been successfully used to measure several haptens, preparing the reagents and these unconventional antibodies is complicated and time consuming. These drawbacks have hindered the popularization of using these methods to detect other haptens.
With the development of phage-displayed technology, more researchers have become interested in establishing the noncompetitive phage anti-immune complex assay (PHAIA) (Dong et al., Citation2014; González-Techera, Vanrell, Last, Hammock, & González-Sapienza, Citation2007). The peptide has been used in these formats. However, peptide with only several amino acids must be used to attach to the phage particles or fusion proteins. Recent success in the generation of nanobodies prompted our interest in developing nanobodies-based immunoassay. The nanobody is the variable domain of a heavy-chain antibody (VHH) derived from the variable region of the heavy-chain antibody existing in camelids (Hamerscasterman, Citation1993). Nanobodies exhibit many unique antibody features, such as a small molecular weight (15 kDa), high expression in various expression levels, thermostability, high solubility, and easy genetic manipulation (del Rio et al., Citation2019; Tu et al., Citation2012). The anti-idiotypic nanobody, with these advantages, has been developed for diagnostic, therapeutic, food safety and environmental immunoassays (Qiu et al., Citation2018). The major difference between the peptide and nanobody is related to the affinities that can be achieved, being the nanobody more complex tridimensional structures that can achieve higher affinity.
So we established a noncompetitive idiometric nanobodies phage immunoassay using two types of anti-idiotypic antibody (Ab2) to explore the possibility of expanding the scope of the noncompetitive immunoassay to small molecules. Ab2 is the second antibody that recognizes different epitopes within the hypervariable region of the primary antibody (Jerne, Citation1974). Alphatype Ab2 (Ab2α) and betatype Ab2 (Ab2β) are the two main types of anti-idiotypic antibodie (Lan et al., Citation2017; Liu et al., Citation2018). Ab2α recognizes an epitope, which is not part of the binding site (paratope) of the primary antibody, involved in antigen recognition. Meanwhile, Ab2β competes with the analyte for an epitope at the paratope. Ab2α will bind to the primary antibody in the presence of or absence the analyte and will not capture onto the Ab2β/primary antibody complex because of steric hindrance, whereas the Ab2β will not bind to the primary antibody in the presence of the analyte.
In this technique, a noncompetitive idiometric nanobodies phage immunoassay system suitable for the measurement of Fuminosin B1 has been generated. The assay was performed using these matched antibodies, namely, the primary antibody and two specific types of anti-idiotypic nanobodies (the fusion protein of the Ab2β nanobody and the phage of the Ab2α nanobody). The noncompetitive idiometric nanobodies phage immunoassay is appropriate for determining small molecules in samples.
2. Materials and methods
2.1. Chemicals and reagents
PfuTurbo Cx Hotstart DNA polymerase was purchased from Agilent Technologies Inc. (Santa Clara, CA). Enzymes and M13KO7 helper phage were purchased from New England Biolabs, Inc. (Beverly, MA). Mycotoxin standards, bovine serum albumin (BSA) and TMB liquid-1 component substate were obtained from Sigma–Aldrich (St. Louis, MO). B-PER bacterial protein extraction reagent, HisPur Ni–NTA resin, and SYPRO Ruby protein gel stain were purchased from Thermo Fisher Scientific Inc. (Waltham, MA). HRP conjugated anti–M13 monoclonal antibody was abtained form GE Healthcare (Piscataway, NJ). Primers A–F (5′–CAT GCC ATG ACT GTG GCC CAG CCG GCC CAG KTG CAG CTC GTG GAG TCN GGN GG), A–R1/R2 (A–R1: 5′–CAT GCC ATG ACT CGC GGC CCC CGA GGC CTC GTG GGG GTC TTC GCT GTG GTG CG; A–R2: 5′–CAT GCC ATG ACT CGC GGC CCC CGA GGC CTG GCC TTG TTT TGG TGT CTT GGG) were synthesized from Integrated DNA Technologies (Division of Biological Sciences, Automated DNA Sequencing Facility, University of California, Davis, CA, USA). The monoclonal antibodies (anti-FB1 mAb 3F11, anti-FB1 mAb 1D11, anti-ZEN mAb, anti-DON mAb) and Ab2β nanobody (B26) were stored in Dr. Yang Xu’s laboratory. The pComb3x phagemid vector was kindly provided by Dr. Carlos Barbas III (Scripps Research Institute, La Jolla, CA).
