ABSTRACT
Macrophage hyperactivation is associated with various inflammatory diseases. In this study, the effects of nine bisbenzylisoquinoline alkaloids on NO production by LPS-activated RAW264.7 macrophages were evaluated. It was found that certain bisbenzylisoquinoline alkaloids with single oxygen bridge have more potent effects than those with two oxygen bridges. Further studies revealed that three bisbenzylisoquinoline alkaloids (Neferine, Liensinine and Isoliensinine) of lotus (Nelumbo nucifera Gaertn.) seed embryo, a homology of traditional Chinese medicine and food, can also reduce LPS-induced expression of iNOS and production of IL-1β, IL-6 and TNF-α by blocking IκBα phosphorylation and degradation. And this effect is mediated at least partly by the inhibition of cytosolic Ca2+ level and CaM expression as well as the binding of three alkaloids with CaM, thereby inhibiting the subsequent phosphorylation of CaMKII. These results revealed for the first time that three bisbenzylisoquinoline alkaloids of lotus embryo play an anti-inflammatory role via suppressing Ca2+-CaM/CaMKII pathway.
1. Introduction
Macrophages are key components of the innate immune system that located throughout the tissues, where they defend against invading pathogens, remove the debris and dead cells by phagocytosis, and recruit other immune cells in response to inflammatory signals (Lavin, Mortha, Rahman, & Merad, Citation2015). However, hyperactivated macrophages alter their physiological functions significantly, producing nitric oxide (NO) and a variety of pro-inflammatory cytokines such as interleukin-1 beta (IL-1β), interleukin-6 (IL-6) and tumour necrosis factor-alpha (TNF-α), which can trigger various of inflammation-related diseases including microbial infection, tumorigenesis, neurodegeneration, autoimmunity, allergy and asthma, metabolism and obesity, and atherosclerosis. Thus, the inhibition of macrophage hyperactivation represents a therapeutic strategy to prevent inflammatory diseases (Murray & Wynn, Citation2011).
Ca2+-CaM/CaMKII pathway has been found to play an important role in the functions of immune cells. The intracellular Ca2+ concentration is kept within a tight range of 10−7–10−8 mol; Ca2+ overload occurs in immune cells during inflammation (Liu et al., Citation2008; Weber et al., Citation2006; Zhou, Yang, & Li, Citation2006). Calmodulin (CaM) is the primary calcium-binding protein and effector of Ca2+ (Chin & Means, Citation2000). Lipopolysaccharide (LPS) has been shown to induce macrophage activation by increasing the levels of cytosolic Ca2+ and CaM and the phosphorylation of their downstream effector, calcium/calmodulin-dependent protein kinase II (CaMKII) (Liu et al., Citation2008; Weber et al., Citation2006; Zhou et al., Citation2006). Nuclear factor (NF)-κB plays a prominent role in inflammation. Activation of NF-κB depends on IκB phosphorylation and subsequent separation from NF-κB (Peng et al., Citation2019). The liberated NF-κB is considered to regulate the transcription of inducible enzymes and inflammatory cytokines, such as inducible nitric oxide synthase (iNOS), IL-1β, IL-6 and TNF-α (Baeuerle & Henkel, Citation1994). CaMKII has been demonstrated to enhance LPS-induced phosphorylation of IκBα and subsequent activation of NF-κB, thus contributing to the significant increase of NO and inflammatory cytokines production in LPS-activated macrophages (Liu et al., Citation2008).
Bisbenzylisoquinoline alkaloids are formed between two 1-benzylisoquinoline monomers. About 500 natural products belong to this large alkaloid class (Weber & Opatz, Citation2019). Lots of bisbenzylisoquinoline alkaloids, such as Neferine, Tetrandrine, Cepharanthine, Berbamine and Dauricine, have been found to inhibit the activation of macrophages and thus have anti-inflammatory activity (Gao, Feng, Zhang, Wang, & Cui, Citation2016; Jia et al., Citation2017; Paudel, Karki, & Kim, Citation2016; Qiao et al., Citation2019; Wu, Guo, Min, Pei, & Chen, Citation2018). However, the structure-activity relationship of these compounds is still unclear.
Nelumbo nucifera Gaertn. (Lotus), a member of the Nymphaeaceae family, is a well-known edible plant widely cultivated in Asia, especially in China, Korea, Japan, Vietnam, Indian, and Thailand. Almost all organs of lotus including seeds, leaves, flowers and rhizomes have specific nutritional and medicinal values, which are considered as a traditional Chinese medicine and food. Lotus seeds, as edible vegetables, have a large market share. Embryo of lotus seeds is commonly prepared as weight-loss tea and traditional Chinese medicines. Recently, many studies have been done to analysis the active components and health care functions of lotus seed embryo (Deng et al., Citation2016; Fang, Li, Shao, Wang, & Wei, Citation2017). Lotus seeds embryo mainly contains three structurally related bisbenzylisoquinoline alkaloids, including Neferine, Liensinine and Isoliensinine (chemical structures shown in ; Sharma, Gautam, Adhikari, & Karki, Citation2017). Studies have documented that these three alkaloids exhibit a wide range of biological and pharmacological activities, such as cardiovascular protection (Saranya, Baskaran, Poornima, & Vijaya Padma, Citation2019; Yang et al., Citation2018), anticarcinogenesis (Yu, Liu, Chen, Li, & Li, Citation2018), neuroprotection (Cometa et al., Citation2012; Jung et al., Citation2010), antioxidation (Qian, Citation2002), and anti-inflammatory effects (Meng et al., Citation2017; Wu et al., Citation2018).
Neferine has been reported to have significant anti-inflammatory effect. It can reduce NO and TNF-α production in LPS-stimulated RAW264.7 macrophages by inhibiting the activation of NF-κB (Jung et al., Citation2010; Wu et al., Citation2018). Moreover, studies have documented that Neferine could inhibit LPS-induced inflammatory response in human umbilical endothelial cells (Liu et al., Citation2018). Our previous studies have demonstrated that Neferine, Liensinine and Isoliensinine exert significant anti-neuroinflammatory activity by suppressing the production of NO, IL-1β, IL-6, and TNF-α in LPS-activated microglial cells (a kind of macrophage-like cells in the central nervous system). And this effect is mediated by blocking NF-κB signalling pathway, as well as the production of reactive oxygen species (ROS) in LPS-stimulated microglial cells (Meng et al., Citation2017). However, the potential anti-inflammatory targets of these three alkaloids in the upstream pathway of NF-κB are still unclear. Previous studies on various cell types have shown that bisbenzylisoquinoline alkaloids, such as Neferine, Liensinine, Isoliensinine, Dauricine, Tetrandrine, Cepharanthine and Berbamine, are inhibitors of Ca2+ (Chen et al., Citation2007; Feng, Yu, & Xiao, Citation2002; Murakami, Okajima, & Uchiba, Citation2000; Qian, Citation2002; Ren et al., Citation2008; Wang, Nong, Yao, & Jiang, Citation2000). In addition, several bisbenzylisoquinoline alkaloids have been found to bind to CaM and inhibit the activity and expression of CaM (Hu, Chen, Hao, Huang, & Peng, Citation1989; Sun, Hu, & Xu, Citation1990; Xu & Zhang, Citation1986). All these results suggest that Ca2+-CaM signalling pathway may play a key role in the anti-inflammatory activity of bisbenzylisoquinoline alkaloids.
