ABSTRACT
Abuse of pyrimethanil in crops to cause deleterious effects on humans has been of great concern. In this study, functionalized derivatives of pyrimethanil have been synthesized and conjugated to carrier proteins, and highly specific and sensitive monoclonal antibodies (mAb) have been produced. Based on the mAb 1F05, an ic-ELISA was developed and characterized for the analysis of pyrimethanil residues. The standard curves based on the pyrimethanil matrix calibration ranged from 0.03 to 3 ng/mL, with a 50% inhibitory concentration of 0.25 ng/mL. The limits of detection were 0.02–0.04 μg/kg in various matrices. The recoveries for pyrimethanil ranged from 88.6% to 102.3%, with coefficient of variation below 11%. A good correlation (R2 > 0.9825) between the ic-ELISA and HPLC-MS/MS showed the reliability of the developed ic-ELISA. The proposed ic-ELISA was demonstrated to be a simple, rapid, and sensitive tool to monitor the residues of pyrimethanil in fruits and vegetables.
Introduction
Pyrimethanil (4,6-dimethyl-N-phenyl-2-pyrimidinamine) is a novel strobilurin fungicide belonging to the anilinopyrimidine class (Mandrile, Giovannozzi, Durbiano, Martra, & Rossi, Citation2018). Due to their broad spectrum of fungicidal activity, pyrimethanil is widely used to control a wide range of postharvest fungal diseases on fruits, vegetables and ornamental crops (Shim, Abd El-Aty, Choi, & Kang, Citation2007). Abuse of pyrimethanil has been reported to cause deleterious effects on humans, livestock, crops and the environment (Fenik, Tankiewicz, & Biziuk, Citation2011; Park et al., Citation2013). Therefore, maximum residue limit of 0.2–10 mg/kg for pyrimethanil in food products has been implemented in many countries and regions, including the EU, USA and China (Liang et al., Citation2013; Mandrile et al., Citation2018). To ensure food safety to protect human health, the development of simple, rapid, low cost, and high sensitive monitoring method for pyrimethanil is important in food control.
Many analytical methods, such as surface-enhanced Raman scattering (Mandrile et al., Citation2018), liquid chromatography (Shim et al., Citation2007), gas chromatography (Amvrazi & Tsiropoulos, Citation2009), gas chromatography–mass spectrometry (Gonzalez-Rodriguez, Rial-Otero, Cancho-Grande, & Simal-Gandara, Citation2008; Raeppel et al., Citation2011; Rodriguez-Cabo, Rodriguez, Ramil, & Cela, Citation2011), high-performance liquid chromatography (Zhou et al., Citation2011), liquid chromatography technique coupled with tandem mass spectrometry (Ortelli, Edder, & Corvi, Citation2004; Park et al., Citation2013), and high performance liquid chromatography–tandem mass spectrometry (HPLC–MS/MS) (Liang et al., Citation2013), have been used for the determination of pyrimethanil fungicides in fruit, vegetables and other samples. Although highly sensitive and reliable, these methods are not suitable for the rapid and routine detection of pyrimethanil because they are also time-consuming and require expensive equipment. Compared to instrumental methods, immunoassays, particularly indirect competitive enzyme-linked immunosorbent assay (ic-ELISA), have been used widely in food safety monitoring, as they can provide an inexpensive, simplicity, reliable, sensitive and fast screening alternative for the analysis of trace small molecules analytes in complex matrices.
Recently, Mercader developed ELISA based on polyclonal antibody for pyrimethanil detection, with a 50% inhibitory concentration (IC50) of 2.3–9.8 ng/mL and a limit of detection (LOD) of 0.024 µg/L in carrot juice (Mercader, Esteve-Turrillas, Agullo, Abad-Somovilla, & Abad-Fuentes, Citation2012). In comparison with polyclonal antibody, the monoclonal antibody (mAb) recognizing a single epitope on pyrimethanil molecule is not only less cross-reactivity with structure related fungicides but also reduces the possibility of matrix interferences. Esteve-Turrillas and co-workers have established an ELISA based on mAb for the detection of pyrimethanil, with an IC50 value of 0.22–12.3 ng/mL and an LOD of 0.04–0.05 ng/mL in spiked strawberry, tomato and cucumber (Esteve-Turrillas, Mercader, Agullo, Abad-Somovilla, & Abad-Fuentes, Citation2015b). Now, to better meet the market rapid testing demand, safe, simple and safe sample pretreatment methods for the extraction of different fungicides are also important. In this work, we aimed to prepare a highly specific and sensitive mAb to pyrimethanil and to develop a highly sensitive ic-ELISA for the detection of pyrimethanil in fruits and vegetables. A modified QuEChERS (quick, easy, cheap, effective, rugged and safe) was employed for extraction and purification of pyrimethanil residues in different food samples (e.g. grape, strawberry, carrot, peach, apple, cucumber matrices, etc.) by ic-ELISA. Meanwhile, the established ic-ELISA was characterized in terms of specificity, calibration curves, precision and reproducibility. Comparisons are made between the developed ic-ELISA method and the established HPLC-MS/MS method for the analysis pyrimethanil in spiked samples and real samples. There was no significant difference between these two methods.