2.2. Naive nanobodies phage-displayed library construction
Construction of a naive nanobodies phage-displayed library was described as Faraji (Faraji et al., Citation2018). In brief, total RNA was extracted using a total RNA isolation system (Thermo Fisher Scientific, Waltham, MA, USA) from llama peripheral blood lymphocytes. It was used to synthesize the first strand of cDNA and was then amplified using VHH IgG specific primers (Primers A–F and A–R1/R2). The DNA fragments were prepared by the digestion using SfiI and subsequently ligated into the digested pComb3x constructs. It was transfected into electrocompetent ER2738 cells. The library size was titered by plating on Luria Bertani-ampicillin plates.
2.3. Library panning
For the sceening procedure, two wells of a microtiter were immobilized with purified anti-FB1 mAb at 50, 10, 5, and 1 μg per well for rounds 1, 2, 3, and 4, respectively, and were left overnight at 4°C. Four additional microwells coated with 100 μL of 3 mg/mL BSA–PBS were used for pre-absorption and post-absorption. After coating, wells were blocked by incubation with 300 μL of 5 mg/mL BSA–PBS at room temperature for 1 h. After washing five times with PBST (0.01 mol/L PBS pH 7.4 containing 0.05% (v/v) Tween-20), the diluted phage library (2 × 1012 pfu/mL, 100 µL) was dispensed into the pre-absorption well. Meanwhile, the anti-FB1 mAb coated well had been incubated with the concentration of FB1 standard at 0, 10, 25, and 50 ng/mL for rounds 1, 2, 3, and 4, respectively. After incubating for 1 h at room temperature, the unbound phage was added to the anti-FB1 mAb well and incubated as described before. Wells were washed 10 times with PBST, then the bound phage was eluted with 100 μL of 0.1 mol/L glycine–HCl (pH 2.2) for 10 min and neutralized by the addition of 15 μL of 1 mol/L Tris–HCl (pH 8.5). At last, the elution was transferred into the post-absorption plate and incubated at room temperature for 1 h. Individual clones were picked randomly and tested using phage ELISA after four rounds of panning-elution selection.
2.4. Preparation of purified of phage Ab2α suspensions
Phage Ab2α clones were selected and individually amplified according to previously report (Barbas, Burton, Scott, & Silverman, Citation2001). After two times of precipitation with PEG–NaCl (20% (w/v) PEG8000/2.5 mol/L NaCl), the phages were suspended with PBS (0.01M, pH7.4) for storage at 4°C.
2.5. Phage ELISA screening
In particular, the Ab2α that is selected in the assay will not bind to the primary antibody-Ab2β complex due to epitope proximity. In order to choose the Ab2α nanobody, a idiometric phage ELISA that based on the use of three matched antibodies, the primary antibody, Ab2α and Ab2β antibody (B26) was set up according to the principle shown in . The anti-FB1 mAb or BSA (5 µg/mL) in 0.05 M carbonate buffer (pH 9.0, 150 µL) was placed in microtiter wells, and left overnight at 4°C. After washing five times with PBST, the wells were blocked for 1 h at room temperature with 3% (w/v) milk–PBS, then washed three times with PBST. PBS containing a solution of B26 (10 μg/mL, 50 µL) which was expressed using a bacterial system (Shu et al., Citation2015), with FB1 (10 ng/mL, 50 µL) or without, and the culture of individual amplified phage clones (50 µL), were added to the wells and mixed. These mixtures were incubated for 1 h at room temperature. After washing ten times as described above, Horseradish peroxidase (HRP) conjugated anti–M13 antibody (diluted 1:5000 in PBS) (100 μL) was added, and incubated at room temperature for another hour. The bound peroxidase activity was measured colorimetrically using a substrate solution (100 μL/well). After incubating at room temperature for 10 min, the enzymatic reaction was stopped with 2 mol/L H2SO4 (50 μL/well). The absorbance at 450 nm was measured with a microtiter plate reader (Molecular Devices, Sunnyvale, CA, USA).