In the present study, the effects of nine bisbenzylisoquinoline alkaloids on the production of NO by LPS-activated RAW264.7 macrophages were investigated to find out their structure-activity relationship and more active compounds. Then, the influences of Neferine, Liensinine and Isoliensinine of lotus seed embryo on cytokines (IL-1β, IL-6 and TNF-α) production, on iNOS expression, and on IκBα phosphorylation and degradation were investigated. Thereafter, the mechanisms of three alkaloids inhibiting macrophage activation were discussed based on Ca2+-CaM/CaMKII pathway for the first time, including the detection of cytosolic free Ca2+ level ([Ca2+]i), the expression of CaM and phosphorylated CaMKII, and the binding ability of three alkaloids to CaM.
This study will reveal the key roles and new mechanisms of Neferine, Liensinine and Isoliensinine in the anti-inflammatory activity of the lotus seed embryo and open a new avenue to understand the potential of this health food in the treatment of inflammatory diseases accompanied by macrophage activation. In addition, this study will be helpful for the rapid screening and rational design of drugs that can inhibit macrophage activation.
2. Materials and methods
2.1. Cell culture and drug treatment
RAW264.7 macrophage cell line was obtained from the Cell Center of Peking Union Medical College (Beijing, China). The cells were cultured at 37°C in Dulbecco’s modified Eagle’s medium (DMEM, Hyclone, Logan, UT, USA) supplemented with 10% FBS (Gibco, GrandIsland, NY, USA) and 1% penicillin/streptomycin (Invitrogen, Carlsbad, CA, USA) in a fully humidified incubator with 5% CO2.
For the experiments, Neferine, Liensinine or Isoliensinine (purity > 98%, Chengdu Mansite Pharmaceutical Co. Ltd, Chengdu, Sichuan, China) was dissolved initially in dimethyl sulfoxide (DMSO, Sigma-Aldrich, St. Louis, MO, USA), and then diluted with DMEM medium without serum. DMSO at the highest concentration possibly present in experimental conditions (0.1%) was not toxic to the cells. RAW264.7 macrophages in exponential growth period were treated with Neferine, Liensinine or Isoliensinine (0.1–10 μM) in the presence or absence of LPS (1 μg/mL, Sigma-Aldrich).
2.2. Cell viability assay
The 3-(4,5-dimethylthiazol-2-yl)-2,5-diphenyltetrazolium bromide (MTT, Gibco) was used to detect the cell viability (Chen et al., Citation2018). RAW264.7 cells at 5 × 105 cells/well were seeded in 96-well plates and treated with 0.1–10 μM of nine bisbenzylisoquinoline alkaloids (Neferine, Liensinine, Isoliensinine, Tetrandrine, Fangchinoline, Isotetrandrine, Cepharanthine, Dauricine, or Berbamine hydrochloride) in the presence or absence of LPS (1 μg/mL) in the serum-free medium for 24 h. After the medium was removed, the cells were incubated with MTT (0.25 mg/mL) for 4 h at 37°C. The formazan crystals in the cells were dissolved with DMSO, and the absorbance was measured at 490 nm with a microplate reader (TECAN, Switzerland).
2.3. Nitrite assay
Accumulation of NO in media samples of cultured cells was analyzed by measuring nitrite levels using the Griess reagent (Meng et al., Citation2008). RAW264.7 macrophages were seeded at a density of 5 × 105 cells/well in 96-well plates and treated with 0.1–10 μM of nine bisbenzylisoquinoline alkaloids (Neferine, Liensinine, Isoliensinine, Tetrandrine, Fangchinoline, Isotetrandrine, Cepharanthine, Dauricine, or Berbamine hydrochloride) in the presence or absence of LPS (1 μg/mL) in the serum-free medium for 24 h. The supernatant fluid of the cells was then collected for nitrite assay. The culture supernatant fluid (50 μL) was mixed with 50 μL Griess reagent (obtained by mixing equal volume of 1% sulfanilamide in 5% phosphoric acid and 0.1% naphthylethylenediamine dihydrochloride in distilled water), and then the absorbance was measured at 540 nm by the microplate reader. The amount of nitrite was calculated from a NaNO2 standard curve.
2.4. Enzyme-linked immunosorbent assay (ELISA)
RAW264.7 cells (5 × 105cells/well) were incubated with Neferine, Liensinine or Isoliensinine (0.3–10 μM) in the presence of LPS (1 μg/mL). After incubation for 4 h, the supernatant fluid of the cells was collected to quantify the levels of IL-1β and IL-6 by the ELISA kits according to the manufacturer’s instructions (R&D Systems, Minneapolis, MN, USA). After incubation for 1 h, the level of TNF-α in the culture medium was detected by the ELISA kit (R&D Systems).
2.5. Reverse transcription PCR (RT-PCR) analysis
RAW264.7 cells were placed in 6-well plates and then treated with Neferine, Liensinine or Isoliensinine (1–10 μM) in the presence of LPS (1 μg/mL) for 24 h. The cells were harvested to detect the mRNA expression of IL-1β, IL-6, TNF-α and iNOS by RT-PCR. Briefly, Total RNA was extracted from the cells by RNAiso plus (TaKaRa Bio Inc., Dalian, China) according to manufacturer’s instructions. Total RNA (1 μg) was then reverse transcribed to cDNA using a PrimeScriptTM RT reagent kit with gDNA Eraser (TaKaRa Bio Inc.). The cDNA was then amplified by PCR with specific primers (). The following conditions of PCR reactions were applied: for iNOS and TNF-α, 30 cycles of denaturation at 94°C for 30 s, annealing at 58°C for 30 s, and extension at 72°C for 30 s; for GAPDH and IL-1β, 28 cycles of denaturation at 94°C for 30 s, annealing at 60°C for 30 s, and extension at 72°C for 30 s; for IL-6, 35 cycles of denaturation at 94°C for 30 s, annealing at 45°C for 30 s, and extension at 72°C for 30 s. The amplified products (5 μL) were separated on 1.2% agarose gels and visualized with ethidium bromide. Band intensities were quantified with Quantity One software (Bio-Rad, Hercules, CA, USA). Expression levels of the GAPDH gene were used for standardization.
Table 1. List of primers and product size for RT-PCR analysis.