Materials and methods
Materials and reagents
Pyrimethanil and the employed pesticide standards were purchased from WITEGA Laboratorien (Berlin, Germany). Pesticide stock solutions were prepared in N,N-dimethylformamide and kept at −20°C in amber glass vials. Complete and incomplete freund’s adjuvants, bovine serum albumin (BSA), human serum albumin (HSA) and ovalbumin (OVA) were obtained from Sigma-Aldrich (St. Louis, MO). Peroxidase-labelled goat anti-mouse IgG (IgG-HRP) was supplied by Sino-American Biotechnology (Shanghai, Branch, China). A mouse monoclonal antibody isotyping kit was purchased from Pierce Biotechnology, Inc. (Rockford, IL, USA). Microtiter plates, microculture plates, and cell culture bottles were obtained from Costar Group, Inc. (Bethesda, MD, USA).
Buffers and solutions
The following solutions were used in the experiments. (a) incubation solution, 0.01 M phosphate-buffered saline (PBS) buffer, pH 7.2; (b) coating solution, 0.05 M carbonate buffer, pH 9.6; (c) washing solution, 0.01 M PBS buffer containing 0.1% Tween-20 (PBST), pH 7.2; (d) blocking solution, 0.01 M PBS buffer with 1% (w/v) OVA, pH 7.2; (e) TMB substrate solution prepared by adding 3.3 mg TMB in 250 mL dimethyl sulfoxide to 25 mL of citrate-phosphate buffer containing 3.25 mL of a 30% H2O2 solution; (f) stopping reagent, 2 M H2SO4 solution.
Hapten synthesis and activation
As demonstrated in , hapten 6-(3-(4,6-dimethylpyrimidin-2-ylamino)phenyl)hexanoic acid (pMm) was synthesized as previously reported method with some modifications (Esteve-Turrillas et al., Citation2015b). Briefly, 285.6 mg of 2-chloro-4,6-dichloropyrimidine (2.00 mmol) and 414 mg of 6-(3-aminophenyl)hexanoic acid (2.00 mmol) were dissolved in 10 mL anhydrous 1,4-dioxane, and heated under reflux for 8 h with magnetic stirring under nitrogen atmosphere. After the mixture was cooled to room temperature and concentrated to dryness under reduced pressure. The obtained residue was dissolved in approximately 1 mL of formic acid and the product precipitated by slow addition of approximately 3 mL of water. The precipitate was filtered off, washed several times with cold water and dried under vacuum to afford hapten pMm.
Figure 1. Chemical structures of the three anilinopyrimidine fungicides (pyrimethanil, cyprodinil and mepanipyrim) and of the three pyrimethanil haptens (pMm, pMn and pMp).
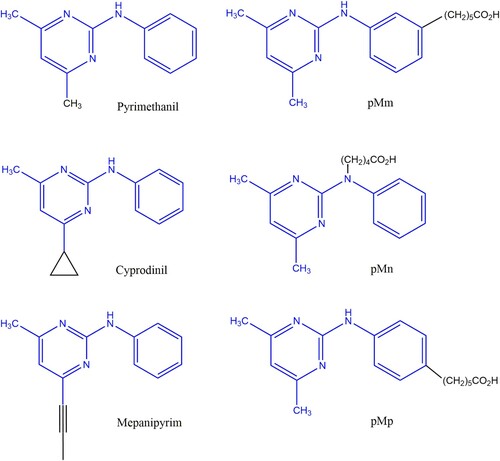
The hapten pMm (0.10 mmol) and 33 mg of N,N’-disuccinimidyl carbonate (0.13 mmol) were dissolved in anhydrous 1 mL of CH3CN under nitrogen atmosphere. The reaction mixture was gently stirred for 12 h at room temperature. Then, the solution was diluted with chloroform, washed with an aqueous saturated solution of NaHCO3 and brine and then dried over anhydrous Na2SO4. Flash column chromatography of the residue that was left after evaporation of the solvent, using chloroform as the eluent, afforded the corresponding N-succinimidyl ester of hapten pMm for further coupling to proteins.