2.6. A noncompetitive idiometric nanobodies phage ELISA
To select a suitable combination of Ab2α and B26, the concentrations of coating anti-FB1 mAb, B26 and serial dilutions of each phage Ab2α particles in the absence or presence of FB1 were determined in checkerboard experiments. A noncompetitive idiometric nanobodies phage ELISA was set up, which use three matched antibodies, namely, anti-FB1 mAb, phage Ab2α, and B26. The microwells were coated with the anti-FB1 mAb (300 ng) and blocked as described above. After washing five times with PBST (10 mM, pH7.4 PBS + 0.05% Tween 20), the following three types of solution were added and mixed: (1) a series of FB1 standard solutions (or extracted samples) (50 μL), (2) a solution containing B26 (50 μL), and (3) a solution containing the Ab2α phage (50 μL). The wells were incubated for 1 h at room temperature, and washed ten times with PBST. Then a 1:5000 dilution of HRP conjugated anti-M13 antibody was added and incubated at room temperature for another hour. The bound peroxidase activity was measured as described before.
2.7. Cross-reactivity evaluation for the noncompetitive idiometric nanobodies phage ELISA
The cross reactivities of the primary mAb (3F11) for a group of mycotoxins (FB2, FB3, AFB1, OTA, and ZEN) in the range of 0.1–1000 ng/mL and different primary monoclonal antibodies were determined using the noncompetitive idiometric nanobodies phage ELISA. The cross-reactivity (%CR) was calculated: SC50 (FB1)/SC50 (cross-reacting compound) × 100%.
2.8. Sample analysis and validation
The specificity of the noncompetitive immunoassay performance was evaluated by spiking 10–1000 μg/kg concentrations of FB1 to corn blank sample extraction. Incurred samples were prepared as our previous report (Shu et al., Citation2016). The supernatant was loaded onto the strong anion exchange–solid phase extraction (SPE) columns (Agilent Technologies, Palo Alto, CA, USA) preconditioned with 5 mL of 75% methanol in water (v/v). Up to 2 mL of the sample solution (acetic acid/methanol, 10:90, v/v) was added to the column and evaporated to dryness under a stream of nitrogen. Thereafter, the dry residue was dissolved in 2 mL of 5% methanol, 10 mM PBS (pH 7.4) for the noncompetitive idiometric nanobodies phage ELISA and LC–MS/MS analysis.
3. Result and discussion
3.1. Panning and sequence alignment of Ab2α nanobody
A naive nanobodies phage-displayed library with an estimated size of 4.2 × 108 Colony-Forming Units (CFU) was constructed on the pComb3X vector. In order to obtain strong Ab2α phage clones, four cycles of panning were conducted with increasing concentrations of the FB1 standard for binding in each round. In addition, vigorous washing steps and pre/post-absorption were used to minimize no or infirm binding. During four rounds of biopanning, the eluted phages were increased from 1.5 × 105–4.6 × 108 /mL phage particles (data not shown), indicating that phages were enriched during each panning. Using a idiometric phage ELISA, up to 90 clones were selected from the third and fourth round and screened for binding to the coating anti-FB1 mAb in the presence or absence of FB1. Fifteen out of 90 clones showed negligible binding to the anti-FB1 mAb in the absence of FB1, although they binded to the coating antibody in the existence of 50 ng/mL FB1 ((a)).