2.6. Western blot assay
After being treated with Neferine, Liensinine or Isoliensinine (1–10 μM) in the presence of LPS (1 μg/mL) for 0.5–24 h, the cells were harvested and lysed with ice-cold radioimmunoprecipitation assay (RIPA) buffer (Beyotime, Shanghai, China) supplemented with protease inhibitors (1 mM phenylmethylsulfonyl fluoride, 1 μg/mL leupeptin, 1 μg/mL pepstatin and 1 μg/mL aprotinin) for 1 h at 4°C. The concentration of protein in the supernatant fluid of the lysate was determined by bicinchoninic acid (BCA) assay (CWbiotech, Beijing, China). Then proteins were separated on SDS-PAGE gels by electrophoresis and transferred onto polyvinylidene fluoride (PVDF, Millipore, Bedford, MA) membranes. The PVDF membranes were blocked in 5% skimmed milk at room temperature for 1 h and incubated with specific primary antibodies for iNOS, phosphorylated-IκBα, IκBα (all from Cell Signaling Technology, Danvers, MA), CaM, phosphorylated-CaMKII, CaMKII (all from Abcam, Cambridge, MA), or β-actin (Proteintech Group, Wuhan, China) overnight at 4°C, followed by horseradish peroxidase conjugated secondary antibodies (Santa Cruz Biotechnology, CA, USA). Specific bands were developed using enhanced chemiluminescence (ECL) reagents (Beyotime), visualized by Tanon-5200 Multi Chemiluminescent System (Tanon, Shanghai, China), and normalized to β-actin.
2.7. Cytosolic free Ca2+ level assay by flow cytometry
After being treated with Neferine, Liensinine or Isoliensinine (10 μM) in the presence of LPS (1 μg/mL) for 24 h, RAW264.7 cells were collected and loaded with 5 μM of Fluo-3/AM (Sigma) for 30 min at 37°C in dark in DMEM medium without serum. After 2 times of PBS washing, the cells were re-suspended in 300 μL of PBS, and cytosolic free Ca2+ level was analyzed by a FACScan flow cytometer (Becton-Dickinson, San Jose, CA, USA) as previously described (Kawanai et al., Citation2011).
2.8. Cytosolic free Ca2+ level assay by fluorescence spectrophotometer
The level of cytosolic free Ca2+ was also monitored using the Fura-2/AM fluorescent indicator as previously described (Meng et al., Citation2019). Briefly, the treated cells were washed twice with D-Hanks and loaded with 5 μM of Fura-2/AM (Sigma) at 37°C for 60 min in dark. After being washed twice with D-Hanks, the cells were resuspended in 1 mL of D-Hanks. Then, Triton X-100 and EGTA were added to the cell suspension (0.1% Triton X-100 and 10 mmol/L EGTA as an external solution). Fluorescence intensity of the cells was measured using the F-7000 fluorescence spectrophotometer (Hitachi, Japan), and the samples were excited alternatively at 340 and 380 nm and the fluorescence emission was monitored at 510 nm. The ratio of emissions at 510 nm (R = F340/F380) was recorded every 2 s and the level of cytosolic free Ca2+ was calculated based on the equation: [Ca2+]i = Kd × (Sf2/Sb2) × (R−Rmin)/(Rmax−R).
2.9. Molecular docking
CDOCKER is a powerful CHARMm-based molecular docking method that has been used to generate highly accurate docked poses (Wu, Robertson, Brooks, & Vieth, Citation2003). For the present investigation, molecular docking of three alkaloids of lotus seed embryo (Neferine, Liensinine and Isoliensinine) and two typical inhibitors of CaM (TFP and W7) to CaM was carried out by CDOCKER with Discovery studio 2.5QSAR. To perform flexible docking, all torsion angles of the small molecules were set to be free. The results were evaluated based upon CDOCKER energy. The lower CDOCKER energy implies more favourable binding. 2D images were obtained to analysis the binding interaction of Neferine, Liensinine and Isoliensinine with CaM.
2.10. Statistical analysis
Data were represented as the means ± SEM of three experiments. Statistical analysis was performed using SPSS 19.0 software. The data were analyzed by one-way ANOVA followed by Dunnett’s t-test among three or more groups. Significance between two groups was analyzed by t-test.
3. Results
3.1. Effects of nine bisbenzylisoquinoline alkaloids on LPS-induced NO release from RAW264.7 macrophages
To avoid the possible effects of reduced viability on the release of NO and cytokines, the cell viability of nine bisbenzylisoquinoline alkaloids (0.1–10 μM) on RAW264.7 macrophages in the presence or absence of LPS (1 μg/mL) was examined. The result indicated that the cell viability of either unstimulated or stimulated macrophages was not significantly affected by the addition of nine alkaloids (data not shown).
Subsequently, NO production by resting or LPS-activated macrophages was detected by Griess assay. As shown in , these nine alkaloids had different degrees of inhibitory effects on the release of NO induced by LPS. Isoliensinine, Neferine, Tetrandrine, Dauricine, Liensinine and Cepharanthine possessed strong inhibitory activity (IC50 = 2.1–5.5 μM), whereas Fangchinoline, Isotetrandrine and Berbamine hydrochloride had little activity (IC50 > 10 μM). In addition, Isoliensinine (IC50 = 2.1 μM) and Neferine (IC50 = 2.6 μM) had more potent effects than other seven compounds. Furthermore, treatment of unstimulated macrophages with nine alkaloids (0.1–10 μM) for 24 h did not result in any change of NO production (data not shown).
Table 2. Effects of nine bisbenzylisoquinoline alkaloids on NO production by LPS-activated RAW264.7 cells.
3.2. Effects of Neferine, Liensinine and Isoliensinine on LPS-induced release and mRNA expression of IL-1β, IL-6 and TNF-α
To further investigate the suppressive effects of Neferine, Liensinine and Isoliensinine on macrophage activation, the release of three cytokines (IL-1β, IL-6 and TNF-α) from LPS-stimulated RAW264.7 cells were assessed by ELISA, and mRNA expression of three cytokines in the cells were detected by RT-PCR. And it was shown that the levels of IL-1β, IL-6 and TNF-α in cell-conditioned medium after LPS treatment were significantly elevated compared to the control group. Neferine, Liensinine and Isoliensinine could significantly inhibit LPS-induced relaese of IL-1β ((A–C)), IL-6 ((D–F)) and TNF-α ((G–I)) in a concentration-dependent manner (). Furthermore, the increased expression of IL-1β, IL-6 and TNF-α mRNA in LPS-activated macrophages was also reduced by Neferine, Liensinine and Isoliensinine (), indicating that the three alkaloids inhibited the production of three cytokines (IL-1β, IL-6 and TNF-α) at the transcriptional level.
Figure 1. Effects of Neferine, Liensinine and Isoliensinine on the release of IL-1β, IL-6 and TNF-α from LPS-activated macrophages. RAW264.7 macrophages were treated with Neferine (A, D and G), Liensinine (B, E and H) or Isoliensinine (C, F and I) (0.3–10 μM) in the presence of LPS (1 μg/mL). After incubation for 4 h, the levels of IL-1β (A–C) and IL-6 (D–F) in the culture medium were measured. After incubation for 1 h, the level of TNF-α (G–I) in the culture medium were detected. ###P < 0.001 as compared with the blank control group (Con), **P < 0.01, ***P < 0.001 as compared with the LPS alone group.
