ABSTRACT
Ricin toxin (RT), extracted from castor bean, is a type II ribosome-inactivating protein (RIP). As a biothreat agent, there is no effective antidote for RT to date, but recent advances in antibody research may have meet this need. This study aimed to produce human single-chain antibody variable fragments (HuscFvs) that bind to and interfere with RT activity for further clinical use. The purified RT component was used in phage biopanning to select ricin-bound HuscFv-displayed phage clones from synthetic (Tomlinson I + J) phage display libraries. The selected HuscFv, named JE11, showed an almost 100% protective effect to HeLa cells by mixing with RT at a 1:1000 ratio in vitro. The antibody showed an 83% protection against injection of 2 × LD50 RT dose mice within 72 h. This research has therapeutic potential for the diagnosis and treatment of RT poisoning.
1. Introduction
Ricin toxin (RT), a product of the castor bean plant (Ricinus communis), is the archetypical type II ribosome-inactivating protein (RIP). RT is considered a potential biological threat agent due to its high availability, ease of production, and lack of any approved medical countermeasure against ricin exposure. The subunits of RT, RTA and RTB, are joined by a single disulfide bond (Lord, Citation1985; Schrot, Weng, & Melzig, Citation2015). Ricin’s enzymatic subunit (RTA) is an RNA N-glycosidase that inactivates eukaryotic ribosomes by catalyzing the hydrolysis of a universally conserved residue within the so-called sarcin/ricin loop (SRL) of 28S rRNA (Endo & Tsurugi, Citation1987; Jolliffe, Craddock, & Frigerio, Citation2005). Ricin’s B subunit (RTB) is a galactose- and N-acetylgalactosamine (Gal/GalNAc)-specific lectin that has two important functions in cytotoxicity.
Currently, the only way to effectively resist ricin poisoning is via passive immunization with neutralizing antibodies at relevant time points after poisoning (Gal et al., Citation2017). After exposure to ricin, the possible antidotes exert their function by preventing ricin from binding to its target (such as sugar analogues) or by inhibiting the catalytic subunit of ricin (such as azidothymidine and antibodies) (Bigalke & Rummel, Citation2005; Shuntao et al., Citation2006). In 2014, Pincus SH studied the role of the antibody (Ab) Fc region in mediating protection against ricin toxicity (Pincus et al., Citation2014). In 2016, Respaud R described the development of an anti-ricin neutralizing monoclonal antibody (IgG 43RCA-G1) and a device for its rapid and effective delivery into the lungs for application in humans (Respaud et al., Citation2016). Although these antibodies may have good therapeutic effects for the treatment of RT poisoning in the experimental stage, human anti-mouse antibody reactions (HAMA) have limited the availability of mouse-derived monoclonal antibodies. The immune response would result in the rapid removal of murine antibodies from human blood, systemic inflammatory effects, and possibly, anaphylaxis, which can sometimes be fatal (Hu, Yin, Chau, Negrych, & Cherwonogrodzky, Citation2012). Numerous clinical studies have confirmed that humanized antibodies are less immunogenic and more therapeutic than murine or chimeric antibodies in humans (Hwang & Foote, Citation2005; Tsurushita, Hinton, & Kumar, Citation2005). Therefore, human antibodies represent the basic direction of current antibody drug development.
In this study, human monoclonal single chain antibodies (HuscFvs) that bound to RT were produced by phage display technology. The produced HuscFv, named JE11, was expressed by E. coli with a non-amber mutation. JE11 showed an almost 100% protective effect to HeLa cells by mixing with RT at a 1:1000 ratio in vitro. The antibody showed an 83% protection against injection of 2 × LD50 RT dose mice within 72 h. A computerized simulation indicated that the HuscFv of the phage-transformed E. coli clone formed a contact interface with both subunits of ricin residues. Thus, this study aimed to produce human single-chain antibody variable fragments (HuscFvs) that neutralized the activity of RT for use in the diagnosis and treatment of RT poisoning.
