ABSTRACT
Benzo[a]pyrene (BaP), a carcinogen, leads to the severe liver injury. Isoorientin (ISO), a natural flavonoid, is known for its bioactivities, including anti-oxidation, anti-inflammation, and so on. However, there is no report on the effects of ISO on liver injury induced by BaP. The present study demonstrated that ISO treatment could relieve BaP-caused autophagic and pyroptotic injury in HL-7702 human hepatocyte and male BALB/c mice by down-regulating the numbers of autophagosomes, the mRNA expression of LC3 II and Beclin-1, and the levels of pyroptotic characteristic indices. Histopathology stained also confirmed that ISO could suppress the BaP-induced liver injury. Meanwhile, ISO was able to improve the liver function, and remarkably (p < 0.01) ameliorate hepatic oxidative injury by enhancing antioxidant enzymes activities (T-SOD, GSH-px) and reducing the levels of MDA and H2O2. In conclusion, ISO possesses the positive inhibitive effects on BaP-induced the autophagic and pyroptotic liver injury in vitro and vivo.
1. Introduction
Isoorientin (3′,4′,5,7-tetrahydroxy-6-C-glucopyranosyl flavone, ISO, (A)), a natural flavonoid compound, exists in the edible plants and some foods, such as buckwheat (Watanabe & Ito, Citation2002), passion fruit (Toda, Shoketsu, Shogo, Norihito, & Hiroshi, Citation2017) and corn silks (Widstrom & Snook, Citation1998). ISO possesses the multiple pharmacological activities, including anti-oxidant activity, anti-inflammatory activity (Anilkumar et al., Citation2017; Lee, Ku, & Bae, Citation2014), and so on. Besides, many studies show that ISO has the notable hepatoprotective effects, for example attenuated CCl4-induced hepatic fibrosis in Male Sprague–Dawley rats through enhancing the free radical scavenging and the activities of antioxidant enzymes (T-SOD, GSH-px) (Lin et al., Citation2015). Our previous studies also found that ISO could protect BRL-3A buffalo rat liver cells from H2O2-induced apoptosis by reducing NO production and elevating T-SOD levels (Yuan et al., Citation2013), and could prevent liver injury by regulating lipid metabolism, antioxidant capability, and inflammatory cytokine release in high-fructose-fed mice (Yuan, Han, Li, Ren, & Yang, Citation2016).
Benzo[a]pyrene (BaP, (B)), a carcinogen and teratogen, is widely produced in a large number of the high-temperature processed foods, such as fried chicken, barbecue and smoked foods (Phillips, Citation1999). It has been reported that the somatic mutations (IARC, Citation2014), oxidative stress (Briede et al., Citation2004), lysosomal membrane disruption (Grundy, Moore, Howell, & Ratcliffe, Citation1996), the dysregulation of the expression levels of microRNA and cytokine (Zhang, Wang, et al., Citation2014), the induction the cytochrome P450 family of oxidases (Bengtsson, Montelius, Mankowitz, & Rydstrom, Citation1983), and the impairment of the female fertility (Sobinoff, Pye, Nixon, Roman, & Mclaughlin, Citation2012) after exposure to BaP had been found. Liver is the target organ of BaP exposure (Chen, Song, Diao, & Zhou, Citation2016; Ji et al., Citation2016). Numerous studies show that BaP results in liver injury through affecting the hepatic function (Fanali, Franco-Belussi, Bonini-Domingos, & Oliveira, Citation2018), inducing the oxidative stress (Ma, Liu, Qin, Jiang, & Sun, Citation2011), activating the aryl hydrocarbon receptor, disrupting carbohydrate and cholesterol metabolism (Jiang et al., Citation2011; Miller & Ramos, Citation2001; Sadikovic, Andrew, Carter, Robinson, & Rodenhiser, Citation2008), and inducing apoptosis (Das, Das, Samadder, Paul, & Khuda-Bukhsh, Citation2013; Weiping, Rong, Qiao, Gil, & Huidong, Citation2018). Our previous study found that BaP could damage HL-7702 cells by inducing apoptosis (Chunxia et al., Citation2018), autophagy and pyroptosis (Yuan, Liu, Deng, & Gao, Citation2017). It has been found that natural flavonoids (β-Naphthoflavone (Adrian, Manuel, Jesus, & Mıguez, Citation2008), baicalein and wogonin (Ueng et al., Citation2001), silmyarin (Perumal, Muruganantham, Subramanian, Shunmugiah, & Kasi, Citation2012), Rutin (Marcarini et al., Citation2011) and naringenin (Ali et al., Citation2016)) are able to prevent or inhibit BaP-induced liver injury. However, the effect of ISO on liver injury induced by BaP remains unknown.
The objective of the present study was to demonstrate whether ISO could weaken the autophagic and pyroptotic liver injury induced by BaP in HL-7702 cells and male BALB/c mice, respectively, through evaluating the autophagic and pyroptotic characteristic indices, the liver function and the antioxidant properties in liver tissue. Our study aimed to provide a theoretical basis for that ISO could be served as an effective approach and a new available food supplement to terminate the liver injury induced by BaP.
2. Materials and methods
2.1. Chemicals and reagents
BaP (purity ≥ 96%), MTT, trypan blue and monodansyl cadaverine (MDC) were purchased from Sigma (St. Louis, MO, USA). ISO (purity ≥ 98%) was purchased from Pharmaceutical Technology Co., Ltd. (Jiangsu, China). Sodium carboxymethyl cellulose (CMC) was obtained from Talida New Material Co., Ltd. (Jiangsu, China). Olive oil was from Sinopharm Chemical Reagent Co., Ltd. (Shanghai, China). 2,7-dichlorodihydrofluorescein diacetate (H2DCFDA), 5,5′,6,6′-Tetrachloro-1,1′,3,3′ -tetraethyl-imidacarbocyanine iodide (JC-1), acridine orange (AO) and ethidium bromide (EB) were from Beyotime biotechnology company (Jiangsu, China). RPMI-1640 medium modified, penicillin-streptomycin solution and BCA protein kit were obtained from Thermo Fisher (Shanghai, China).
The total triglyceride (TG), total cholesterol (TC), alanine aminotransferase (ALT), aspartate amino-transferase (AST), total superoxide dismutase (T-SOD), glutathione peroxidase (GSH-px), hydrogen peroxide (H2O2) and malondialdehyde (MDA) assay kits were from the Nanjing Jiancheng Bioengineering Institute (Nanjing, Jiangsu, China). Polyclonal antibodies specific to Bcl-2 (SC-492), Bax (SC-493), iNOS (SC-8310), Cytochrome-C (SC-7159), IL-1β (16806-1-AP) and IL-18 (SC-6179) were purchased from Santa Cruz Biotechnology (Santa Cruz, USA). Cleaved-Caspase-3 (p17) (BS7004), Pro-Caspase-1 (EPR16883) and GAPDH (BA2913) were obtained from Bioworld Technology, Inc. (Louis Park, MN, USA). Poly (ADP-ribose) polymerase (PARP) (9542), Cox-2 (4842), LC3A/B (4108), Beclin-1 (3738) and Cleaved-Caspase-1 (4199) were purchased from Cell Signaling Technology (Shanghai, China).
2.2. Cell culture
HL-7702 cells were purchased from the Type Culture Collection of the Chinese Academy of Sciences (Shanghai, China). HL-7702 cells were cultured in RPMI-1640 medium with 10% fetal bovine serum (FBS) and 1% penicillin-streptomycin, at 37°C in a humidified incubator (5% CO2, 95% air), respectively.