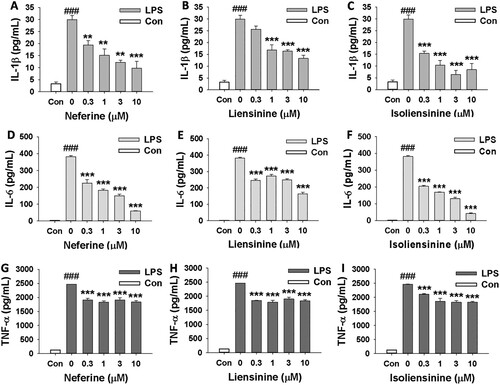
Figure 2. Effects of Neferine, Liensinine and Isoliensinine on the mRNA expression of IL-1β, IL-6 and TNF-α in LPS-stimulated macrophages. RAW264.7 macrophages were treated with Neferine (A), Liensinine (B) or Isoliensinine (C) (1–10 μM) in the presence of LPS (1 μg/mL) for 24 h. The mRNA expression of IL-1β, IL-6 or TNF-α was detected by RT-PCR. ###P < 0.001 as compared with the blank control group (Con), *P < 0.05, **P < 0.01, ***P < 0.001 as compared with the LPS alone group.
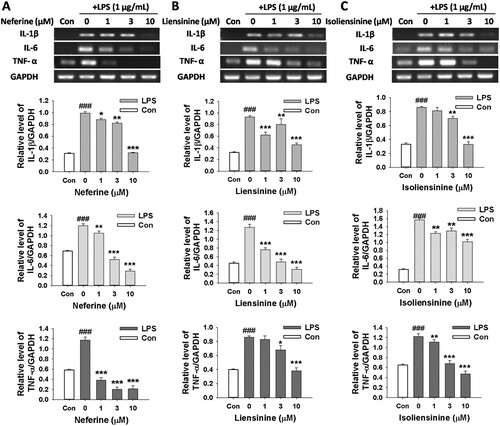
3.3. Effects of Neferine, Liensinine and Isoliensinine on iNOS expression in activated macrophages
In order to evaluate whether the inhibitory effects of Neferine, Liensinine and Isoliensinine on NO production are associated with the expression of iNOS, the mRNA and protein expression of iNOS in macrophages was detected by RT-PCR and western blot, respectively. As shown in , the expression levels of iNOS mRNA and protein were nearly undetectable in unstimulated RAW264.7 cells. However, stimulation of the cells with LPS led to a significant increase in iNOS mRNA and protein expression. Neferine, Liensinine and Isoliensinine exhibited the potent inhibitory effects on iNOS expression at both the mRNA ((A–C)) and protein ((D–F)) levels.
Figure 3. Effects of Neferine, Liensinine and Isoliensinine on LPS-induced expression of iNOS mRNA and protein in macrophages. RAW264.7 cells were treated with Neferine (A and D), Liensinine (B and E) or Isoliensinine (C and F) (1–10 μM) in the presence of LPS (1 μg/mL) for 24 h. (A–C) The expression of iNOS mRNA was analyzed by RT-PCR. (D–F) The expression of iNOS protein was detected by western blot. ###P < 0.001 as compared with the blank control group (Con), *P < 0.05, **P < 0.01, ***P < 0.001 as compared with the LPS alone group.
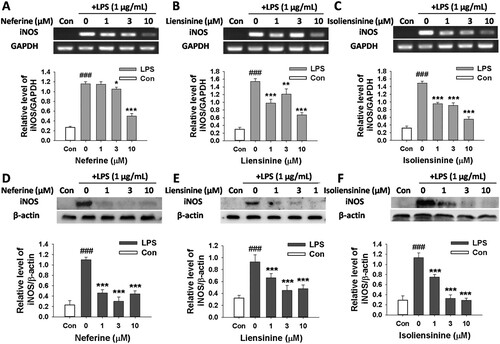
3.4. Effects of Neferine, Liensinine and Isoliensinine on the phosphorylation and degradation of IκBα in activated macrophages
Next, we investigated the effects of three alkaloids on the expression of phosphorylated-IκBα and IκBα protein. The stimulation of macrophages with LPS resulted in the increase of phosphorylated-IκBα level and the decrease of IκBα level, indicating that IκBα was significantly phosphorylated and degraded. Neferine, Liensinine and Isoliensinine inhibited LPS-induced phosphorylation and degradation of IκBα (), indicating the potential role of NF-κB in the possible mechanism of the three alkaloids in suppressing the production NO, IL-1β, IL-6 and TNF-α.
Figure 4. Effects of Neferine, Liensinine and Isoliensinine on LPS-induced IκBα phosphorylation and degradation in macrophages. RAW264.7 cells were treated with Neferine (A), Liensinine (B) or Isoliensinine (C) (1–10 μM) in the presence of LPS (1 μg/mL) for 0.5 h. Phosphorylated or total IκBα was detected by western blot. ##P < 0.01, ###P < 0.001 as compared with the blank control group (Con), **P < 0.01, ***P < 0.001 as compared with the LPS alone group.
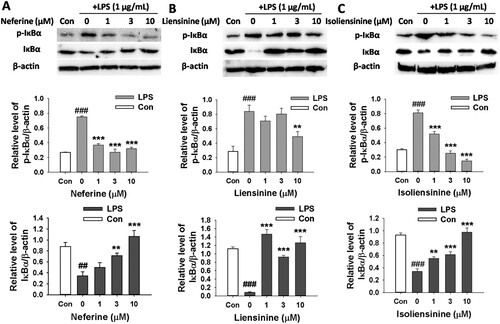
3.5 . Effects of Neferine, Liensinine and Isoliensinine on cytosolic free Ca2+ level in LPS-activated RAW264.7 cells
Since changes in intracellular Ca2+ levels can lead to the activation of NF-κB in LPS-stimulated macrophages (Zhou et al., Citation2006), and Neferine, Liensinine and Isoliensinine are inhibitors of Ca2+ in ventricular myocytes and corpus cavernosum smooth muscle cells (Chen et al., Citation2007; Feng et al., Citation2002; Wang et al., Citation2000), the effects of Neferine, Liensinine and Isoliensinine on the level of cytosolic free Ca2+ in LPS-activated RAW264.7 macrophages were investigated. Flow cytometry analysis showed that increased cytosolic free Ca2+ level in LPS-stimulated macrophages was significantly inhibited by treatment with Neferine, Liensinine or Isoliensinine (10 μM), and Isoliensinine had more potent inhibitory activity than Neferine and Liensinine ((A)). Further studies by fluorescence spectrophotometer also showed that increased cytosolic free Ca2+ level by LPS was attenuated by treatment with three alkaloids (10 μM), and Isoliensinine also had stronger inhibitory activity than Neferine and Liensinine ((B)).