Synthesis of antigens
The synthetic route for the preparation of the immunogens and coating antigens is shown in . Briefly, 200 μL of N-succinimidyl ester hapten pMm solution (50 mM) in DMF was added dropwise to 2 mL of a 15 mg/mL BSA solution in coating buffer. The conjugates reaction were gently stirred at room temperature for 5 h and purified on Sephadex G-25. The eluted conjugates were then dialysed against PBS (0.01 M, pH 7.4) at 4°C for 3 days and then stored at 4°C. The pMm-HSA and pMm-OVA antigens were prepared as described in the pMm-BSA synthesis section, except that BSA was replaced with HSA or OVA. The formed pMm-BSA and pMm-HSA were used as immunogens, and pMm-OVA was coating antigens. The conjugate synthesis was verified and molar ratios of hapten to protein were estimated using an Ultraviolet Visible Spectrophotometer (Agilent Technologies, Palo Alto, CA, USA). The number of hapten conjugated to the carrier molecules was estimated according to the ultraviolet absorbance spectra of the haptens, carrier proteins, and conjugates as follows: [ε(conjugation) − ε(protein)]/ε(hapten), where ε is the absorbance coefficient of the analytes (Peng et al., Citation2016).
Monoclonal antibody preparation
All animal experiments that were described in this study were performed in adherence to the Chongqing Normal University animal experiment centre guidelines and approved by the Animal Ethics Committee. Animals were immunized with pMm-BSA or pMm-HSA conjugates following equivalent procedures to those used in a previous study (Sun, Luo, Zhang, Zhang, & Le, Citation2018). Briefly, each female BALB/c mice (8–10 weeks old) supplied by the Chongqing Center for Disease Control and Prevention were immunized by subcutaneous injection with different doses (75, 100 and 125 μg) of pMm-BSA or pMm-HSA conjugates in 1 mL of a 1:1 mixture of PBS and complete Freund’s adjuvant. It was then injected subcutaneously into multiple sites on the back of each mouse. After three booster doses of pMm-BSA or pMm-HSA in incomplete Freund’s adjuvant at 2-week intervals, the antiserum titre was determined by a non-competitive indirect ELISA. Three days before cell fusion, the mouse exhibiting the highest titre and specificity was immunized with a final dose of pMm-BSA or pMm-HAS (75, 100 and 125 μg) in distilled PBS. Cell fusion procedures were performed as previously described with some modifications (Le et al., Citation2011; Ngom et al., Citation2011). Hybridoma cell lines were produced through the fusion of myeloma cells (Sp2/0) at a ratio of 5-10:1, and spleen cells obtained from immunized mice. Hybridoma from wells having a positive response in the ELISA described below were cloned three by limiting dilution method. Stable antibody-producing clones were expanded until monoclones were obtained. After cell culture, the hybridoma cell was intraperitoneally injected into paraffin-primed mice to produce ascitic fluids. Ascitic fluids were collected purified by the caprylic acid and ammonium sulphate precipitation method followed using a protein G affinity column (Amersham Biosciences, Uppsala, Sweden) according to the manufacturer’s protocol. The purified mAb was stored at –20°C before use.
The mAbs raised against each of the immunizing conjugates were screened against each of the corresponding coating antigens in a checkerboard for the best dilution of the coating conjugate and antiserum. The extent of cross-reactivity was assessed by determining the IC50 values with the ic-ELISA. The cross-reactivity of mAb to various pyrimethanil and their related derivatives was tested using the ic-ELISA described, which was determined by measuring their IC50 values. Several pyrimethanil analogues such as cyprodinil, mepanipyrim, kresoxim-methyl, trifloxystrobin, pyraclostrobin, azoxystrobin, dimoxystrobin, fluoxastrobin, picoxystrobin, fenhexamid, captan, procymidone, atrazine, pyriftalid, and fenarimol were selected for testing cross-reactivity. The concentrations of the above compounds covered the range from 0.01 to 1000 ng/mL. Cross-reactivity values were calculated as follows: cross-reactivity (%) = 100×IC50 value of pyrimethanil/IC50 value of competitor. The mAb with the lowest IC50 for pyrimethanil was selected for the subsequent experiments.