2.3. Animal care and treatments
Four-week-old male BALB/c mice, weighing 15–20 g, were provided by the Experimental Animal Center of Xi’an Jiaotong University (Xi’an, Shaanxi, China). All animals were housed in air-conditioned quarters with 12 h alternating light-dark cycle. The mice were fed a standard diet, provided with food and water ad libitum. After acclimatizing to the laboratory conditions for one work, 52 mice were randomly divided into four groups. In the BaP group, mice received 50 mg/kg·bw (10 mL/kg·bw) of BaP (dissolved in the olive oil) by intra-gastricl gavaging once daily (Malik, Williams, Lemieux, White, & Yauk, Citation2012). In the ISO group, the mice were administered ISO at 20 mg/kg·bw (10 mL/kg·bw) according to the previous report and experiment (Yuan et al., Citation2016) which dissolved in the sodium carboxymethyl cellulose by intra-gastric gavaging once daily. In the ISO + BaP group, mice firstly were allowed access to ISO at a volume of 20 mg/kg·bw, and then were gavaged with BaP at 50 mg/kg·bw (10 mL/kg·bw), respectively. For the control group, mice were given the same volume of vehicle by intra-gastric gavaging. The body weights of all of the mice were measured once daily. After six weeks of administration, mice were allowed free access to water for 8 h but no food. Then, all of mice were anesthetized by ether, weighed, and sacrificed to obtain blood and liver samples. The blood samples were centrifuged at 1200 rpm for 20 min to obtain serum and stored at 4°C, the liver samples were frozen at −80°C.
All the experimental procedures conformed to the Guide for the Care and Use of Laboratory Animals (Eighth Edition, ISBN-10: 0-309-15396-4).
2.4. Cell viability by MTT and AO-EB staining assay
HL-7702 cells were individually exposed in different treatments, including 25 μM BaP, 5 μM ISO and 25 μM BaP plus 5 μM ISO. Subsequently, MTT was added to assay cell viability. Absorbance at 490 nm was measured with a microplate reader (Thermo Fisher, US.). Additionally, the cell mortality rate was analyzed by trypan blue exclusion assay (0.04%, 10 min).
The morphology of apoptotic cells was determined by AO-EB stain. After incubation, cells were stained by AO-EB (1 μg/ml AO and 1 μg/ml EB) at 37°C for 15 min, then were cleaned by PBS and observed using a fluorescence microscopy (Olympus Optical Co., Ltd., Tokyo, Japan).
2.5. Measurement of intracellular ROS and mitochondrial membrane potential (MMP)
Intracellular ROS was measured by H2DCFDA. Briefly, after different treatment, cells were stained by 1 mL10 μM H2DCFDA per well at 37°C with 5% (v/v) CO2 for 30 min. Subsequently, the fluorescence of DCF was detected by the inverted fluorescence microscope (Olympus Optical Co., Ltd., Tokyo, Japan).
Cells were cultured with different treatment. After 24 h, 100 μL of 5 μg/mL JC-1 was added to the cells for 1 h. Finally, cells were washed twice with PBS, and were observed in a fluorescence microscope (Olympus Optical Co., Ltd., Tokyo, Japan).
2.6. AO and MDC staining assay
The level of autophagy was surveyed by AO (1 μg/ml AO at 37°C for 15 min) and MDC (50 m/L MDC at 37°C for 1 h) fluorescence staining, respectively. After incubation, cells were observed using a fluorescence microscopy (Olympus Optical Co., Ltd., Tokyo, Japan).
2.7. Relative electrical conductivity assay
The relative electrical conductivity was determined by DDSJ-308A conductivity metre (Shanghai precision scientific instrument co., Ltd, China). After treatments, the culture supernatants liquid was collected and examined by conductivity metre and the tri-distilled was prepared to zero the conductivity metre.
2.8. LDH release assay
LDH is used to indicate the irreversible cell death due to cell membrane damage and to determine cellular permeability. LDH in the culture supernatants was measured using LDH kit (Nanjing Jiancheng Bioengineering Institute, China), and the absorbance was read at the wavelength of 450 nm. The results were expressed as the percentage of LDH leakage versus control cells.
2.9. Assay for NO levels
Serous and intracellular NO were determined by the Griess reagent. After treatments, samples were incubated with the same volume of the Griess reagent (0.1% (w/v) N-(1-naphathyl)-ethylenediamine and 1% (w/v) sulfanilamide in 5% (v/v) phosphoric acid) at 37°C to avoid light reaction for 10 min. Then, measured absorbance of the reaction solution at 540 nm using a Microplate Reader Instrument (Thermo Fisher, USA). NO was calculated using a sodium nitrites standard curve.
2.10. Real-time RT-PCR assessment
RT-PCR was performed to test the mRNA expression of LC3 II, Beclin-1 and the release of inflammatory factors IL-1β and IL-18. Briefly, total RNA was extracted from liver tissues and 500 ng of RNA in each group was reversely transcribed into cDNA using oligo dT and superscriptIII reverse transcriptase following the manufacturer’s instructions. RT-PCR was tested on a 7500 Fast Real-time PCR System (Applied Biosystems, Thermo Fisher Scientific, USA). Expression of mRNA was controlized by a reference GAPDH. The PCR mixtures were pre-heated at 50°C for 2 min and then at 95°C for 10 min to activate the Ampli Taq Gold DNA polymerse, then were subjected to 40 cycles of amplification (denaturation at 94°C for 15 s, annealing at 60°C for 30 s, and extension at 72°C for 30 s). Finally, the PCR reaction was extended for 10 min at 60°C. Reactions were performed in triplicate and the results were plotted, and the ΔΔCt method was used to calculate relative expression of mRNA. PCR primer sequences were shown in the .
Table 1. Primer sequences used for RT-PCR.
2.11. Protein extraction and western blot analysis
Samples were homogenized with lysis buffer (Beyotime, Jiangsu, China) containing 1% PMSF. Homogenates were centrifuged at 15,000 rpm for 15 min at 4°C. Protein concentrations in homogenates were measured using BCA kits. Protein samples were mixed with loading buffer and heated at 100°C for 10 min, then subjected to SDS-PAGE, transferred onto polyvinylidene difluoride membrane using a semi-dry electroblotting system (Bio-Rad, USA) and blocked with 5% non-fat dry milk at room temperature for 2 h. After a washing procedure, the membranes were incubated overnight with primary specific antibodies (1:1000) at 4°C, followed by incubation with horseradish peroxidase-conjugated second antibody (1:2000) for additional 2 h at 37°C. Immunoreactive signals were detected using an ECL kit (Pioneer Biotechnolgy, China) and visualized on Chemi. Doc. XRS Imaging System (UVP, USA). The bands were quantified using the Quantity One analysis software (UVP, USA).
2.12. Evaluation of liver function
The levels of total glycerin (TG), total cholesterol (TC), alanine aminotransferase (ALT), and aspartate aminotransferase (AST) in serum were determined using commercial kits (Nanjing Jiancheng Bio-engineering Institute, Nanjing, China) according to the manufacturer’s instructions.
2.13. Evaluation of antioxidant properties of liver
The liver samples obtained from each mouse were homogenized at 3700 rpm for 10 min at 4°C using a DY89-II homogenizer (Ningbo Scientz Biotechnology, China), then the supernatants were collected. The protein concentrations in supernatants were measured using the BCA protein assay kit and BSA was used as the standard protein. The activities of T-SOD and GSH-px and the levels of H2O2 and MDA in liver supernatants were determined using commercial kits according to the manufacturer’s instructions.