Figure 2. (a) Phage ELISA results with several single clones towards 3F11 with 50 ng/mL FB1 (red column), without FB1 (black column) or BSA (slash column). (b) Noncompetitive idiometric nanobodies phage ELISA for FB1 set up with different phage Ab2α. Data are reported as an average ± standard error.
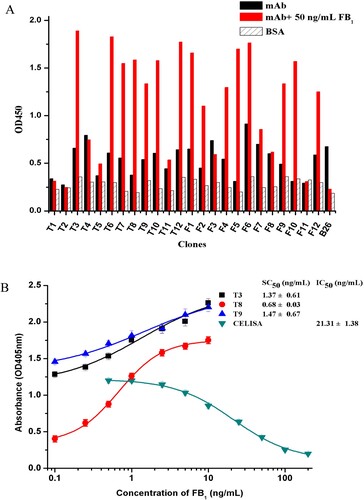
The plasmids of the positive clones were isolated and sequenced. The amino acid sequences were aligned using the software of BioEdit (Carlsbad Biosciences, CA, USA). Analysis of the nanobody sequences revealed three unique sequences were obtained. Among them, T8 contains the possession of amino acid residue F(Phe), E(Glu), R(Arg), and F in the FR2 and 21 amino acids in CDR3 regions, which are the primary features of heavy-chain antibodies. T3 and T9 possess G and P instead of E and F in FR2 and a relatively short CDR3 region (13 amino acids) (Figure S1).
3.2. Ab2α and Ab2β nanobodies based noncompetitive assay
To select a suitable combination of Ab2α and Ab2β, three dilutions of phage particles (3 × 1011, 3 × 1010, 3 × 109 pfu/mL) were added to the wells of the plate coated with four different concentrations of coating anti-FB1 mAb (10, 5, 2.5, and 1.25 µg/mL), Ab2β nanobody (20, 10, 5, 0 µg/mL) in the absence or presence of FB1 (0, 0.1, 0.5, 1, 5,10, 20 ng/mL). Figure S2 shows the result with clone T8. Similar results were evaluated for the other two clones (data not shown). The maximal intensity of spots difference was obtained at 5 µg/mL coating anti-FB1 mAb with 3 × 1010 pfu/mL phage particles and 10 µg/mL B26. With a higer quantity of phage Ab2α nanobody, the intensity of spots was observed increasing background absorbance value. The intensity of spots difference gradually decreased with decreasing the concentrations of coating anti-FB1 mAb and increased with decreasing the concentrations of B26. It is insensitive for the phage Ab2α nanobody without adding B26.
Based on the results described above, the assay sensitivities for each of the three clones were estimated. As shown in (b), the 50% of the maximal signal (SC50) were 1.37 ± 0.61, 0.68 ± 0.03 and 1.47 ± 0.67 ng/mL for Ab2α T3/B26, Ab2α T8/B26 and Ab2α T9/B26, respectively. Among them, Ab2α T8/Ab2β that provided low background signals, which correspond to the absorbance value at the zero analyte concentration of FB1, were selected to set up the noncompetitive idiometric nanobodies phage immunoassay. The noncompetitive assay exhibited the lowest the limit of detection (LOD) of 0.19 ng/mL and the linear range was 0.31–1.74 ng/mL. It presented a 17-fold improvement in the noncompetitve assay sensitivity as compared to the LOD of 3.41 ng/mL on the competivite ELISA that set up with the same primary mAb.
3.3. Cross-reactivity
The selectivity of the noncompetitive idiometric phage ELISA was determined by performing cross-reactivity analyses with other mycotoxins and different primary antibodies. As shown in (a), 6.89% and 2.93% cross-reacivity was observed for FB2 and FB3, respectively, but no cross-reactivity (<0.01%) with other compounds (AFB1, ZEN and OTA). And for other primiary monoclonal antibodies, the cross-reactivity was negligible ((b)). Its specificity should be mostly dependent on the recognition properties of the primary antibody and B26. The results suggesting that when the same primary antibody is used the noncompetitve assay allows us to acquire not only a significant improvement in assay sensitivity but also acceptable specificity.