Development of the ic-ELISA
The protocol of ic-ELISA was carried out using the methodology described previously with a slight modification (Le et al., Citation2009; Le et al., Citation2013). Microwell plates were coated with 100 µL per well of pMm-OVA conjugate diluted in carbonate buffer (pH 9.6) and incubated overnight at 4°C. The plates were washed three times with PBST, and the excess binding sites were blocked by adding 200 µL of blocking buffer at 37°C for 1 h. The plates were washed three times with PBST, and then added 50 µL per well of mAb plus 50 µL per well of standard samples or extracts were added to plates. The reaction was took place during at room temperature for 1 h and the plates were washed again. Then 100 µL per well of IgG-HRP conjugate (1:5000) was added, and the plates were incubated at room temperature for 1 h. After washing the plate with PBST, 100 µL per well of TMB substrate solution were added and incubated for 15 min at 37°C in the dark. Then, the reaction was stopped with 50 μL per well of stopping reagent. The absorbance measured at 450 nm using a Tecan Sunrise 2.5 Microplate Reader (SUNRISE, Austria). A calibration curve was generated in the form (B/B0) × 100% vs. log C, where B and B0 were the absorbance of the analyte at the standard point and at zero concentration of the analyte, respectively. The IC50 was determined as a measure of the sensitivity of the ic-ELISA. The LOD was based on 20 blank samples accepting no false positive rates.
Sample preparation
Grape, strawberry, carrot, peach, apple, and cucumber plants were purchased from Yonghui supermarket (Chongqing, China). Samples without detectable pyrimethanil determined by HPLC-MS/MS were used as negative controls. The modified QuEChERS method was employed for extraction and purification of fungicide residues from food samples (Lehotay, Citation2007). Briefly, the samples were cut into pieces and homogenized. Fifteen grams of each sample were weighed in a 50 mL plastic centrifuge tube and 15 mL of acetonitrile was added. The mixture was homogenized at 10,000 rpm for 3 min, and then 2 g of sodium chloride and 4 g of anhydrous magnesium sulphate were added. The supernatant was concentrated on a rotary evaporator at 40°C and redissolved to 1 mL with acetonitrile. The clean extracts were filtered with a 0.2 μm filter membrane and analysed by the ic-ELISA and HPLC-MS/MS method. The procedure for HPLC–MS/MS analysis was described in the literature (Liang et al., Citation2013).
Spiked sample analysis and comparison of the ic-ELISA with HPLC–MS/MS
Negative-fungicide grape, strawberry, carrot, peach, apple, and cucumber crops samples previously confirmed by HPLC-MS/MS analysis were spiked with concentrations 1, 10, and 50 μg/kg of pyrimethanil, and then analysed by ic-ELISA and HPLC–MS/MS. The analyses were performed at five replicates for each concentration (n = 5). Each sample was analysed using ic-ELISA to demonstrate the range of blank matrix effects and to determine the LOD. The determination of LOD was based on 20 blank samples accepting no false positive rates, with an average +3 standard deviation (SD). The accuracy and precision of the method were represented by the recovery and coefficient of variation (CV), respectively. Recoveries (%) of spiked pyrimethanil were determined using five spiked duplicate blanks at 1, 10, and 50 μg/kg of pyrimethanil, were divided equally. Half of these samples were subjected to the ic-ELISA analysis, and the other half were subjected to HPLC–MS/MS analysis. The recovery (%) was calculated by the following equation: (conc. measured/conc. spiked) × 100. CVs were determined from the analysis of the above samples spiked with pyrimethanil at three different levels for five different analyses. Analysis of each concentration level was repeated five times over a time span of two months. The correlations between the ic-ELISA and HPLC–MS/MS analysis in terms of the detection of pyrimethanil in the spiked samples were calculated.
Detection local market samples by ic-ELISA and HPLC–MS/MS
To assess the detection capability and accuracy of the proposed ic-ELISA for real sample, 60 unknown real samples, including 10 grape samples, 10 strawberry samples, 10 carrot samples, 10 peach samples, 10 apple samples, and 10 cucumber samples, were randomly purchased from local markets in Chongqing city (Chongqing, China). These samples were analysed and compared by ic-ELISA and HPLC-MS/MS method.