2.14. Histopathology
The liver tissue was fixed with 10% phosphate-buffered formaldehyde and embedded in paraffin. Sections were prepared and stained with hematoxylin and eosin. The histologic images (50 μm original magnification) were captured using a Motic BA400 microscope (Motic Co., Ltd., Causeway Bay, HongKong, China).
2.15. Observation of autophagic vacuoles in liver
Liver tissues were fixed with 2.5% glutaraldehyde for 24 h, then embedded in 2% agarose gel, and post-fixed in 4% osmium tetroxide solution for 1 h. Next, the examples were dehydrated using a series of graded ethyl alcohols from 70% to 100%, and embedded in epoxy resin at 30°C for 24 h and 60°C for 48 h, respectively. Finally, ultrathin sections were stained with uranyl acetate and lead citrate before examination using the transmission electron microscope (Hitachi, Japan).
2.16. Statistical analysis
All results were presented as the means ± standard deviation (SD), and were analyzed using the one-way factorial analysis of variance (ANOVA) and Duncan’s post hoc test (SPSS v19.0).
3. Results
3.1. Effects of ISO on cell viability in HL-7702 cells exposed to BaP
BaP significantly (p < 0.01) inhibited cell growth to 71.4%, and an obvious increase (144.9%) in cell viability was found in ISO-treated alone cells. Pretreatment with ISO could notably (p < 0.05) increase cell viability by 20.7%, compared with BaP group ((A)). Trypan blue staining assay also showed that the number of cells dyed blue was notably increased after BaP treatment and observably decreased after treatment with ISO and BaP simultaneously ((B)). All the results suggested that ISO could prevent the decrease in cell viability in HL-7702 cells exposed to BaP.
Figure 2. Effects of ISO on BaP-induced injury in HL-7702 cells. Cells viability (A), the results are shown as the means ± SD of nine separate experiments. **p < 0.01 and *p < 0.05 versus control treatment, ##p < 0.01 and #p < 0.05 versus BaP treatment. Trypan blue staining (B), arrows indicate dead cells.
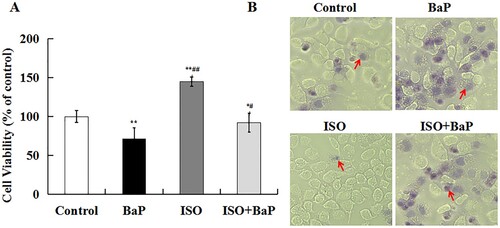
3.2. Effects of ISO on BaP-induced autophagic and pyroptotic injury in HL-7702 cells
As shown in (A), the more red fluorescence, the more the number of autophagic vacuoles in AO fluorescence staining. The considerably red fluorescence in BaP-treated cells was observed, and ISO weakened the red fluorescence intensity motivated by BaP, indicating that ISO inhibited the formation of autophagic vacuoles induced by BaP.
Figure 3. Effects of ISO on the autophagic injury in HL-7702 cells caused by BaP. The red fluorescence and bright green fluorescence indicate the autophagic vacuoles (A), Scale bar = 50 μm. Beclin-1 and LC3 I/II were assessed by western blot analysis, GAPDH was used as an internal control (B).
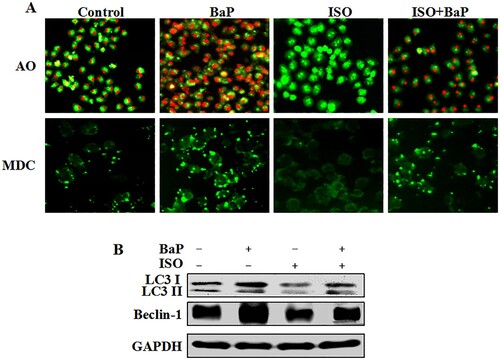
In MDC fluorescence staining, the more green fluorescence, the more number of the vacuoles. There was no significant difference in cells treated with ISO alone compared to control group, ISO pretreatment could obviously decrease the green fluorescence intensity, reverse the effects of BaP on the vacuoles formation. Furthermore, the conspicuous reduced expression of LC3 II and Beclin-1 were found in ISO-treated cells, compared with control group. And ISO pretreatment also decreased the overexpression of LC3 II and Beclin-1, compared with an apparently increased expression in BaP-treated cells ((B)). On the whole, these results suggested that ISO could relieve autophagic cell death induced by BaP in HL-7702 cells.
The level of the electrical conductivity and the release of LDH and NO were regarded as indicators of cell pyroptosis. As shown in , BaP markedly (p < 0.01) increased the levels of the electrical conductivity, LDH and NO in HL-7702 cells. In detail, compared with control group, BaP increased the electrical conductivities by 31.93 ms/cm. And pretreatment with ISO could decrease it by 16.87 ms/cm comparing with BaP group ((A)). The release of LDH in BaP-treated cells was 1.98 times as much as it in the control group, and pretreatment with ISO could be decreased by 40.40% compared with BaP treatment ((B)). Besides, the level of NO in BaP-treated cells was increased by 43 μm compared with control group, and pretreatment with ISO could be decreased by 23 μm compared with BaP treatment ((C)). These results showed that ISO could weaken BaP-induced pyroptotic cell death.
Figure 4. Effects of ISO on the pyroptotic injury in HL-7702 cells induced by BaP. The culture supernatant was used to access electrical conductivity (A), LDH release (B), NO (C). The results are shown as the means ± SD of nine separate experiments. **p < 0.01 versus control treatment, and #p < 0.05 and ##p < 0.01 versus BaP treatment. Cell pellet was to examine the pyroptotic marker proteins (Pro-Caspase-1, Cleaved-Caspase-1, iNOS, Cox-2, IL-1β, IL-18) by western blot (D).
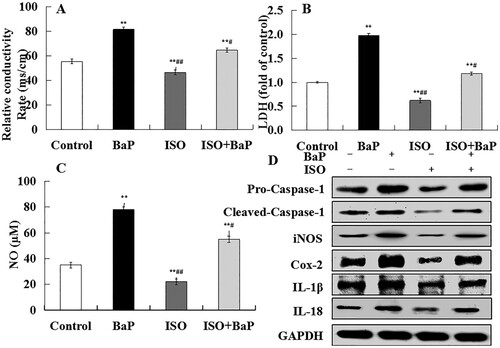
Additionally, pyroptosis is uniquely dependent on Caspases-1 activation and inflammatory factors release. The marked increase in the proteins expression of Pro-Caspase-1, Cleaved-Caspase-1, iNOS, Cox-2, IL-1β and IL-18 were found in BaP-treated cells ((D)), and ISO obviously reversed the enhanced effects of BaP on these proteins expression. All the results suggested that ISO was able to protect HL-7702 cells against pyroptotic cell death caused by BaP.
3.3. Effects of ISO on BaP-induced apoptotic injury in HL-7702 cells
Furthermore, our results found that BaP significantly increased the number of apoptotic cells, stimulated the intracellular ROS production and cut down MMP, whereas ISO markedly reversed the adverse effect of BaP on them ((A)). Meanwhile, a decrease in Bcl-2 protein expression and an increase in Bax, Cyto-C, Cleaved-Caspase-3 and PARP proteins expression were found in BaP-treated cells. Compared with BaP treatment, the significant elevation of Bcl-2 expression and reduction of Bax, Cyto-C, Cleaved-Caspase-3 and PARP expression are indicated in ISO alone or/and BaP simultaneously treated cells ((B)). From the above, the BaP-induced apoptotic cell injury in HL-7702 cells could be relieved by ISO.