2. Results
2.1. Purified ricin toxin
SDS-PAGE was performed using RT protein, and a protein concentration of 14.4 mg/ml and a purity of 99% were obtained for RT ().
2.2. Enrichment, isolation, and identification of the high-affinity scFv
The purified RT was used as the antigen, and the scFv phage clones were enriched by binding to the immobilized antigen, followed by elution and repropagation four times. It was necessary to monitor the titers after each round of biopanning, and the results of the enrichment are shown in . After four rounds of panning, the output/input ratio increased to 3.00*10−5, which indicated effective enrichment. The RT-bound phages were used to infect HB2151 E. coli. Among the 184 positive clones, the 20 clones with the highest signals using PBS as the control antigen were found to be RT-specific. By direct colony PCR, 9 clones were positive (935 bp); their representatives are shown in A. The 9 positive clones were analyzed by ELISA. The ELISA results showed that 2 positive clones were specific binding antigens, as shown in B. After sequencing, these clones were found to be the same. The scFv that had the highest ELISA signal was called JE11, and it was used for further study.
Figure 2. (A) Gel electrophoresis of amplicons from PCR analysis of the 20 selected RT-binding clones. (B) ELISA results of the binding of phage antibodies to antigens. Indirect enzyme-linked immunosorbent assay (ELISA) for determining the binding of HuscFvs expressed from various phage-infected E. coli clones to purified RT. E11 and G8 gave significant ELISA signals (OD450 nm) for RT that were greater than two times higher than bovine serum albumin (BSA) (the antigen control).
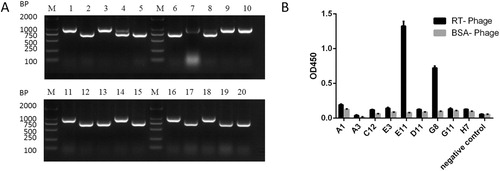
Table 1. Biopanning efficacy of phage libraries against RT.
2.3. Expression and purification of the JE11 scFv
Previous studies have shown that the scFvs expressed by the vector pIT2 in E. coli HB2151 are only secreted in small amounts (Ossysek et al., Citation2015). Therefore, in the current study, the scFv was extracted by acid-ammonium sulfate precipitation from the culture medium. Impurities were removed from the protein preparation by metal chelate chromatography and anion exchange chromatography (A and B). The expressed protein reached a purity of greater than 97% after purification (B).
Figure 3. SDS-PAGE (12%) analysis of fractions collected from each step of the purification process. (A) Metal chelate chromatography. Lane 1: crude extract, lane 2: flowing fluid, lane 3: fraction eluted with 20 mM imidazole, lane 4: fraction eluted with 50 mM imidazole. lane 5: fraction eluted with 100 mM imidazole, lane 6: fraction eluted with 200 mM imidazole, lane 7: fraction eluted with 400 mM imidazole. (B) Anion exchange chromatography. Lane 1: concentration of metal-bonded chromatography products, lane 2: flowing fluid, lane 3: condensed fluid.
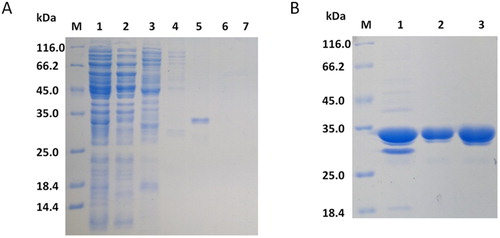
2.4. ELISA and western blot analysis of the scFv
Doses from 0.1 μg/mL to 100 μg/mL of JE11 single-chain antibody were used to detect solid-coated RT, and the ELISA results showed that its binding was dose-dependent (A). The experimental results show that the antibody has a linear range of R2 = 0.9523 from 1 μg/mL to 100 μg/mL and the logarithm of the concentration of the antibody. The EC50 of the antibody was 13.28 μg/mL, compared to the EC50 of the false positive control group is 1.37 μg/mL. Western blot experiment showed that the 10 μg/mL JE11 could specifically bind to RT. The western blot results were the same as the ELISA results (B).