Results and discussion
Hapten design and antigen preparation
The anilinopyrimidine class of fungicides are small molecules that must be conjugated to carrier proteins to render them immunogenic. Several haptens of anilinopyrimidines fungicide had been designed to obtain the special antibodies against pyrimethanil (Esteve-Turrillas et al., Citation2015b; Mercader et al., Citation2012) and/or cyprodinil (Esteve-Turrillas, Agullo, Abad-Fuentes, Abad-Somovilla, & Mercader, Citation2012; Esteve-Turrillas, Abad-Somovilla et al., Citation2015; Esteve-Turrillas et al., Citation2015a) in previous studies. In this study, the main aims of the hapten design were to produce the monoclonal antibody against pyrimethanil, and establish an ic-ELISA. The design and synthesis of haptens is the key step in the development of a rapid immunoassay method for antibiotics residue. If the analyte provides reactive groups (–NH2, –COOH and –OH), the active group of the analyte can be added to the linker, and then the haptens were combined with the carrier protein to successfully synthesize the immunogen to prepare a specific antibody. However, pyrimethanil do not have reactive groups for direct coupling to a protein. In the previous study, three pyrimethanil derivatives (pMn, pMm and pMp) with different linker tethering sites were prepared (), and which employed in the production of immunizing conjugates for screening antibody (Esteve-Turrillas et al., Citation2015b; Mercader et al., Citation2012). In this study, a novel synthetic method was employed to synthesize hapten pMm (). Compared with Mercader’s and Esteve-Turrillas’s method, the novel synthetic method not only simplified the operation and shortened the total reaction time, but also reduced the use of raw materials. As a hapten, it should be identical to the target molecule in structure, stereochemistry, electron distribution and hydrophobic properties. In general, the introduced linker should have no effect on the electronic distribution of the hapten. In this work, a novel regioisomeric immunizing haptens have been designed with the linker at the aniline ring (pMm) of the pyrimethanil molecule (). After coupling with the spacer arm at the positions of the aniline ring of the target pyrimethanil, the electronic properties will undergo a minimal change and there may also be a slight alteration in geometry. In terms of electrostatic potentials and molecular charges, pMm mimicked the target analyte pyrimethanil more authentically than haptens pMn and pMp. Our results were inconsistent with data described by Esteve-Turrillas and co-workers (Esteve-Turrillas, Abad-Somovilla et al., Citation2015).
In addition, the kinds of carrier protein and hapten-protein ratios are very important parameter which generally affects the sensitivity of the obtained antibodies. BSA, HSA and OVA are popular carrier protein for conjugation to haptens. Therefore, they were covalently attached to haptens PMm using the modified active ester method using N,N’-disuccinimidyl carbonate to produce antigens in this study. All synthetic antigens were characterized by an 8453 UV-Visible spectrophotometer. The ultraviolet absorbance spectra of pMm-BSA (λmax, 278 nm), pMm-HSA (λmax, 278 nm) and pMm-OVA (λmax, 276 nm) were different from those of pMm (λmax, 268 nm), BSA (λmax, 280 nm), HSA (λmax, 280 nm) and OVA (kmax, 278 nm). The estimated incorporation rates of pMm-BSA, pMm-HSA and pMm-OVA are 5.2, 4.8 and 2.6, respectively. These results suggested that the conjugates were successfully synthesized. A number of studies have demonstrated that lower incorporation rates induced a slower immune response with higher affinity antibodies (Zhao et al., Citation2017). In the present study, incorporation rates of pMm-BSA, pMm-HSA and pMm-OVA are 16, 3 and 7, respectively (Esteve-Turrillas et al., Citation2015b). Obviously, compared with the incorporation rates of this study were lower than the previous studies.
Characterization of the bleeding serum and the monoclonal antibody
Two immunogens pMm-BSA and pMm-HSA were used to inject mice with at three different doses (75, 100, and 125 μg) to elicit specific antibodies against pyrimethanil. The titre and specificity of the antisera from the immunized mice were determined by ELISA (shown in ). Eighteen mice were immunized and showed varying antisera titres ranging from 1:800 to 1:12,800. It was clear that the serum from the mice immunized with middle dose (100 μg) exhibited a higher titre than the mice immunized with high dose (150 μg) and low dose (50 μg). In addition, the serum from the mice immunized with the middle dose (100 μg) of pMm-BSA exhibited more sensitivity toward pyrimethanil than the serum from the other immunized mice.
Table 1. Titre and specificity of the immunized mice.
Based on the serum characterization results, the spleen cells from the mouse that was immunized with the middle dose (100 μg) of pMm-BSA were used for the fusion experiment. After cell fusion and cloning, we obtained seven hybridomas (referred to as 1A11, 1B04, 1F05, 1H12, 2B09, 2G07, and 2E08), which secreted high affinity and stable mAbs against pyrimethanil. The sensitivity of mAbs was investigated by testing their ability to inhibit pyrimethanil (1 ng/mL). The titres of mAbs were determined by the indirect ELISA (). Among them, mAb 1F05 showed the highest titre and best sensitivity toward pyrimethanil. Therefore, it was selected to produce the mAb. The obtained mAb 1F05 was identified as the IgG1 isotype possessing a kappa light chain by a mouse monoclonal isotyping kit. This hybridoma (1F05) was used for further evaluation of mAb specificity and subsequent immunoassay development.