Figure 5. Effects of ISO on BaP- induced apoptotic injury in HL-7702 cells. Cells were observed by fluorescent microscopy (A). In AO-EB experiments, green cells were counted as live cells; yellow cells were early apoptotic cells; orange cells were late apoptotic cells; and red cells were dead cells. In JC-1 staining experiments, red fluorescence represents the intact MMP and green fluorescence represents the dissipation of MMP. In ROS experiments, the stronger the fluorescence, the more reactive oxygen is released. Scale bar = 50 μm. The pyroptotic marker proteins (Bcl-2, Bax, Cyto-C, Cleaved-Caspase-3, PARP) were determined by western blot, and GAPDH was used as an internal control (B).
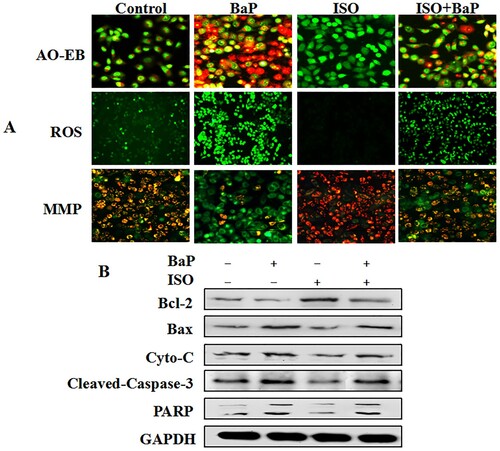
3.4. Effects of ISO on body weight, food intake and liver index
As shown in , there were no significant differences in the initial body weight among all the groups. After 6 weeks trial, compared with the control group, BaP effectively (p < 0.05) reduced the final body weight by 3.36 ± 1.54 g, and the final body weight of the ISO-treated mice was much (p < 0.05) higher than that of the control group. And simultaneous treatment of ISO and BaP could alleviate the final body weight loss after exposure to BaP, though there was no obvious change.
Table 2. Effects of ISO on body weight, food intake and liver index of BaP-treated mice.
The ADG and ADFI of BaP-treated mice were much (p < 0.05) lower than that of control group. The pretreatment with ISO effectively increased the ADFI (p < 0.05), compared with BaP group. As for the liver index, there was a certain degree of increase in BaP group as compared with the control group, and ISO pretreatment attenuated the BaP-induced elevation of the liver index. All of these results showed that ISO was contributed to decrease the liver index, increase the body weight gain and the food intake of the BaP-exposed mice.
3.5. Effects of ISO on liver function of mice exposed to BaP
In order to determine the effects of ISO on BaP-induced the liver dysfunction, the levels of TC, TG and the activities of AST and ALT in serum of mice were measured. As shown in (A) and (B), no apparent differences were observed in the serum levels of TC and TG among control group, BaP group, and ISO + BaP group. The levels of AST (p < 0.01) and ALT (p < 0.01) in BaP group were markedly higher than that of control group. And the simultaneous treatment of ISO and BaP slightly suppressed the BaP-induced increase in the level of AST ((C)), and effectively (p < 0.05) lowered the level of ALT ((D)). These results indicated that ISO could attenuate liver dysfunction induced by BaP.
Figure 6. Effects of ISO on liver function of mice exposed to BaP. Levels of TC (A), TG (B), AST (C) and ALT (D) in serum were measured to reflect the liver function after exposure with BaP or/and ISO. The results are shown as the means ± SD of thirteen separate experiments. **p < 0.01 and *p < 0.05 are compared with the control treatment, ##p < 0.01and #p < 0.05, as compared with BaP treatment.
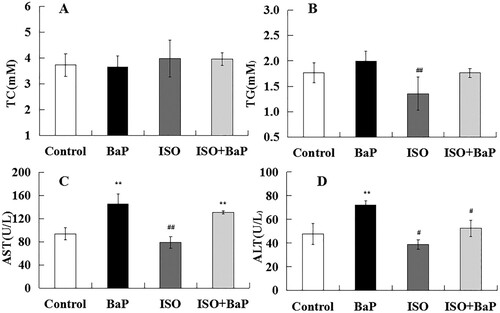
3.6. Effects of ISO on antioxidant properties of liver in BaP-treated mice
Compared with control group, the activities of T-SOD and GSH-px in liver were markedly (p < 0.01) lowered by the exposure of BaP, and the notable increase of them in the ISO group were observed ((A), (B)). Compared with BaP group, ISO reversed the inhibitive effects of BaP on the activities of T-SOD and GSH-px.
Figure 7. Effects of ISO on the antioxidant properties of mice exposed to BaP. The activities of antioxidant enzyme T-SOD (A), GSH-px (B) and the levels of oxidation products MDA (C) and H2O2 (D). The results are shown as the means ± SD of thirteen separate experiments. **p < 0.01 and *p < 0.05 are compared with the control treatment, ##p < 0.01 and #p < 0.05 are compared with BaP treatment.
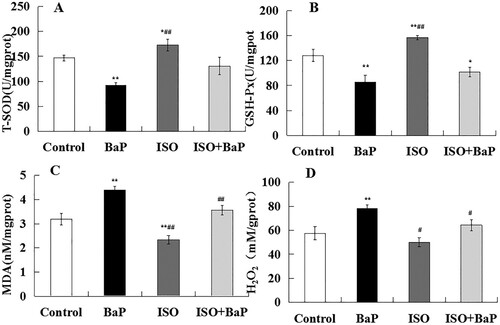
MDA is a biomarker of oxidative stress as the end product of lipid peroxidation. H2O2 is one of ROS. An obvious (p < 0.01) increase in the levels of MDA ((C)) and H2O2 ((D)) was observed in BaP group, compared with the control group. ISO supplementation effectively (p < 0.01) prevented the BaP-caused up-regulation on these levels in liver. These results suggested that ISO could alleviate BaP-induced liver oxidative injury in mice.
3.7. Histopathology of liver
To further confirm the effects of ISO on BaP-induced hepatic injury, the histopathology of liver tissue was performed with H&E staining. As shown in , the liver sections in BaP-treated mice showed the obvious liver damage, which is manifested as liver cell cord disorder, a significant decrease in the number of hepatocyte nucleus and an obvious increase in the interval between cells, compared with the control mice. ISO pretreatment effectively improved the BaP-induced liver tissue injury, subsequently increased the number of hepatocyte nucleus and narrowed the interval between cells. In short, the BaP-induced liver tissue injury could be attenuated by the ISO treatment.
3.8. Effects of ISO on BaP-induced autophagic and pyroptotic injury in liver
In order to further investigate whether ISO could alleviate BaP-induced liver injury by affecting autophagy and pyroptosis in vivo, the TEM ultrastructure of liver tissue was observed. As shown in (A), compared with the control group, autophagic vacuoles were observed more frequently in liver tissue in the BaP-treated group, which were different shaped double membrane-surrounded structure, and had enveloped some cytoplasm and organelles. ISO supplementation effectively inhibited the excessive increase in the number of the autophagic vacuoles caused by BaP. Meanwhile, as the characteristic factors of autophagy, the mRNA levels of LC3 II ((B)) and Beclin-1 ((C)) were increased by 21.5% and 37.4% after BaP treatment, respectively, compared with the control group. ISO treatment markedly (p < 0.01) inhibited the increase of Beclin-1 induced by BaP, as compared with BaP group. These results indicated that ISO could inhibit BaP-induced autophagic liver injury of mice.
Figure 9. Effects of ISO on the autophagic injury of liver in mice exposed to BaP. TEM shows the ultrastructure of liver tissue treated with BaP and ISO. Arrows indicate autophagosomes including residual digested material (A), the mRNA levels of LC3 II (B) and Beclin-1 (C). *p < 0.05 as compared with the control group, ##p < 0.01 and #p < 0.05 are compared with BaP group.