3.4. Matrix effect
Matrix effects can substantially interfere with enzyme activity, binding between antibody and analyte on ELISAs. For the purpose of minimizing matrix effects, it seems that it is essential to use solid-phase extraction (SPE) as sample pre-treatment method. The dilution of sample extracts with PBS by 5, 10, and 20 times was examined subsequently to evaluate the dilution effect. All dilution times showed a similar behaviour, with SC50 values 0.96 ng/mL (1:1), 0.82 ng/mL (1:5), 0.71 ng/mL (1:10), and 0.81 ng/mL (1:20), compared with 0.66 ng/mL in PBS (). These results indicate that the matrix effects of these corn samples were completely eliminated. using SPE as a cleanup step before assay. Thus far in order to minimize the matrix effects, the extracts were diluted 10-fold with PBS.
Figure 4. Calibration curves of FB1 in PBS and in sample extracts of different dilutions with or without solid phase extraction.
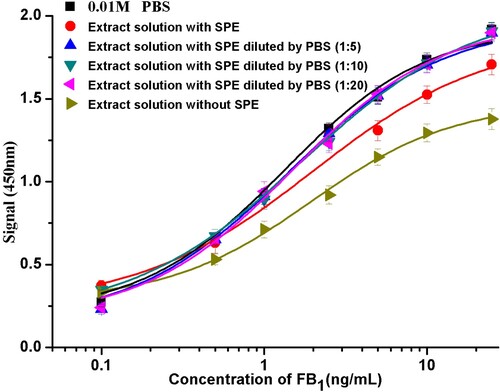
The accuracy and reproducibility of the noncompetitive idiometric nanobodies phage ELISA format were conducted by evaluating the spiked corn samples with various amounts (10–1000 μg/kg) of FB1. Satisfactory recovery rate (mean 74.10%–110.92%) with coefficient variation (mean CV 2.79%–10.95%) for within-assay evaluation were obtained. Meanwhile, the recovery rate estimated for between-assay evaluation was 71.60%–115.34%, with the CV level ranging from 3.17%–11.35% (). These results suggested the accuracy and reproducibility of corn samples were achieved using the noncompetitive idiometric nanobodies phage ELISA.
Table 1. Recoveries of FB1 from the spiked corn samples by the noncompetitive idiometric nanobodies phage ELISA.
Furthermore, we attempted to examine FB1 levels in 25 naturally contaminated cereal samples using the noncompetitive idiometric nanobodies phage ELISA and LC–MS/MS. Results are shown that among the 25 samples, corn and wheat samples were FB1 positive, which three corn samples surpassed the maximum limit for FB1 in cereal (1000 μg/kg), and five rice samples were all FB1 negative (). The correlation (R2) was 0.988 between the two methods (Figure S3). It should be noted that the noncompetitive idiometric nanobodies phage ELISA based on two types of anti-idiotypic nanobody is an acceptable method to detect FB1 in cereal samples.
Table 2. Analysis results of the cereal samples by the noncompetitive idiometric nanobodies phage ELISA and LC–MS/MS.
4. Conclusions
The current work described a sensitive and specific noncompetitive idiometric nanobodies phage immunoassay based on two types of anti-idiotypic nanobodies for the quantitative determination of FB1. The SC50 of the noncompetitive nanobodies phage ELISA was 0.68 ng/mL, with the limit of detection (LOD) of 0.19 ng/mL and the line arc scale ranged from 0.31 ng/mL to 1.74 ng/mL. This assay provided an over 17-fold higher sensitivity, comparing to the competitive ELISA (LOD = 3.41 ng/mL). Using LC–MS/MS for FB1 detecion, the proposed method was successfully validated in actual cereal samples. Overall, it was indicated that the noncompetitive idiometric nanobodies phage ELISA is a prospect format for actual FB1 analysis in cereals. The assay serves as a rapid approach to convert competitive formats to noncompetitive assays for monitoring small molecules, providing significantly improved sensitivity and adaptability to other immunoassay detections.