2.5. Cell growth assay
First, we detected whether JE11 was toxic to cells using a cell growth assay. The results showed that JE11 showed no statistically significant growth inhibition on cells at a dose of 50 µg/mL (A).
Figure 5. (A) Cell growth assay to detect the effect of JE11 on HeLa cell growth. (B) Neutralization of ricin toxicity by the JE11 ScFv.
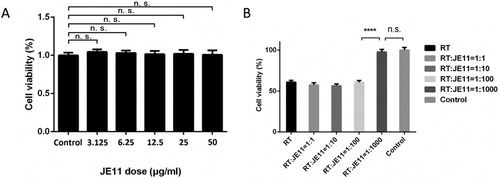
Next, comparative concentrations of RT and JE11 (RT dose: JE11 dose = 1:1, 1:10, 1:100, 1:1000) were added to HeLa cells (5 × 104 per well). After 24 h, the results showed that JE11 could almost 100% neutralize the toxicity of RT when ratio increased to 1:1000 (B).
2.6. Neutralization of mouse lethal activity of RT
We divided 18 mice into 3 groups, and injected intraperitoneally with PBS, 2 × LD50 dose of RT and the preincubated mixtures of RT(2 × LD50) and JE11. As a result, the group injected with the antibody RT mixture showed an 83% survival rate within 72 h, compared with the group in which the RT injection died within 72 h ().
2.7. Residues and regions of RT bound by JE11
The deduced amino acid sequences of JE11 were predicted for immunoglobulin framework regions (FRs) and complementarity determining regions (CDRs) by the International ImMunoGeneTics (IMGT) tool website (www.imgt.org). The deduced HuscFv sequences of JE11 were complete sequences of single-chain antibodies, i.e. each sequence contained 4 FRs and 3 CDRs of the variable heavy chain domain (VH) and variable light chain domain (VL), which were linked by (G4S)3. The sequences had high identity with the human VH and VL sequences in the database.
The DS Protein Docking method, a fast and accurate protein–protein complex structure prediction tool in DS molecular simulation software, consists of three calculation modules: ZDOCK, ZRANK, and RDOCK. The crystal structure of ricin in the PDB protein database (ID: 2vlc) and the scFv’s crystal structure (ID: 6g8r) were used as templates to optimize and construct the three-dimensional structure of ricin and antibodies. First, the ZDOCK method was used to dock the ricin and antibody molecules. The ZDOCK method applied the Fast Fourier transform correlation technique to search for the translational and rotational spaces of the protein–protein system. In the calculation process, the combined conformation was sampled with a Euler angle of 15 degrees, and the final sample number reached 3,600 combined constellations, from which the best docking conformations were output. Then, the optimized structure based on the CHARMm energy minimization method took the top 30 constellations with the highest ZDOCK scores, applied the ZRANK analysis tool, clustered the ZDOCK docking results, determined the complex structure of ricin and the antibody, and applied the RDOCK method to optimize the conformation of the complex and determine the optimal structure of the ricin toxin complex based on the score of the optimized E-Rdock.
The presumptive residues and regions of RT bound to JE11 are shown in and . Interestingly, some of the amino acids of FR3 of the VL also bound to amino acids on the RT surface.
Figure 7. Computerized simulation to reveal the regions of RT bound by HuscFvs. (B) is obtained by shielding JE11 in (A). JE11 formed a contact interface with several residues in both the RTA and RTB domains. The RTA, linker peptide, RTB and JE11 are shown as red, gray, orange and blue, respectively; the interface RT residues are shown as yellow. The details of the interaction are provided in Table 2.