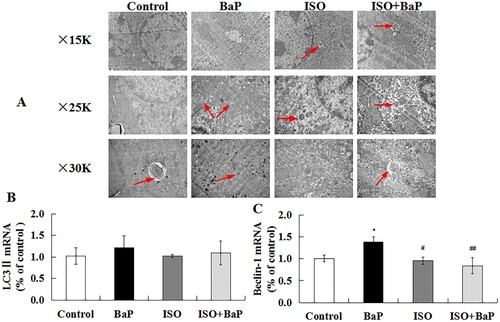
Caspase-1, NO, IL-1β and IL-18 are the characteristic indices of pyroptosis. As shown in (A) and (B), the increased mRNA levels of IL-1β and IL-18 (p < 0.01) were observed in BaP group, compared with the control group. Furthermore, simultaneous treatment of ISO and BaP reduced the mRNA levels of IL-1β (by 31.6%) and IL-18, when compared with BaP group. The NO level was significantly increased (p < 0.01) after BaP treatment ((C)), and ISO pretreatment markedly (p < 0.01) reduced it. (D) showed that BaP increased the proteins expression of Cleaved-Caspase-1 and the ration of Cleaved-Caspase-1 to pro-Caspase-1, and ISO pretreatment decreased this expression of them (p < 0.05).. Taken together, these results showed that ISO could diminish the BaP-induced pyroptotic injury in mice.
Figure 10. Effects of ISO on the pyroptotic injury of liver in mice exposed to BaP. The mRNA levels of IL-1β (A) and IL-18 (B), the level of NO (C) and the proteins expression of pro-Caspase-1, Cleaved-Caspase-1, the ration of Cleaved-Caspase-1 to pro-Caspase-1(D), **p < 0.01 and *p < 0.05, as compared with the control group, ##p < 0.01 and #p < 0.05, as compared with BaP group.
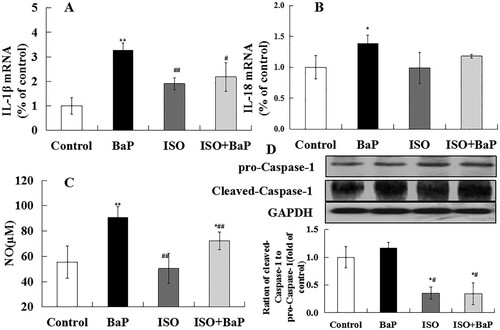
4. Discussion
BaP is widely produced in the high-temperature processed foods due to incomplete combustion of the protein, carbohydrates and lipids, and it results in a serious threat to human health. Some plant flavonoids compound like laricitrin (Chang et al., Citation2016), chrysin (Kasala et al., Citation2016) and quercetin (Günay et al., Citation2016) could protect the body tissue from damage caused by BaP. ISO is a natural flavonoid compound and possesses the high hepatoprotective activity (Lin et al., Citation2015; Yuan, Li, et al., Citation2018). This work firstly revealed that ISO could relieve BaP-caused apoptotic, autophagic and pyroptotic liver injury in vitro through decreasing the number of autophagic vacuoles, the levels of LC3 II and Beclin-1, and the proteins expression of Cleaved-Caspase-1, IL-1β and IL-18. Consistent with in vitro studies, ISO could attenuate BaP-induced liver injury by reducing the levels of ALT and AST to improve liver function, up-regulating the activities of T-SOD and GSH-px, and down-regulating the levels of MDA and H2O2 to enhance the antioxidant defense system in vivo. Furthermore, ISO could also effectively promote the ADFI, increase the body weight, and decrease the liver index to attenuate liver injury.
Autophagy is a stress reaction that arises when cells resist the undesirable external stimulus. The excessive autophagy impairs the morphology and functions of cells (Cui, Liu, & Zhang, Citation2018; Zhang et al., Citation2019) and results in the autophagic cell death (Sergi & Jordi, Citation2018). BaP could induce the liver dysfunction by increasing the appearance of vesicles containing degraded cellular material (Gorria et al., Citation2008). LC3 II and Beclin-1 are associated with the formation of autophagosome (Klionsky et al., Citation2016). Our previous study found that BaP could trigger the autophagic death in HL-7702 cells through promoting the formation of autophagic vacuoles and enhancing the proteins expression of Beclin-1 and LC3 II (Yuan et al., Citation2017). This study found that ISO could reduce the formation of autophagic vacuoles and down-regulate the overexpression of Beclin-1 and LC3 II after exposure to BaP in vitro and vivo ( and ), indicating that ISO could relieve autophagic injury induced by BaP in vitro and vivo.
Pyroptosis is a recently discovered type of the programmed cell death triggered by various pathologies, such as virus (Yuan, Zhu, et al., Citation2018), acinetobacter baumannii (Dai et al., Citation2018), and gossypol (Lin et al., Citation2016), which depends on the activation of Caspase-1, the release of a large number of pro-inflammatory cytokines (IL-1β, IL-18), the loss of cell membrane integrity and the permeability of cells, and the increase in conductivity (Cookson & Brennan, Citation2001; Fernandes-Alnemri et al., Citation2007). Carbon black as an organic material could induce pyroptosis (Reisetter, Stebounova, Grassian, & Monick, Citation2011). CdSe/ZnS, the heavy metal toxicants, could induce pyroptotic cell death in L02 human hepatic cells through inhibiting cell viability, motivating ROS release and inflammatory cytokine expression (Pro-Caspase-1, Caspase-1 and IL-1β) (Lu et al., Citation2016). Chlorpyrifos as a pesticide could increase the release level of LDH and the overexpression of Caspase-1 and induce pyroptosis in HaCaT human keratinocyte cells (Jang et al., Citation2015). It has reported that BaP results in increase in NO level and iNOS protein expression (Hardonnière et al., Citation2015). NO is a pro-inflammatory factors and a signal of pyroptotic cell death. Consistent with these studies, our previous study also found that BaP could induce pyroptotic cell death in HL-7702 human hepatocyte cells (Yuan et al., Citation2017). The present study showed that ISO could weaken pyroptotic injury caused by BaP in vitro and vivo through decreasing the levels of the electrical conductivity, LDH and NO, inhibiting the proteins expression of Pro-Caspase-1, Cleaved-Caspase-1, iNOS, Cox-2 and the mRNA levels of IL-1β and IL-18 ( and ).
Furthermore, the present study also found that ISO could effectively weaken the apoptotic injury caused by BaP. Numerous studies showed that BaP could result in cell death through inducing apoptosis (Chang, Lin, Chan, & Yeh, Citation2012; Kim, Lee, Hwang, & Choi, Citation2017; Qin, Wu, & Nan, Citation2015). Quercetin decreased the level of ROS and protected A549 cells from BaP-induced injury (Das et al., Citation2014). Apigenin could relieve BaP-induced apoptotic cell death through decreasing ROS accumulation, ameliorating MMP change, down-regulating proteins expression of Bax, Cyto-C and Cleaved- Caspase-3 and up-regulating Bcl-2 protein expression (Chang et al., Citation2012). We found that ISO also markedly reversed the BaP-induced apoptotic injury by decreasing the number of apoptotic cells, ROS levels, MMP loss and the proteins expression of Bax, Cyto-C, Cleaved-Caspase-3 and PARP ().
Liver damage could cause lipid metabolism dysfunction, subsequently result in a large accumulation of lipid in serum, and the alterations in blood lipid profile (TC, TG) (Miller et al., Citation2011). AST and ALT are considered as the effective biochemical markers for early hepatic damage (Zhang, Chen, Sun, & Zhao, Citation2014). In agreement with these studies, ISO pretreatment reversed the BaP-induced liver dysfunction through reducing the activities of ALT and AST ().