Table 2. The titre of each monoclonal antibody, and its sensitivity to pyrimethanil.
The standard curve and the specificity for the ic-ELISA
The optimum conditions for the ic-ELISA were determined as a coating antigen (pMm-OVA) concentration of 0.5 mg/L and a mAb (1F05) dilution ratio of 1:16,000. Under the optimized conditions mentioned above, the ic-ELISA standard curve for pyrimethanil detection was constructed in concentrations of 0.001–100 ng/mL and shown in (a). After transposing, as shown in (b), the good linearity was between 0.03 and 3 ng/mL with acceptable correlation coefficients (R2 = 0.9918). With regarding to assay sensitivity, IC50 value was 0.25 ng/mL. The LOD for pyrimethanil was determined by repeated analysis (n = 5) of pyrimethanil-PBS samples. The LOD was 0.02 ng/mL and the within-assay CV was less than 15%.
Figure 3. The standard curve for pyrimethanil using ic-ELISA: (a) typical best fit calibration curves of the ic-ELISA with pyrimethanil concentration range from 0.001 to 100 ng/mL and (b) the linearity was good from 0.03 to 3 ng/mL which has a linear regression equation and acceptable correlation (R2). Note that B and B0 are the absorbance of samples with and without pyrimethanil, respectively (n = 5).
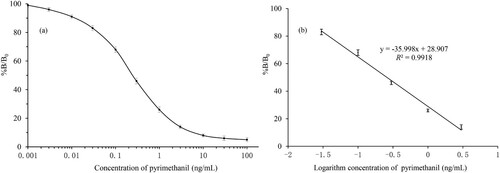
Based on this standard curve, the cross-reactivity of the obtained mAb 1F05 was calculated according to the above formula. The mAb 1F05 showed cross-reactivity towards pyrimethanil (100%), and cross-reactivity of only 2.3% with cyprodinil and 1.1% with mepanipyrim (). The mAb 1F05 did not generate significant cross-reactivity (<0.01) with other analytes listed above. The obtained mAb 1F05 exhibit more excellent sensitivity and specificity to pyrimethanil than those reported antibodies previously (Esteve-Turrillas et al., Citation2015; Mercader et al., Citation2012). These results indicated that the optimized ic-ELISA had a high specificity and could be applied for determination of pyrimethanil in samples.
Table 3. Cross-reactivity of the mAb 1F05 to pyrimethanil and related compounds tested by ic-ELISA.
Spiked sample analysis and comparison of ic-ELISA with HPLC-MS/MS
To determine of accuracy and precision of the ic-ELISA, fungicide-free grape, strawberry, carrot, peach, apple, and cucumber samples spiked with pyrimethanil at levels of 1, 10 and 50 μg/kg. Spiked samples were extracted by modified QuEChERS and analysed by the developed ic-ELISA and HPLC-MS/MS. As shown in , based on the results from the 20 different blank samples, the LODs of ic-ELISA ranged from 0.02 to 0.04 μg/kg in various product matrices. The recoveries and coefficients of variation were found to be 88.6–102.3% and 5.76–10.7%, respectively. It is also worthwhile to mention that a side-by-side comparison of the ic-ELISA and HPLC-MS/MS detection results showed that there was an excellent correlation between results from ic-ELISA and HPLC–MS/MS (R2 > 0.9825). It shows that the proposed ic-ELISA method is reliable for the detection of pyrimethanil residue in fruits and vegetables.
Table 4. Mean recoveries and coefficients of variation (CV) for pyrimethanil in fruit and vegetables and the correlations between the results of ic-ELISA and HPLC-MS/MS (n = 5).
Comparison between ic-ELISA and HPLC-MS/MS for the analysis of samples from local markets
A side-by-side comparison between ic-ELISA and HPLC-MS/MS was achieved by testing 60 samples. The result is that the detection of the sixty unknown real samples by the ic-ELISA and HPLC-MS/MS analyses indicated that no pyrimethanil were detected. These results demonstrated that the developed ic-ELISA was a robust and reliable method for the detection of pyrimethanil in fruits and vegetables.