Figure 5. Effects of Neferine, Liensinine and Isoliensinine on cytosolic free Ca2+ level in LPS-activated macrophages. RAW264.7 cells were treated with Neferine, Liensinine and Isoliensinine (10 μM) in the presence of LPS (1 μg/mL) for 24 h. (A) Cytosolic free Ca2+ level in macrophages were tested by Fluo-3/AM labelling and flow cytometry analysis. (B) Cytosolic free Ca2+ level in the cells were tested by Fura-2/AM labelling and fluorescence spectrophotometer analysis. #P < 0.05, ##P < 0.01 as compared with the blank control group (Con), *P < 0.05, **P < 0.01, ***P < 0.001 as compared with the LPS alone group.

3.6. Effects of Neferine, Liensinine and Isoliensinine on the expression of CaM, phosphorylated-CaMKII and total CaMKII in LPS-activated macrophages
CaM is the critical effector of Ca2+. Furthermore, CaMKII, one of the main downstream targets of Ca2+ and CaM, is activated by Ca2+/CaM (Bi et al., Citation2019; Chin & Means, Citation2000; Ohmori & Hamilton, Citation1992). To further elucidate the role of CaM and CaMKII in the inhibition of macrophage activation by three alkaloids, the expression of CaM, phosphorylated-CaMKII and total CaMKII was detected by western blot assay. As shown in , LPS-induced up-regulation of CaM and phosphorylated-CaMKII in macrophages was significantly suppressed by treatment with Neferine, Liensinine or Isoliensinine (10 μM). However, the expression of total CaMKII in cells was not affected. Taken together, these observations suggested that these three compounds inhibited LPS-induced macrophage activation through regulation of CaM/CaMKII signalling.
Figure 6. Effects of Neferine, Liensinine and Isoliensinine on the expression of CaM, phosphorylated CaMKII and total CaMKII in LPS-activated macrophages. RAW264.7 macrophages were treated with Neferine, Liensinine or Isoliensinine in the presence of LPS (1 μg/mL) for 24 h. The expression of CaM (A–C), phosphorylated CaMKII and total CaMKII (D) was determined by western blot. #P < 0.05, ##P < 0.01, ###P < 0.001 as compared with the blank control group (Con), *P < 0.05, **P < 0.01, ***P < 0.001 as compared with the LPS alone group.
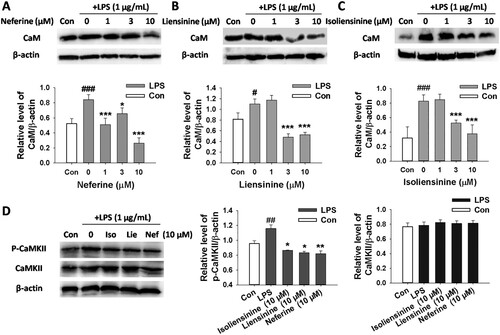
3.7. Molecular docking
Some of bisbenzylisoquinoline alkaloids have been found to be potential calmodulin antagonists by binding with CaM (Hu et al., Citation1989; Sun et al., Citation1990; Xu & Zhang, Citation1986). To preliminarily determine whether the inhibitory effects of Neferine, Liensinine and Isoliensinine on macrophage activation may be related to their binding to CaM, molecular docking was carried out to evaluate the binding ability of three alkaloids and two typical CaM inhibitors (TFP and W7) to CaM by CDOCKER with Discovery studio 2.5QSAR. As shown in (A), CaM molecule has two docking sites, site 1 and site 2. The CDOCKER scores of these compounds are displayed in . It was showed that the binding ability of five compounds to site 1 of CaM was stronger than that of site 2. The CDOCKER energy of Isoliensinine, Liensinine, or Neferine binding to CaM site 1 was less than zero, implying that they may have potential to bind to CaM. The CDOCKER energy order of the compounds binding to CaM site 1 is as follows: TFP < Isoliensinine < W7 < Liensinine < Neferine. And Isoliensinine displayed lower CDOCKER energy than Liensinine or Neferine, implying more favourable binding. Furthermore, analysis of 2D images ((B–D)) showed that the hydroxyl group in the C-ring of Isoliensinine or Liensinine can form an H-bond with the amino acid residue Lys75 of CaM, and the B-ring of Isoliensinine can also form a σ-π bond with Lys75; the B-ring of Neferine can form a π-π bond with the benzene ring of the amino acid residue Phe19 in CaM.
Figure 7. The binding ability of Neferine, Liensinine and Isoliensinine with CaM. (A) Active docking sites in CaM. (B–D) 2D representation of docking interaction of Neferine (B), Liensinine (C) or Isoliensinine (D) with CaM.
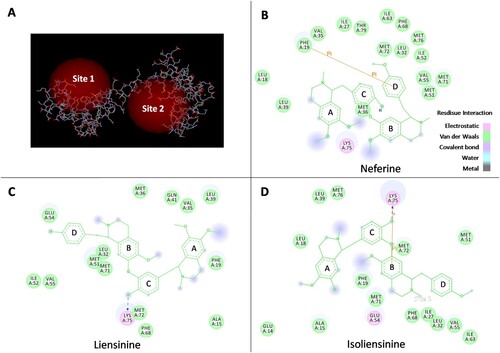
Table 3. CDOCKER scores.
4. Discussion
Hyperactivated macrophages play an important role in the progression of various inflammatory diseases. Correspondingly, suppression of macrophage hyperactivation will lead to the attenuation of formation and development of inflammation-related diseases.
In the present study, the inhibitory effects of nine bisbenzylisoquinoline alkaloids on NO release from LPS-activated RAW264.7 macrophages were compared. This is the first report of the structure–activity relationships of these compounds in this regard. The results demonstrated that Neferine, Liensinine, Isoliensinine and Dauricine have more potent effects on NO production than Fangchinoline, Isotetrandrine, Cepharanthine and Berbamine hydrochloride. This may be due to the fact that bisbenzylisoquinoline alkaloids with a single oxygen bridge are more conducive to chemical rotation than those with two oxygen bridges. Furthermore, for bisbenzylisoquinoline alkaloids with two oxygen bridges, the enantiomers with S configuration at C1 (Tetrandrine, Fangchinoline and Cepharanthine) have more inhibitory activity on NO production than those with R configuration at C1 (Cepharanthine and Berbamine hydrochloride). The above information might be useful for the chemical modification of bisbenzylisoquinoline alkaloids and rational design of new drugs.
Liensinine and its analogs (Neferine and Isoliensinine), the major active alkaloids in the green embryo of lotus seeds, were chosen for further study. Lotus seed embryo is a famous traditional Chinese medicine to remove heat from the heart, anchor the mind, improve seminal emission, and arrest bleeding used for centuries in China (Jiang et al., Citation2018). Lotus seeds are also made as a folk dish in Asia. As the homology of traditional Chinese medicine and food, lotus seed embryo has high pharmacological effects and nutritional values (Deng et al., Citation2016; Fang et al., Citation2017).