BaP could produce a variety of ROS during the metabolism, the excessive ROS in the body would be involved in the pathogenesis of liver tissue and cause changes in the antioxidase activities (Debnath, Kim, Das, Nath, & Lee, Citation2019). MDA is the product of lipid peroxidation (Zhuang et al., Citation2016), H2O2 is produced as a natural byproduct of the normal of oxygen and possesses strong oxidizing properties and causes the deleterious physiological effects (Debnath et al., Citation2018). It has reported that EGCG inhibited the BaP-induced oxidative damage by increasing the enzymes activities of T-SOD and GSH-px and reducing the level of MDA (Rangi, Dhatwalia, Bhardwaj, Kumar, & Dhawan, Citation2018). In the present investigation, we found that ISO overturned the reduction of activities of T-SOD and GSH-px and down-regulated the levels of MDA and H2O2 induced by BaP (). Thus, our study provided the evidence that ISO could regulate oxidative injury of liver caused by BaP in vivo.
Additionally, BaP could cause slow weight gain and reduce the food intake (Briede et al., Citation2004), consistent with the previous study, the lower body weight and food intake were observed in the BaP group in this study (). And ISO could relieve BaP-resulted the decrease in body weight, food intake and the increase in liver index, maybe because the emergence of cancer cells (Garry, Nesslany, & Aliouat, Citation2003; Liu et al., Citation2018). Histopathological observations of liver further showed that ISO could alleviate the liver injury induced by BaP.
5. Conclusion
In conclusion, this work showed that the important role of ISO in weakening liver injury induced by BaP in vitro and vivo. In addition, ISO could enhance antioxidant system, relieve oxidative injury, improve the liver function, increase the body weight, and decrease the liver index to prevent BaP-induced liver injury. Thus, it is likely that ISO could be served as an effective approach and a new available food supplement to terminate the liver injury induced by BaP.
Disclosure statement
No potential conflict of interest was reported by the authors.
Additional information
Funding
References
- Adrian, T., Manuel, G., Jesus, M., & Mıguez, J. (2008). Soengas.b-Naphthoflavone and benzo(a)pyrene treatment affect liver intermediary metabolism and plasma cortisol levels in rainbow trout oncorhynchus mykiss. Ecotoxicology and Environmental Safety, 69, 180–186. doi: 10.1016/j.ecoenv.2007.03.009
- Ali, R., Shahid, A., Ali, N., Hasan, S. K., Majed, F., & Sultana, S. (2016). Amelioration of benzo[a]pyrene-induced oxidative stress and pulmonary toxicity by naringenin in wistar rats: A plausible role of Cox-2 and NF-κB. Human & Experimental Toxicology, 36, 349–364. doi: 10.1177/0960327116650009
- Anilkumar, K., Reddy, G. V., Azad, R., Yarla, N. S., Dharmapuri, G., Srivastava, A., … Pallu, R. (2017). Evaluation of anti-inflammatory properties of isoorientin isolated from tubers of Pueraria tuberosa. Oxidative Medicine Cellular Longevity. doi: 10.1155/2017/5498054
- Bengtsson, M., Montelius, J., Mankowitz, L., & Rydstrom, J. (1983). Metabolism of polycyclic aromatic hydrocarbons in the rat ovary comparison with metabolism in adrenal and liver tissues. Biochemical Pharmacology, 32, 129–136. doi: 10.1016/0006-2952(83)90664-0
- Briede, J. J., Godschalk, R. W. L., Emans, M. T. G., De Kok, T. M. C. M., Van Agen, E., Van Maanen, J., … Kleinjans, J. C. (2004). In vitro and in vivo studies on oxygen free radical and DNA adduct formation in rat lung and liver during benzo (a)pyrene metabolism. Free Radical Research, 38, 995–1002. doi: 10.1080/10715760400000976
- Chang, W. A., Hung, J. Y., Tsai, Y. M., Hsu, Y. L., Chiang, H. H., Chou, S. H., … Kuo, P. L. (2016). Laricitrin suppresses increased benzo(a)pyrene-induced lung tumor-associated monocyte-derived dendritic cell cancer progression. Oncology Letters, 11, 1783–1790. doi: 10.3892/ol.2016.4153
- Chang, Y. Z., Lin, H. C., Chan, S. T., & Yeh, S. L. (2012). Effects of quercetin metabolites on the enhancing effect of β-carotene on DNA damage and cytochrome P1A1/2 expression in benzo[a]pyrene-exposed A549 cells. Food Chemistry, 133, 445–450. doi: 10.1016/j.foodchem.2012.01.060
- Chen, H., Song, Q., Diao, X., & Zhou, H. (2016). Proteomic and metabolomic analysis on the toxicological effects of benzo[a]pyrene in pearl oyster Pinctada martensii. Aquatic Toxicology, 175, 81–89. doi: 10.1016/j.aquatox.2016.03.012
- Chunxia, G., Wang, F., Li, Y., Liu, J., Sun, D., & Li, X. (2018). Physicochemical property, antioxidant activity, and cytoprotective effect of the germinated soybean proteins. Food Science& Nutrition, 1, 1–12. doi: 10.1002/fsn3.822
- Cookson, B. T., & Brennan, M. A. (2001). Pro-inflammatory programmed cell death. Trends in Microbiology, 9, 113–114. doi: 10.1016/S0966-842X(00)01936-3
- Cui, Y.-Q., Liu, Y.-J., & Zhang, F. (2018). The suppressive effects of Britannin (Bri) on human liver cancer through inducing apoptosis and autophagy via AMPK activation regulated by ROS. Biochemical and Biophysical Research Communications, 497, 916–923. doi: 10.1016/j.bbrc.2017.12.144
- Dai, M., Pan, P., Li, H., Liu, S., Zhang, L., Song, C., … Hu, C. (2018). The antimicrobial cathelicidin peptide hlF(1-11) attenuates alveolar macrophage pyroptosis induced by acinetobacter baumannii in vivo. Experimental Cell Research, 364, 95–103. doi: 10.1016/j.yexcr.2018.01.035
- Das, S., Das, J., Samadder, A., Paul, A., & Khuda-Bukhsh, A. R. (2013). Efficacy of PLGA-loaded apigenin nanoparticles in benzo[a]pyrene and ultraviolet-B induced skin cancer of mice: Mitochondria mediated apoptotic signalling cascades. Food and Chemical Toxicology, 62, 670–680. doi: 10.1016/j.fct.2013.09.037
- Das, D. N., Panda, P. K., Mukhopadhyay, S., Sinha, N., Mallick, B., Behera, B., … Bhutia, S. K. (2014). Prediction and validation of apoptosis through cytochrome p450 activation by benzo[a]pyrene. Chemico-Biological Interactions, 208, 8–17. doi: 10.1016/j.cbi.2013.11.005
- Debnath, T., Ghosh, M., Lee, Y. M., Nath, N. C. D., Lee, K.-G., & Lim, B. O. (2018). Identification of phenolic constituents and antioxidant activity of Aloe barbadensis flower extracts. Food and Agricultural Immunology, 29, 27–38. doi: 10.1080/09540105.2017.1358254
- Debnath, T., Kim, E.-K., Das, G., Nath, N. C. D., & Lee, K.-G. (2019). Protective effect of oat (Avena sativa) bran extracts on acute hepatic liver damage in mice. Food and Agricultural Immunology, 30, 34–46. doi: 10.1080/09540105.2018.1541169
- Fanali, Z., Franco-Belussi, L., Bonini-Domingos, C. R., & Oliveira, C. (2018). Effects of benzo[a]pyrene on the blood and liver of Physalaemus cuvieri and Leptodactylus fuscus (Anura: Leptodactylidae). Environmental Pollution, 237, 93–102. doi: 10.1016/j.envpol.2018.02.030
- Fernandes-Alnemri, T., Wu, J., Yu, J. W., Datta, P., Miller, B., Jankowski, W., … Alnemri, E. S. (2007). The pyroptosome: A supramolecular assembly of ASC dimmers mediating inflammatory cell death via caspase-1 activation. Cell Death & Differentiation, 14, 1590–1604. doi: 10.1038/sj.cdd.4402194