Conclusion
The present study described the generation and characterization of specific mAb against pyrimethanil. Based on this excellent mAb, a highly sensitive and specific ic-ELISA method coupled with a simple sample preparation procedure to detect pyrimethanil in fruits and vegetables was developed. The described methodology showed excellent analytical parameters, with LODs of 0.02–0.04 μg/kg in various product matrices. Recovery rates of 88.6–102.3% and coefficients of variation of 5.7–10.7% demonstrated high accuracy and precision of the assay. The accuracy and precision of ic-ELISA were validated by the instrumental method of HPLC–MS/MS with good correlations (R2 > 0.9825). Results showed obviously better performances than the previously reported ELISA methods (Esteve-Turrillas et al., Citation2015; Mercader et al., Citation2012). In conclusion, developed ic-ELISA is a simple, rapid, sensitive, accurate and reproducible analytical method for the analysis of pyrimethanil in fruits and vegetables.
Disclosure statement
No potential conflict of interest was reported by the authors.
Additional information
Funding
References
- Amvrazi, E. G., & Tsiropoulos, N. G. (2009). Application of single-drop microextraction coupled with gas chromatography for the determination of multiclass pesticides in vegetables with nitrogen phosphorus and electron capture detection. Journal of Chromatography A, 1216(14), 2789–2797. doi: 10.1016/j.chroma.2008.07.070
- Esteve-Turrillas, F. A., Abad-Somovilla, A., Quinones-Reyes, G., Agullo, C., Mercader, J. V., & Abad-Fuentes, A. (2015). Monoclonal antibody-based immunoassays for cyprodinil residue analysis in QuEChERS-based fruit extracts. Food Chemistry, 187, 530–536. doi: 10.1016/j.foodchem.2015.04.119
- Esteve-Turrillas, F. A., Agullo, C., Abad-Fuentes, A., Abad-Somovilla, A., & Mercader, J. V. (2012). Immunoreagent generation and competitive assay development for cyprodinil analysis. Journal of Agricultural and Food Chemistry, 60(19), 4803–4811. doi: 10.1021/jf300319n
- Esteve-Turrillas, F. A., Mercader, J. V., Agulló, C., Abad-Somovilla, A., & Abad-Fuentes, A. (2015a). Moiety and linker site heterologies for highly sensitive immunoanalysis of cyprodinil in fermented alcoholic drinks. Food Control, 50, 393–400. doi: 10.1016/j.foodcont.2014.09.023
- Esteve-Turrillas, F. A., Mercader, J. V., Agullo, C., Abad-Somovilla, A., & Abad-Fuentes, A. (2015b). Site-heterologous haptens and competitive monoclonal antibody-based immunoassays for pyrimethanil residue analysis in foodstuffs. Lwt-Food Science and Technology, 63(1), 604–611. doi: 10.1016/j.lwt.2015.03.074
- Fenik, J., Tankiewicz, M., & Biziuk, M. (2011). Properties and determination of pesticides in fruits and vegetables. Trends in Analytical Chemistry, 30, 814–826. doi: 10.1016/j.trac.2011.02.008
- Gonzalez-Rodriguez, R. M., Rial-Otero, R., Cancho-Grande, B., & Simal-Gandara, J. (2008). Determination of 23 pesticide residues in leafy vegetables using gas chromatography-ion trap mass spectrometry and analyte protectants. Journal of Chromatography A, 1196, 100–109. doi: 10.1016/j.chroma.2008.02.087
- Le, T., Xu, J., He, H., Niu, X., Chen, Y., & Jia, Y. (2013). Development and validation of an enzyme-linked immunosorbent assay for rapiddetection of multi-residues of five quinoxaline-1,4-dioxides in animal feeds. Food and Agricultural Immunology, 24(4), 457–466. doi: 10.1080/09540105.2012.716024
- Le, T., Yu, H., Guo, Y., Ngom, B., Shen, Y., & Bi, D. (2009). Development of an indirect competitive ELISA for the detection of doxycycline residue in animal edible tissues. Food and Agricultural Immunology, 20(2), 111–124. doi: 10.1080/09540100902849740
- Le, T., Yu, H., Wang, X. L., Ngom, B., Guo, Y. C., & Bi, D. R. (2011). Development and validation of an immunochromatographic test strip for rapid detection of doxycycline residues in swine muscle and liver. Food and Agricultural Immunology, 22(03), 235–246. doi: 10.1080/09540105.2011.556713
- Lehotay, S. J. (2007). Determination of pesticide residues in foods by acetonitrile extraction and partitioning with magnesium sulfate: Collaborative study. Journal of AOAC International, 90(2), 485–520.