Supplemental Material
Download ()Disclosure statement
No potential conflict of interest was reported by the authors.
Additional information
Funding
References
- Akter, S., Vehniäinen, M., Kankaanpää, H. T., & Lamminmäki, U. (2017). Rapid and highly sensitive non-competitive immunoassay for specific detection of nodularin. Microorganisms, 5, 58. doi: 10.3390/microorganisms5030058
- Barbas, C. F., Burton, D. R., Scott, J. K., & Silverman, G. J. (2001). Phage display: A laboratory manual. NY: Cold Spring Harbor Laboratory Press.
- Carlomagno, M., Lassabe, G., Rossotti, M., Gonzáleztechera, A., Vanrell, L., & Gonzálezsapienza, G. (2014). Recombinant streptavidin nanopeptamer anti-immunocomplex assay for noncompetitive detection of small analytes. Analytical Chemistry, 86, 10467–10473. doi: 10.1021/ac503130v
- Chung, C. I., Makino, R., Dong, J., & Ueda, H. (2015). Open flower fluoroimmunoassay: A general method to make fluorescent protein-based immunosensor probes. Analytical Chemistry, 87, 3513–3519. doi: 10.1021/acs.analchem.5b00088
- del Rio, B., Redruello, B., Fernandez, M., Martin, M. C., Ladero, V., & Alvarez, M. A. (2019). Lactic acid bacteria as a live delivery system for the in situ production of nanobodies in the human gastrointestinal tract. Frontiers in Microbiology, 9, 3179. doi: 10.3389/fmicb.2018.03179
- Dong, J. X., Xu, C., Wang, H., Xiao, Z. L., Gee, S. J., Li, Z. F., … Yang, J. Y. (2014). Enhanced sensitive immunoassay: Noncompetitive phage anti-immune complex assay for the determination of malachite green and leucomalachite green. Journal of Agricultural and Food Chemistry, 62, 8752–8758. doi: 10.1021/jf5019824
- Faraji, F., Tajik, N., Behdani, M., Shokrgozar, M. A., Zarnani, A. H., Shahhosseini, F., & Habibi-Anbouhi, M. (2018). Development and characterization of a camelid single-domain antibody directed to human CD22 biomarker. Biotechnology and Applied Biochemistry, 65(5), 718–725. doi: 10.1002/bab.1654
- González-Techera, A., Vanrell, L., Last, J. A., Hammock, B. D., & González-Sapienza, G. (2007). Phage anti-immune complex assay: General strategy for noncompetitive immunodetection of small molecules. Analytical Chemistry, 79(20), 7799–7806. doi: 10.1021/ac071323h
- Gujral, N., Yoo, H., Bamdad, F., Suh, J.-W., & Sunwoo, H. (2017). Sensitive double antibody sandwich ELISA for the quantification of phosvitin. Food and Agricultural Immunology, 28, 834–847. doi: 10.1080/09540105.2017.1313821
- Haasnoot, W., & Verheijen, R. (2001). A direct (non-competitive) immunoassay for gentamicin residues with an optical biosensor. Food and Agricultural Immunology, 13, 131–134. doi: 10.1080/09540100120055701
- Hamerscasterman, C. (1993). Naturally occurring antibodies devoid of light chains. Nature, 363, 446–448. doi: 10.1038/363446a0
- Jerne, N. K. (1974). Towards a network theory of the immune system. Annales Dimmunologie, 125C, 373.