- Garry, S., Nesslany, F., & Aliouat, E. (2003). Hematite(Fe2O3) enhances benzo(a)pyrene genotoxicity in endotracheally treated rat, as determined by comet assay. Mutation Research, 538, 19–29. doi: 10.1016/S1383-5718(03)00082-2
- Gorria, M., Tekpli, X., Rissel, M., Sergent, O., Huc, L., Landvik, N., … Lagadicgossmann, D. (2008). A new lactoferrin-and iron-dependent lysosomal death pathway is induced by benzo[a]pyrene in hepatic epithelial cells. Toxicology and Applied Pharmacology, 228, 212–224. doi: 10.1016/j.taap.2007.12.021
- Grundy, M. M., Moore, M. N., Howell, S. M., & Ratcliffe, N. A. (1996). Phagocytic reduction and effects on lysosomal membranes by polycyclic aromatic hydrocarbons, in haemocytes of Mytilus edulis. Aquatic Toxicology, 34, 273–290. doi: 10.1016/0166-445X(95)00044-5
- Günay, E., Celik, S., Sarinc-Ulasli, S., Özyürek, A., Hazman, O., Günay, S., … Ünlü, M. (2016). Comparison of the anti-inflammatory effects of proanthocyanidin, quercetin, and damnacanthal on benzo(a)pyrene exposed A549 alveolar cell line. Inflammation, 39, 744–751. doi: 10.1007/s10753-015-0301-3
- Hardonnière, K., Huc, L., Podechard, N., Fernier, M., Tekpli, X., Gallai, I., … Laqadic-Gossmann, D. (2015). Benzo[a]pyrene-induced nitric oxide production acts as a survival signal targeting mitochondrial membrane potential. Toxicology in Vitro, 29, 1597–1608. doi: 10.1016/j.tiv.2015.06.010
- IARC. (2014). Diesel and gasoline engine exhausts and some nitroarenes. IARC Monographs on the Evaluation of Carcinogenic Risks to Humans, 105, 1–703.
- Jang, Y., Lee, A. Y., Jeong, S. H., Park, K. H., Paik, M. K., Cho, N. J., … Cho, M. H. (2015). Chlorpyrifos induces NLRP3 inflammasome and pyroptosis/apoptosis via mitochondrial oxidative stress in human keratinocyte HaCaT cells. Toxicology, 338, 37–46. doi: 10.1016/j.tox.2015.09.006
- Ji, X., Li, Y., He, J., Shah, W., Xue, X., Feng, G., … Gao, M. (2016). Depletion of mitochondrial enzyme system in liver, lung, brain, stomach and kidney induced by benzo(a)pyrene. Environmental Toxicology Pharmacology, 43, 83–93. doi: 10.1016/j.etap.2016.03.001
- Jiang, Y., Zhou, X., Chen, X., Yang, G., Wang, Q., Rao, K., … Yuan, J. (2011). Benzo(a)pyrene-induced mitochondrial dysfunction and cell death in p53-null Hep3B cells. Mutation Research, 726, 75–83. doi: 10.1016/j.mrgentox.2011.08.006
- Kasala, E. R., Bodduluru, L. N., Barua, C. C., Madhana, R. M., Dahiya, V., Budhani, M. K., … Gogoi, R. (2016). Chemopreventive effect of chrysin, a dietary flavone against benzo(a)pyrene induced lung carcinogenesis in Swiss albino mice. Pharmacological Reports, 68, 310–318. doi: 10.1016/j.pharep.2015.08.014
- Kim, S. M., Lee, H. M., Hwang, K. A., & Choi, K. C. (2017). Benzo(a)pyrene induced cell cycle arrest and apoptosis in human choriocarcinoma cancer cells through reactive oxygen species-induced endoplasmic reticulum-stress pathway. Food and Chemical Toxicology, 107, 339–348. doi: 10.1016/j.fct.2017.06.048
- Klionsky, D. J., Abdelmohsen, K., Abe, A., Abedin, M. J., Abeliovich, H., Acevedo Arozena, A., … Adeli, K. (2016). Guidelines for the use and interpretation of assays for monitoring autophagy. Autophagy, 12, 1–222. doi: 10.1080/15548627.2015.1100356
- Lee, W., Ku, S.-K., & Bae, J.-S. (2014). Vascular barrier protective effects of orientin and isoorientin in LPS-induced inflammation in vitro and in vivo. Vascular Pharmacology, 62, 3–14. doi: 10.1016/j.vph.2014.04.006
- Lin, X., Chen, Y., Lv, S., Tan, S., Zhang, S., Huang, R., … Huang, Q. (2015). Gypsophila elegans isoorientin attenuates CCl4-induced hepatic fibrosis in rats via modulation of NF-κB and TGF-β1/smad signaling pathways. International Immunopharmacology, 28, 305–312. doi: 10.1016/j.intimp.2015.06.021
- Lin, Q.-R., Li, C.-G., Zha, Q.-B., Xu, L.-H., Pan, H., Zhao, G.-X., … He, X.-H. (2016). Gossypol induces pyroptosis in mouse macrophages via a non-canonical inflammasome pathway. Toxicology and Applied Pharmacology, 292, 56–64. doi: 10.1016/j.taap.2015.12.027
- Liu, H., Pei, X., Wang, J., Zhou, Y., Wang, L., & Qi, B. (2018). Effect of loach paste on the liver and immune organs of D-galactose-induced ageing mice. Food and Agricultural Immunology, 29, 316–331. doi: 10.1080/09540105.2017.1376037
- Lu, Y., Xu, S., Chen, H., He, M., Deng, Y., Cao, Z., … Zhou, Z. (2016). Cdse/zns quantum dots induce hepatocyte pyroptosis and liver inflammation via NLRP3 inflammasome activation. Biomaterials, 90, 27–39. doi: 10.1016/j.biomaterials.2016.03.003
- Ma, J. Q., Liu, C. M., Qin, Z. H., Jiang, J. H., & Sun, Y. Z. (2011). Ganoderma applanatum terpenes protect mouse liver against benzo(a)pyren-induced oxidative stress and inflammation. Environmental Toxicology and Pharmacology, 31, 460–468. doi: 10.1016/j.etap.2011.02.007
- Malik, A. I., Williams, A., Lemieux, C. L., White, P. A., & Yauk, C. L. (2012). Hepatic mRNA, microRNA, and miR-34a-target responses in mice after 28 days exposure to doses of benzo(a)pyrene that elicit DNA damage and mutation. Environmental and Molecular Mutagenesis, 53, 10–21. doi: 10.1002/em.20668
- Marcarini, J. C., Tsuboy, M. S. F., Luiz, R. C., Ribeiro, L. R., Hoffmann-Campo, C. B., & Mantovania, M. S. (2011). Investigation of cytotoxic, apoptosis-inducing, genotoxic and protective effects of the flavonoid rutin in HTC hepatic cells. Experimental and Toxicologic Pathology, 63, 459–465. doi: 10.1016/j.etp.2010.03.005
- Miller, K., & Ramos, K. (2001). Impact of cellular metabolism on the biological effects of benzo[a]pyrene and related hydrocarbons. Drug Metabolism Reviews, 33, 1–35. doi: 10.1081/DMR-100000138
- Miller, M., Stone, N. J., Ballantyne, C., Bittner, V., Criqui, M. H., & Ginsberg, H. N. (2011). Triglycerides and cardiovascular disease: A scientific statement from the American Heart Association. Circulation, 123, 2292–2333. doi:10.1016/CIR.0b013e3182160726 doi: 10.1161/CIR.0b013e3182160726
- Perumal, V. K., Muruganantham, S., Subramanian, M., Shunmugiah, K. P., & Kasi, P. D. (2012). Silymarin attenuates benzo(a)pyrene induced toxicity by mitigating ROS production, DNA damage and calcium mediated apoptosis in peripheral blood mononuclear cells (pbmc). Ecotoxicology and Environmental Safety, 86, 79–85. doi: 10.1016/j.ecoenv.2012.08.031
- Phillips, D. H. (1999). Polycyclic aromatic hydrocarbons in the diet. Mutation Research, 443, 139–147. doi: 10.1016/S1383-5742(99)00016-2
- Qin, G., Wu, M., & Nan, S. (2015). Sulfur dioxide and benzo(a)pyrene trigger apoptotic and anti-apoptotic signals at different post-exposure times in mouse liver. Chemosphere, 139, 318–325. doi: 10.1016/j.chemosphere.2015.06.052
- Rangi, S., Dhatwalia, S. K., Bhardwaj, P., Kumar, M., & Dhawan, D. K. (2018). Evidence of similar protective effects afforded by white tea and its active component EGCG on oxidative-stress mediated hepatic dysfunction during benzo(a)pyrene induced toxicity. Food and Chemical Toxicology, 116, 281–291. doi: 10.1016/j.fct.2018.04.044
- Reisetter, A., Stebounova, L., Grassian, V., & Monick, M. M. (2011). Induction of inflammasome-dependent pyroptosis by carbon black nanoparticles. Journal of Biological Chemistry, 38, 286.