In our previous study, Neferine, Liensinine and Isoliensinine were found to inhibit inflammatory response of microglial cells induced by LPS, including the production of NO, IL-1β, IL-6, and TNF-α, by blocking the NF-κB pathway (Meng et al., Citation2017). Our current results revealed that these three alkaloids of lotus seed embryo could also suppress IL-1β, IL-6 and TNF-α production at transcriptional level in LPS-stimulated macrophages. NO is mainly synthesized by iNOS in activated immune cells (Murakami & Ohigashi, Citation2007). In this study, three alkaloids inhibited both protein and mRNA expression of iNOS in activated macrophages, suggesting that the suppression of NO production may be attributed to the inhibition of iNOS transcription and subsequently the reduction of protein production. NF-κB is a major transcription factor in the regulation of cytokines and inducible enzymes, such as IL-1β, IL-6, TNF-α and iNOS (Baeuerle & Henkel, Citation1994). Activation of NF-κB depends on phosphorylation and subsequent degradation of IκB protein; IκBα is the most well characterized (Vallabhapurapu & Karin, Citation2009). Our results indicated that the decreased expression of iNOS, IL-1β, IL-6 and TNF-α in macrophages was due to the inhibition of phosphorylation and degradation of IκBα. The effect of Neferine on macrophage activation is consistent with previous reports (Jung et al., Citation2010; Wu et al., Citation2018). Thus, we can conclude that all of these three major alkaloids play an important role in the anti-inflammatory effect of lotus seed embryo. Moreover, our results showed that Isoliensinine has more potent inhibitory effect on macrophage activation than Neferine and Liensinine, indicating that it may have a better application prospect to be developed as a single-compound drug than Neferine and Liensinine.
Although these results suggest that NF-κB pathway plays a key role in the anti-inflammatory effects of three alkaloids, the potential anti-inflammatory targets of these compounds in the upstream pathway of NF-κB remain unclear. Therefore, further studies were carried out to explore their possible anti-inflammatory targets and upstream signal transduction pathway of NF-κB. Ca2+-CaM/CaMKII pathway was found to be significantly activated in LPS-stimulated macrophages, including the increase of intracellular Ca2+ level and CaM expression as well as subsequent CaMKII phosphorylation (Letari, Nicosia, Chiavaroli, Vacher, & Schlegel, Citation1991; Liu et al., Citation2008; Ohmori & Hamilton, Citation1992; Weber et al., Citation2006; Zhou et al., Citation2006). The activation of NF-κB depends on changes in intracellular Ca2+ level, and both CaM and CaMKII help regulate NF-κB activation in T-cells and macrophages after stimulation (Liu et al., Citation2008; Oruganti, Edin, Grundström, & Grundström, Citation2011; Zhou et al., Citation2006). Since Neferine, Liensinine and Isoliensinine are inhibitors of Ca2+ in many cell types, including ventricular myocytes and corpus cavernosum smooth muscle cells (Chen et al., Citation2007; Feng et al., Citation2002; Wang et al., Citation2000), the mechanism of three alkaloids inhibiting the phosphorylation and degradation of IκBα in macrophages was discussed based on Ca2+-CaM/CaMKII pathway. It was shown for the first time that Neferine, Liensinine and Isoliensinine could significantly inhibit the levels of cytosolic free Ca2+ in LPS-activated RAW264.7 cells, and that Isoliensinine also had a stronger inhibitory effect on the level of cytosolic free Ca2+ than Neferine and Liensinine. In addition, it was found for the first time that these three alkaloids could reduce the expression of CaM and phosphorylation of CaMKII. It has been reported that some bisbenzylisoquinoline alkaloids can bind to CaM and inhibit the activation of CaM (Hu et al., Citation1989; Sun et al., Citation1990; Xu & Zhang, Citation1986). Therefore, CaM may be one of the targets of bisbenzylisoquinoline alkaloids to inhibit macrophage activation. The results of molecular docking of Neferine, Liensinine and Isoliensinine to CaM indicated that all of these three alkaloids have the ability to bind to the site 1 of CaM, implying that CaM may be one of the targets of the three alkaloids to inhibit macrophage activation. It can be seen from the CDOCKER energy that Isoliensinine also displayed a stronger binding ability to CaM than Neferine and Liensinine, and its binding ability to CaM was even stronger than that of W7 (a typical inhibitor of CaM). This might be due to the formation of H-bond and σ-π bond between CaM and Isoliensinine. All of these results suggested that Neferine, Liensinine and Isoliensinine may suppress NF-κB signalling at least partly by inhibiting Ca2+-CaM/CaMKII pathway in cells.
In addition, intracellular ROS generation and mitogen-activated protein kinase (MAPK) activation augment NF-κB activation through the phosphorylation and degradation of IκB, leading to inflammation (Guha & Mackman, Citation2001; Morgan & Liu, Citation2011). Neferine, Liensinine and Isoliensinine have also been demonstrated to scavenge free radicals and suppress the ROS generation in LPS-stimulated microglial cells (Meng et al., Citation2017). And Neferine was proved to exert anti-inflammatory effect on LPS-stimulated human endothelial cells via MAPK pathway (Feng et al., Citation2002). Whether the ROS and MAPK signalling contribute to the inhibition of NF-κB activation by Neferine, Liensinine and Isoliensinine in LPS-stimulated macrophages will be further investigated. Moreover, further studies are needed to evaluate the efficacy and safety of these compounds in animal models of inflammatory diseases in vivo.
In conclusion, this is the first report that three bisbenzylisoquinoline alkaloids (Neferine, Liensinine and Isoliensinine) of lotus (Nelumbo nucifera Gaertn.) seed embryo exert anti-inflammatory effects by inhibiting Ca2+-CaM/CaMKII signalling pathway. Neferine, Liensinine and Isoliensinine inhibited the production of NO, IL-1β, IL-6 and TNF-α, the expression of iNOS, as well as the phosphorylation and degradation of IκBα in LPS-activated macrophages, and this effect is mediated at least partly by inhibiting Ca2+-CaM/CaMKII pathway in cells. These results revealed a new anti-inflammatory mechanism of Neferine, Liensinine and Isoliensinine of lotus seed embryo and open a new avenue to better understand the potential of this health food and medicine in the treatment of various inflammatory diseases. The present results provide a better understanding of the structure-activity relationship of bisbenzylisoquinoline alkaloids for the inhibition of LPS-induced NO production from macrophages. This information might be helpful for the rapid screening of candidate drugs from bisbenzylisoquinoline alkaloids and the rational design of new drugs.
Disclosure statement
No potential conflict of interest was reported by the authors.