- Lan, H., Hong, P., Li, R., Suo, L., Shan, A., Li, S., & Zheng, X. (2017). Internal image anti-idiotypic antibody: A new strategy for the development a new category of prolactin receptor (PRLR) antagonist. Molecular Immunology, 87, 86–93. doi: 10.1016/j.molimm.2017.04.006
- Li, S., Wu, X., Song, S., Zheng, Q., & Kuang, H. (2019). Development of ic-ELISA and an immunochromatographic strip assay for the detection of aristolochic acid I. Food and Agricultural Immunology, 30, 140–149. doi: 10.1080/09540105.2018.1551331
- Li, Y., Zhang, G., Xin, M., Yang, S., De Ruyck, K. D., & Wu, Y. (2018). High sensitivity immunoassays for small molecule compounds detection – novel noncompetitive immunoassay designs. Trac Trends in Analytical Chemistry, 103, 198–208. doi: 10.1016/j.trac.2018.04.008
- Liu, Y., Lin, M., Wu, J., Hu, X., Zhang, X., Xu, C., … Liu, X. (2018). Generation of panels of anti-idiotypic single-chain variable fragments mimicking Cry2Aa toxin using the chain shuffling technique. Food and Agricultural Immunology, 29, 735–743. doi: 10.1080/09540105.2018.1440535
- Niwa, T., Kobayashi, T., Pi, S., Goto, J., Oyama, H., & Kobayashi, N. (2009). An enzyme-linked immunometric assay for cortisol based on idiotype–anti-idiotype reactions. Analytica Chimica Acta, 638, 94–100. doi: 10.1016/j.aca.2009.02.010
- Omi, K., Ando, T., Sakyu, T., Shirakawa, T., Uchida, Y., Oka, A., … Goishi, K. (2015). Noncompetitive immunoassay detection system for haptens on the basis of antimetatype antibodies. Clinical Chemistry, 61, 627–635. doi: 10.1373/clinchem.2014.232728
- Qiu, Y. L., Li, P., Dong, S., Zhang, X., Yang, Q., Wang, Y., … Liu, X. (2018). Phage-mediated competitive chemiluminescent immunoassay for detecting Cry1Ab toxin by using an anti-idiotypic camel nanobody. Journal of Agricultural and Food Chemistry, 66, 950–956. doi: 10.1021/acs.jafc.7b04923
- Saha, D., Roy, D., & Dhar, T. K. (2013). Immunofiltration assay for aflatoxin B1 based on the separation of pre-immune complexes. Journal of Immunological Methods, 392, 24–28. doi: 10.1016/j.jim.2013.03.003
- Shu, M., Xu, Y., Liu, X., Li, Y., He, Q., Tu, Z., … Hammock, B. D. (2016). Anti-idiotypic nanobody-alkaline phosphatase fusion proteins: Development of a one-step competitive enzyme immunoassay for fumonisin B1 detection in cereal. Analytica Chimica Acta, 924, 53–59. doi: 10.1016/j.aca.2016.03.053
- Shu, M., Xu, Y., Wang, D., Liu, X., Li, Y., He, Q., … Wang, X. (2015). Anti-idiotypic nanobody: A strategy for development of sensitive and green immunoassay for fumonisin B₁. Talanta, 143, 388–393. doi: 10.1016/j.talanta.2015.05.010
- Suryoprabowo, S., Liu, L., Kuang, H., Xu, L., Ma, W., & Wu, X. (2019). Development of monoclonal antibody-based colloidal gold immunochromatographic assay for analysis of halofuginone in milk. Food and Agricultural Immunology, 30, 112–122. doi: 10.1080/09540105.2018.1550058
- Tsutsumi, T., Nagata, S., Yoshida, F., Harada, K. I., & Ueno, Y. (2000). Development and application of highly sensitive anti-immune complex ELISAs for microcystins in tap water. Food and Agricultural Immunology, 12, 231–241. doi: 10.1080/09540100050140768
- Tu, Z., Xu, Y., He, Q., Fu, J., Liu, X., & Tao, Y. (2012). Isolation and characterisation of deoxynivalenol affinity binders from a phage display library based on single-domain camelid heavy chain antibodies (VHHs). Food and Agricultural Immunology, 23, 123–131. doi: 10.1080/09540105.2011.606560