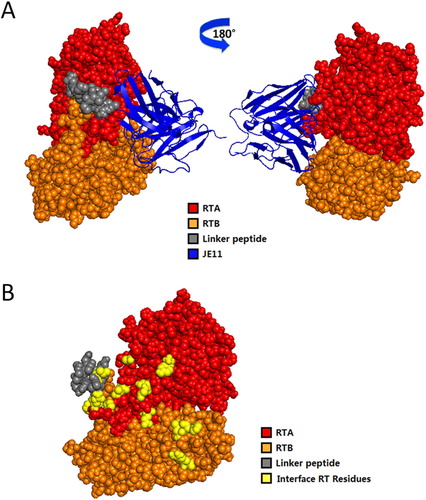
Table 2. Presumptive residues of RT that formed contact interface with JE11.
3. Discussion
Ricin toxin (RT), a product of the castor bean plant (Ricinus communis), is the archetypical type II ribosome-inactivating protein (RIP). Due to the strong toxicity and easy preparation of ricin, it is considered a potential biothreat agent and poses a serious threat to the safety of public society. The toxicity of RT is dose-dependent. High-dose RT is capable of inhibiting protein synthesis. Low-dose RT mainly induces cytokine production and target cell apoptosis and produces lipid peroxidation in vivo.
To date, specific antibody therapy is the most effective treatment for ricin poisoning. After exposure to ricin, antibodies exert their function by preventing ricin from binding its target or by inhibiting the catalytic subunit of ricin (Bigalke & Rummel, Citation2005; Shuntao et al., Citation2006). In recent years, more specific RT antibodies have been prepared, and studies have shown that these antibodies neutralize RT toxicity to a certain degree (Hu et al., Citation2012; Hwang & Foote, Citation2005; Pincus et al., Citation2014; Respaud et al., Citation2016; Tsurushita et al., Citation2005). Despite progress in the experimental stage, these mouse-derived monoclonal antibodies still have a long way to go regarding their use in the clinic due to the limitation of human anti-mouse antibody reactions (HAMA).
To solve this problem, we isolated a human scFv that specifically recognizes and binds RT from Human Single-Fold scFv Libraries I + J. This selection technique offers several advantages over conventional hybridoma methods, including a higher efficiency and a broader spectrum of antigens. The Tomlinson I + J library correlates phenotypes and genotypes using phage display technology in conjunction with antigen recognition and reamplification (Ossysek et al., Citation2015). Human antibodies were obtained after several rounds of biopanning in vitro without human immunization, which avoided human anti-mouse antibody responses and other side effects of heterologous antibodies. This method significantly improves the safety and efficacy of antibodies. The ability of the functional scFvs in the Tomlinson I and J libraries to bind to the metal chelating column by means of the His6 tag significantly contributes to purification, and thus, a large number of antibodies with high purity can be obtained.
However, phage display technology itself has limitations. Firstly, using this technique, it is possible to screen for antibodies that bind to a target antigen, but there is no guarantee that the obtained antibodies will neutralize the active site. The toxicity neutralization of the antibodies displayed in vitro can be explained by steric hindrance or by affecting the separation of RTA from RTB. These mechanisms account for the protective effect of the antibodies in vitro and also explain why the in vitro protection of some antibodies is not significant. To avoid this problem, the storage capacity of the phage library must be as large as possible. Secondly, human antibody libraries that are not immunized may not be as specific for antigen. This may result in lower binding of the antibody obtained by screening, which is also consistent with the experimental results.
In this study, human monoclonal single chain antibodies (HuscFvs) that bound to functionally important residues of RT were produced by phage display technology. A HuscFv of the phage-transformed E. coli clone bound to RT. In this study, we discovered that JE11 had in vitro toxin-neutralized activity in the Cell Growth Assay and can successfully detect RT by ELISA. The antibody showed an 83% protection against injection of 2 × LD50 RT dose mice within 72 h. A computerized simulation indicated that JE11 formed a contact interface with both subunits of ricin residues.