- Sadikovic, B., Andrew, J., Carter, D., Robinson, J., Rodenhiser, D. I. (2008). Genome-wide H3K9 histone acetylation profiles are altered in benzopyrene-treated MCF7 breast cancer cells. Journal of Biological Chemistry, 283, 4051–4060. doi: 10.1074/jbc.M707506200
- Sergi, G., & Jordi, G. (2018). Autophagy and cardometabolic diseases. doi: 10.1016/B978-0-12-805253-2.00017-1
- Sobinoff, A. P., Pye, V., Nixon, B., Roman, S. D., & Mclaughlin, E. A. (2012). Jumping the gun: Smoking constituent bap causes premature primordial follicle activation and impairs oocyte fusibility through oxidative stress. Toxicology and Applied Pharmacology, 260, 70–80. doi: 10.1016/j.taap.2012.01.028
- Toda, K., Shoketsu, H., Shogo, T., Norihito, S., & Hiroshi, S. (2017). Passionflower extract induces high-amplitude phythms without phase shifts in the expression of several circadian clock genes in vitro and in vivo. International Journal for Biomedical Science, 13, 84–92.
- Ueng, Y.-F., Shyu, C.-C., Liu, T.-Y., Oda, Y., Lin, Y.-L., Liao, J.-F., & Chen, C.-F. (2001). Protective effects of baicalein and wogonin against benzo[a]pyreneand aflatoxin B1-induced genotoxicities. Biochemical Pharmacology, 62, 1653–1660. doi: 10.1016/S0006-2952(01)00816-4
- Watanabe, M., & Ito, M. (2002). Changes in antioxidative activity and flavonoid composition of the extracts from aerial parts of buckwheat during growth period. Nippon Shokuhin Kagaku Kogaku Kaishi, 49, 119–125. doi: 10.3136/nskkk.49.119
- Weiping, W., Rong, W., Qiao, Z., Gil, M., & Huidong, Z. (2018). Benzo(a)pyren-7,8 -dihydrodiol-9,10-epoxide induces human trophoblast Swan 71 cell dysfunctions due to cell apoptosis through disorder of mitochondrial fission/fusion. Environmental Pollution, 233, 820–832. doi: 10.1016/j.envpol.2017.11.022
- Widstrom, N. W., & Snook, M. E. (1998). A gene controlling biosynthesis of isoorientin, a compound in corn silks antibiotic to the corn earworm. Entomologia Experimentalis et Applicata, 89, 119–124. doi: 10.1046/j.1570-7458.1998.00390.x
- Yuan, L., Han, X., Li, W., Ren, D., & Yang, X. (2016). Isoorientin prevents hyperlipidemia and liver injury by regulating lipid metabolism, antioxidant capability, and inflammatory cytokine release in high-fructose-fed mice. Journal of Agricultural and Food Chemistry, 64, 2682–2689. doi: 10.1021/acs.jafc.6b00290
- Yuan, L., Li, X., He, S., Gao, C., Wang, C., & Shao, Y. (2018). Effects of natural flavonoid isoorientin on growth performance and gut microbiota of mice. Journal of Agricultural and Food Chemistry, 66, 9777–9784. doi: 10.1021/acs.jafc.8b03568
- Yuan, L., Liu, J., Deng, H., & Gao, C. (2017). Benzo[a]pyrene induces autophagic and pyroptotic death simultaneously in HL-7702 human control liver cells. Journal of Agricultural and Food Chemistry, 65, 9763–9773. doi: 10.1021/acs.jafc.7b03248
- Yuan, L., Ren, X., Wu, Y., Wang, J., Xiao, H., & Liu, X. (2013). Isoorientin protects BRL-3A rat liver cell against hydrogen peroxide-induced apoptosis by inhibiting mitochondrial dysfunction,inactivating MAPKs, activating Akt and scavenging ROS and NO. Biomedicine & Aging Pathology, 3, 153–159. doi: 10.1016/j.biomag.2013.06.004
- Yuan, J., Zhu, M., Deng, S., Fan, S., Xu, H., Liao, J., … Chen, J. (2018). Classical swine fever virus induces pyroptosis in the peripheral lymphoid organs of infected pigs. Virus Research, 250, 37–42. doi: 10.1016/j.virusres.2018.04.004
- Zhang, S., Chen, J., Sun, A., & Zhao, L. (2014). Protective effects and antioxidant mechanism of bamboo leaf flavonoids on hepatocytes injured by CCl4. Food and Agricultural Immunology, 25, 386–396. doi: 10.1080/09540105.2013.810709
- Zhang, Y., Fan, Y., Gao, J., Xu, W., Xu, Z., Liu, Y., … Tao, L. (2019). A new 24-membered macrolide shows insecticidal activity against Pieris rapae potentially through induction of programmed cell death. Food and Agricultural Immunology, 30, 727–742. doi: 10.1080/09540105.2019.1626808
- Zhang, Y., Wang, X., Fu, Y., Yin, L., Pu, Y., & Liang, G. (2014). Expression profiling and pathway analysis of microRNA expression in the lungs of mice exposed to longterm, low-dose benzo(a)pyrene. Molecular & Cellular Toxicology, 10, 67–74. doi: 10.1007/s13273-014-0008-9
- Zhuang, C., Xu, N.-W., Gao, G.-M., Ni, S., Miao, K.-S., Li, C.-K., … Xie, H.-G. (2016). Polysaccharide from Angelica sinensis protects chondrocytes from H2O2-induced apoptosis through its antioxidant effects in vitro. International Journal of Biological Macromolecules, 87, 322–328. doi: 10.1016/j.ijbiomac.2016.02.031