- Liang, X., Liu, X., Dong, F., Xu, J., Qin, D., Li, Y., … Zheng, Y. (2013). Simultaneous determination of pyrimethanil, cyprodinil, mepanipyrim and its metabolite in fresh and home-processed fruit and vegetables by a QuEChERS method coupled with UPLC-MS/MS. Food Additives & Contaminants: Part A, 30(4), 713–721. doi: 10.1080/19440049.2013.768777
- Mandrile, L., Giovannozzi, A. M., Durbiano, F., Martra, G., & Rossi, A. M. (2018). Rapid and sensitive detection of pyrimethanil residues on pome fruits by surface enhanced Raman scattering. Food Chemistry, 244, 16–24. doi: 10.1016/j.foodchem.2017.10.003
- Mercader, J. V., Esteve-Turrillas, F. A., Agullo, C., Abad-Somovilla, A., & Abad-Fuentes, A. (2012). Antibody generation and immunoassay development in diverse formats for pyrimethanil specific and sensitive analysis. The Analyst, 137(23), 5672–5679. doi: 10.1039/c2an35801h
- Ngom, B., Jin, X.E., Guo, Y.C., Shi, D.S., Zeng, Y., Le, T., … Bi, D.R. (2011). Monoclonal antibody against sulfaquinoxaline and quantitative analyses in chicken tissues by competitive indirect ELISA and lateral flow test strip immunoassay. Food and Agricultural Immunology, 22(1), 1–16. doi: 10.1080/09540105.2010.514895
- Ortelli, D., Edder, P., & Corvi, C. (2004). Multiresidue analysis of 74 pesticides in fruits and vegetables by liquid chromatography-electrospray-tandem mass spectrometry. Analytica Chimica Acta, 520(1-2), 33–45. doi: 10.1016/j.aca.2004.03.037
- Park, J. H., Park, J. S., Abd El-Aty, A. M., Rahman, M. M., Na, T. W., & Shim, J. H. (2013). Analysis of imidacloprid and pyrimethanil in shallot (Allium ascalonicum) grown under greenhouse conditions using tandem mass spectrometry: Establishment of pre-harvest residue limits. Biomedical Chromatography, 27(4), 451–457. doi: 10.1002/bmc.2812
- Peng, D., Yang, B., Pan, Y., Wang, Y., Chen, D., Liu, Z., … Yuan, Z. (2016). Development and validation of a sensitive monoclonal antibody-based indirect competitive enzyme-linked immunosorbent assay for the determination of the aflatoxin M1 levels in milk. Toxicon, 113, 18–24. doi: 10.1016/j.toxicon.2016.02.006
- Raeppel, C., Nief, M., Fabritius, M., Racault, L., Appenzeller, B. M., & Millet, M. (2011). Simultaneous analysis of pesticides from different chemical classes by using a derivatisation step and gas chromatography-mass spectrometry. Journal of Chromatography A, 1218(44), 8123–8129. doi: 10.1016/j.chroma.2011.08.098
- Rodriguez-Cabo, T., Rodriguez, I., Ramil, M., & Cela, R. (2011). Dispersive liquid-liquid microextraction using non-chlorinated, lighter than water solvents for gas chromatography mass spectrometry determination of fungicides in wine. Journal of Chromatography A, 1218(38), 6603–6611. doi: 10.1016/j.chroma.2011.07.054
- Shim, J. H., Abd El-Aty, A. M., Choi, J. H., & Kang, C. A. (2007). Determination of field-incurred pyrimethanil residues in persimmon (Diospyros kaki Linn) by liquid chromatography. Biomedical Chromatography, 21(12), 1279–1283. doi: 10.1002/bmc.884
- Sun, Q., Luo, J., Zhang, L., Zhang, Z., & Le, T. (2018). Development of monoclonal antibody-based ultrasensitive enzyme-linked immunosorbent assay and fluorescence-linked immunosorbent assay for 1-aminohydantoin detection in aquatic animals. Journal of Pharmaceutical and Biomedical Analysis, 147, 417–424. doi: 10.1016/j.jpba.2017.06.068
- Zhao, L., Li, J., Li, Y., Wang, T., Jin, X., Wang, K., … Zhou, F. (2017). Preparation of monoclonal antibody and development of an indirect competitive enzyme-linked immunosorbent assay for ornidazole detection. Food Chemistry, 229, 439–444. doi: 10.1016/j.foodchem.2017.02.100
- Zhou, Y. W., Han, L. T., Cheng, J., Guo, F., Zhi, X. R., Hu, H. L., & Chen, G. (2011). Dispersive liquid-liquid microextraction based on the solidification of a floating organic droplet for simultaneous analysis of diethofencarb and pyrimethanil in apple pulp and peel. Analytical and Bioanalytical Chemistry, 399(5), 1901–1906. doi: 10.1007/s00216-010-4567-x