Additional information
Funding
References
- Baeuerle, P. A., & Henkel, T. (1994). Function and activation of NF-kappaB in the immune system. Annual Review of Immunology, 12, 141–179. doi: 10.1146/annurev.iy.12.040194.001041
- Bi, H., Sun, Z., Chu, Q. B., Li, L. Z., Guan, X., Zhou, Y. L., & Li, Z. W. (2019). Analgesic effects of astilbin partially via calcium channels through regulation on CaMKII. Food and Agricultural Immunology, 30(1), 309–319. doi: 10.1080/09540105.2019.1580677
- Chen, J., Liu, J. H., Jiang, Z. J., Wang, T., Liu, B., Yang, J., … Chen, F. (2007). Effects of neferine on cytosolic free calcium concentration in corpus cavernosum smooth muscle cells of rabbits. Andrologia, 39(4), 141–145. doi: 10.1111/j.1439-0272.2007.00781.x
- Chen, Q., Qi, C., Peng, G., Liu, Y., Zhang, X., & Meng, Z. (2018). Immune-enhancing effects of a polysaccharide PRG1-1 from Russula griseocarnosa on RAW264.7 macrophage cells via the MAPK and NF-κB signaling pathways. Food and Agricultural Immunology, 29(1), 833–844. doi: 10.1080/09540105.2018.1461198
- Chin, D., & Means, A. R. (2000). Calmodulin: A prototypical calcium sensor. Trends in Cell Biology, 10(8), 322–328. doi: 10.1016/S0962-8924(00)01800-6
- Cometa, M. F., Fortuna, S., Palazzino, G., Volpe, M. T., Rengifo Salgado, E., Nicoletti, M., & Tomassini, L. (2012). New cholinesterase inhibiting bisbenzylisoquinoline alkaloids from Abuta grandifolia. Fitoterapia, 83(3), 476–480. doi: 10.1016/j.fitote.2011.12.015
- Deng, X., Zhu, L., Fang, T., Vimolmangkang, S., Yang, D., Ogutu, C., … Han, Y. (2016). Analysis of isoquinoline alkaloid composition and wound-induced variation in Nelumbo using HPLC-MS/MS. Journal of Agricultural and Food Chemistry, 64(5), 1130–1136. doi: 10.1021/acs.jafc.5b06099
- Fang, Y., Li, Q., Shao, Q., Wang, B., & Wei, Y. (2017). A general ionic liquid pH-zone-refining countercurrent chromatography method for separation of alkaloids from Nelumbo nucifera Gaertn. Journal of Chromatography A, 1507, 63–71. doi: 10.1016/j.chroma.2017.05.048
- Feng, X., Yu, X., & Xiao, J. (2002). Effect of isoliensinine on cardiovascular function and experimental left ventricular hypertrophy. Journal of Huazhong University of Science and Technology (Medical Sciences), 31, 608–611.
- Gao, L. N., Feng, Q. S., Zhang, X. F., Wang, Q. S., & Cui, Y. L. (2016). Tetrandrine suppresses articular inflammatory response by inhibiting pro-inflammatory factors via NF-κB inactivation. Journal of Orthopaedic Research, 34(9), 1557–1568. doi: 10.1002/jor.23155
- Guha, M., & Mackman, N. (2001). LPS induction of gene expression in human monocytes. Cellular Signalling, 13(2), 85–94. doi: 10.1016/S0898-6568(00)00149-2
- Hu, Z. Y., Chen, S. L., Hao, Z. G., Huang, W. L., & Peng, S. X. (1989). Benzylisoquinoline compounds inhibit the ability of calmodulin to activate cyclic nucleotide phosphodiesterase. Cellular Signalling, 1(2), 181–185. doi: 10.1016/0898-6568(89)90008-9
- Jia, X. J., Li, X., Wang, F., Liu, H. Q., Zhang, D. J., & Chen, Y. (2017). Berbamine exerts anti-inflammatory effects via inhibition of NF-κB and MAPK signaling pathways. Cellular Physiology and Biochemistry, 41(6), 2307–2318. doi: 10.1159/000475650
- Jiang, X. L., Wang, L., Wang, E. J., Zhang, G. L., Chen, B., Wang, M. K., & Li, F. (2018). Flavonoid glycosides and alkaloids from the embryos of Nelumbo nucifera seeds and their antioxidant activity. Fitoterapia, 125, 184–190. doi: 10.1016/j.fitote.2018.01.009
- Jung, H. A., Jin, S. E., Choi, R. J., Kim, D. H., Kim, Y. S., Ryu, J. H., … Choi, J. S. (2010). Anti-amnesic activity of neferine with antioxidant and anti-inflammatory capacities, as well as inhibition of ChEs and BACE1. Life Sciences, 87(13–14), 420–430. doi: 10.1016/j.lfs.2010.08.005
- Kawanai, T., Fujinaga, M., Koizumi, K., Kurotani, I., Hashimoto, E., Satoh, M., … Oyama, Y. (2011). Some characteristics of membrane Cd²+ transport in rat thymocytes: An analysis using Fluo-3. Biometals, 24(5), 903–914. doi: 10.1007/s10534-011-9444-3
- Lavin, Y., Mortha, A., Rahman, A., & Merad, M. (2015). Regulation of macrophage development and function in peripheral tissues. Nature Reviews Immunology, 15(12), 731–744. doi: 10.1038/nri3920
- Letari, O., Nicosia, S., Chiavaroli, C., Vacher, P., & Schlegel, W. (1991). Activation by bacterial lipopolysaccharide causes changes in the cytosolic free calcium concentration in single peritoneal macrophages. Journal of Immunology, 147(3), 980–983.
- Liu, X. Y., Xu, H. X., Li, J. K., Zhang, D., Ma, X. H., Huang, L. N., … Wang, X. Z. (2018). Neferine protects endothelial glycocalyx via mitochondrial ROS in lipopolysaccharide-induced acute respiratory distress syndrome. Frontiers in Physiology, 9, 1–14.
- Liu, X., Yao, M., Li, N., Wang, C., Zheng, Y., & Cao, X. (2008). CaMKII promotes TLR-triggered proinflammatory cytokine and type I interferon production by directly binding and activating TAK1 and IRF3 in macrophages. Blood, 112(13), 4961–4970. doi: 10.1182/blood-2008-03-144022
- Meng, X. L., Chen, C. L., Liu, Y. Y., Su, S. J., Gou, J. M., Huan, F. N., … Lu, J. (2019). Selenoprotein SELENOK enhances the migration and phagocytosis of microglial cells by increasing the cytosolic free Ca2+ level resulted from the up-regulation of IP3R. Neuroscience, 406, 38–49. doi: 10.1016/j.neuroscience.2019.02.029
- Meng, X. L., Yang, J. Y., Chen, G. L., Zhang, L. J., Wang, L. H., Li, J., & Wu, C. F. (2008). RV09, a novel resveratrol analogue, inhibits NO and TNF-alpha production by LPS-activated microglia. International Immunopharmacology, 8(8), 1074–1082. doi: 10.1016/j.intimp.2008.03.011
- Meng, X. L., Zheng, L. C., Liu, J., Gao, C. C., Qiu, M. C., Liu, Y. Y., … Chen, C. L. (2017). Inhibitory effects of three bisbenzylisoquinoline alkaloids on lipopolysaccharide-induced microglial activation. RSC Advances, 7, 18347–18357. doi: 10.1039/C7RA01882G
- Morgan, M. J., & Liu, Z. G. (2011). Crosstalk of reactive oxygen species and NF-κB signaling. Cell Research, 21(1), 103–115. doi: 10.1038/cr.2010.178
- Murakami, A., & Ohigashi, H. (2007). Targeting NOX, INOS and COX-2 in inflammatory cells: Chemoprevention using food phytochemicals. International Journal of Cancer, 121(11), 2357–2363. doi: 10.1002/ijc.23161
- Murakami, K., Okajima, K., & Uchiba, M. (2000). The prevention of lipopolysaccharide-induced pulmonary vascular injury by pretreatment with cepharanthine in rats. American Journal of Respiratory and Critical Care Medicine, 161(1), 57–63. doi: 10.1164/ajrccm.161.1.9808142
- Murray, P. J., & Wynn, T. A. (2011). Protective and pathogenic functions of macrophage subsets. Nature Reviews Immunology, 11(11), 723–737. doi: 10.1038/nri3073
- Ohmori, Y., & Hamilton, T. A. (1992). Ca2+ and calmodulin selectively regulate lipopolysaccharide-inducible cytokine mRNA expression in murine peritoneal macrophages. Journal of Immunology, 148(2), 538–545.
- Oruganti, S. R., Edin, S., Grundström, C., & Grundström, T. (2011). CaMKII targets Bcl10 in T-cell receptor induced activation of NF-κB. Molecular Immunology, 48(12–13), 1448–1460. doi: 10.1016/j.molimm.2011.03.020
- Paudel, K. R., Karki, R., & Kim, D. W. (2016). Cepharanthine inhibits in vitro VSMC proliferation and migration and vascular inflammatory responses mediated by RAW264.7. Toxicology in Vitro, 34, 16–25. doi: 10.1016/j.tiv.2016.03.010
- Peng, Y., Hu, M. J., Lu, Q., Tian, Y., He, W. Y., Chen, L., … Pan, S. Y. (2019). Flavonoids derived from Exocarpium Citri Grandis inhibit LPS-induced inflammatory response via suppressing MAPK and NF-kappa B signalling pathways. Food and Agricultural Immunology, 30(1), 564–580. doi: 10.1080/09540105.2018.1550056
- Qian, J. Q. (2002). Cardiovascular pharmacological effects of bisbenzylisoquinoline alkaloid derivatives. Acta Pharmacologica Sinica, 23(12), 1086–1092.
- Qiao, B., Wang, H., Wang, C., Liang, M., Huang, K., & Li, Y. (2019). Dauricine negatively regulates lipopolysaccharide- or cecal ligation and puncture-induced inflammatory response via NF-κB inactivation. Archives of Biochemistry and Biophysics, 666, 99–106. doi: 10.1016/j.abb.2019.03.018
- Ren, Y., Lu, L., Guo, T. B., Qiu, J., Yang, Y., Liu, A., & Zhang, J. Z. (2008). Novel immunomodulatory properties of berbamine through selective down-regulation of STAT4 and action of IFN-gamma in experimental autoimmune encephalomyelitis. The Journal of Immunology, 181(2), 1491–1498. doi: 10.4049/jimmunol.181.2.1491
- Saranya, S., Baskaran, R., Poornima, P., & Vijaya Padma, V. (2019). Berbamine ameliorates isoproterenol-induced myocardial infarction by inhibiting mitochondrial dysfunction and apoptosis in rats. Journal of Cellular Biochemistry, 120(3), 3101–3113. doi: 10.1002/jcb.27522
- Sharma, B. R., Gautam, L. N., Adhikari, D., & Karki, R. (2017). A comprehensive review on chemical profiling of Nelumbo Nucifera: Potential for drug development. Phytotherapy Research, 31(1), 3–26. doi: 10.1002/ptr.5732
- Sun, Y., Hu, Z. Y., & Xu, L. M. (1990). Studies on the interaction of daurisoline alkaloid derivatives and calmodulin by fluorescence spectroscopy. Second Messengers and Phosphoproteins, 13(1), 51–57.
- Vallabhapurapu, S., & Karin, M. (2009). Regulation and function of NF-kappaB transcription factors in the immune system. Annual Review of Immunology, 27, 693–733. doi: 10.1146/annurev.immunol.021908.132641
- Wang, J. L., Nong, Y., Yao, W. X., & Jiang, M. X. (2000). Effects of liensinine on action potentialsand INa, ICa-L in guinea pig ventricular myocytes. China Tradit Herb Drugs, 31, 193–196.
- Weber, C., & Opatz, T. (2019). Bisbenzylisoquinoline Alkaloids. The Alkaloids: Chemistry and Biology, 81, 1–114.
- Weber, T. J., Smallwood, H. S., Kathmann, L. E., Markillie, L. M., Squier, T. C., & Thrall, B. D. (2006). Functional link between TNF biosynthesis and CaM-dependent activation of inducible nitric oxide synthase in RAW 264.7 macrophages. American Journal of Physiology-Cell Physiology, 290(6), C1512–C1520. doi: 10.1152/ajpcell.00527.2005
- Wu, X., Guo, Y., Min, X., Pei, L., & Chen, X. (2018). Neferine, a bisbenzylisoquinoline alkaloid, ameliorates dextran sulfate sodium-induced ulcerative colitis. The American Journal of Chinese Medicine, 46(6), 1263–1279. doi: 10.1142/S0192415X18500660
- Wu, G., Robertson, D. H., Brooks, C. L., & Vieth, M. (2003). Detailed analysis of grid-based molecular docking: A case study of CDOCKER-A CHARMm-based MD docking algorithm. Journal of Computational Chemistry, 24(13), 1549–1562. doi: 10.1002/jcc.10306
- Xu, Y. H., & Zhang, S. P. (1986). A derivative of bisbenzylisoquinoline alkaloid is a new and potential calmodulin antagonist. Biochemical and Biophysical Research Communications, 140(1), 461–467. doi: 10.1016/0006-291X(86)91113-7
- Yang, G. M., Sun, J., Pan, Y., Zhang, J. L., Xiao, M., & Zhu, M. S. (2018). Isolation and identification of a tribenzylisoquinoline alkaloid from Nelumbo nucifera Gaertn, a novel potential smooth muscle relaxant. Fitoterapia, 124, 58–65. doi: 10.1016/j.fitote.2017.10.020
- Yu, M., Liu, T., Chen, Y., Li, Y., & Li, W. (2018). Combination therapy with protein kinase inhibitor H89 and Tetrandrine elicits enhanced synergistic antitumor efficacy. Journal of Experimental & Clinical Cancer Research, 37(1), 1–16. doi: 10.1186/s13046-017-0664-4
- Zhou, X., Yang, W., & Li, J. (2006). Ca2+- and protein kinase C-dependent signaling pathway for nuclear factor-kappaB activation, inducible nitric-oxide synthase expression, and tumor necrosis factor-alpha production in lipopolysaccharide-stimulated rat peritoneal macrophages. Journal of Biological Chemistry, 281(42), 31337–31347. doi: 10.1074/jbc.M602739200