Considering the problem of the low affinity of human antibodies, we will integrate the human antibodies screened in this study and murine monoclonal antibodies obtained in previous studies to prepare humanized chimeric antibodies. Chimeric antibodies minimize the sequence of the murine source and retain the toxicity neutralization of murine monoclonal antibodies. In summary, the study of ricin-antibody interactions is essential for the ongoing development of countermeasures against this toxin.
4. Materials and methods
4.1. Bacterial strains, helper phage, and libraries
Tomlinson (I + J) phage antibody libraries, E. coli strains TG1 and HB2151, and helper phage KM13 were purchased from Source BioScience (Nottingham, United Kingdom).
4.2. Main reagents
aThe C-Myc (9E10) HRP antibody was purchased from Santa Cruz Biotechnology (CA, USA). Sepharose 4B, Sephacryl S-200, Q Sepharose Fast Flow and Chelating Sepharose Fast Flow, and the AKTA Prime chromatography were was purchased from GE Healthcare (Fairfield, CT, USA). The TECAN Infinite F500 microplate reader was purchased from TECAN (Männedorf, Switzerland).
4.3. Preparation of RT
Castor bean powder was suspended in a 10 mM PBS solution (pH = 7.4, 0.2 M NaCl) and allowed to stand at 4°C for 24 h. The suspension was centrifuged at 10,000 rpm/min for 30 min, and the precipitate containing the upper layer of fat was discarded. Solid ammonium sulfate was added to 55% saturation and allowed to stand at 4°C for 4 h. After centrifugation at 10,000 rpm/min for 40 min, the precipitate was dissolved in distilled water, dialyzed overnight in a 10 mM PBS solution (pH = 7.4), and centrifuged at 10,000 rpm/min for 4 min at 4°C. The supernatant was removed and used as the crude extract of RT. The crude RT extract was purified by a Sepharose 4B column and Sephacryl S-200 column and used as the antigen for the next experiment.
4.4. Biopanning and identification of the scFv
The Tomlinson I and J Human Single-Fold scFv libraries were panned on an Immuno Stick (P.O. Box 280, Kamstrupvej 90, Roskilde, DK-4000, DK) separately to identify ricin binders. Each round of panning was completed according to the manufacturer’s instructions. Binding of the identified clones to ricin was assessed by monoclonal phage enzyme-linked immunosorbent assay (ELISA). The VH and VL inserts were amplified from phages showing positive binding to ricin via monoclonal ELISA using vector-specific primers (LMB3: 5′-CAGGAAACAGCTATGAC-3′; pHEN seq: 5′-CTATGCGGCCCCATTCA-3′). The resulting amplicons were examined by electrophoresis on 1% agarose gels and then sequenced by Comate Biosciences Co. (Changchun, China). The nucleotide sequences of the most successful clones were analyzed using the International ImMunoGeneTics (IMGT) tool website (www.imgt.org) to determine the framework and complementary determining regions (CDR) of the VH and VL chains.
4.5. Expression, purification and western blot analysis of the scFv
Expression of the optimal scFv was induced in E. coli HB2151 (containing the pIT2-scFv vector) using IPTG. After shaking overnight at 12 h 20°C, the scFv was extracted using ammonium sulfate and purified by immobilized metal ion affinity chromatography and Q-Sepharose chromatography. The purified scFv was subjected to 12% sodium dodecyl sulfate-polyacrylamide gel electrophoresis (SDS- PAGE) analysis.
4.6. Western blot analysis of the scFv
To identify the combination of the scfv and RT, purified RT was also subjected to 12% SDS-PAGE. Western blot analysis used the scFv as the primary antibody and c-Myc (9E10) HRP as the secondary antibody. 3,3′,5,5′-Tetramethylbenzidine was used as the chromogenic reagent.
4.7. ELISA analysis of the scFv
Each well of a 96-well culture plate was coated with purified RT (10 μg/mL) or PBS (false positive control) and incubated at 4°C overnight. A 3% PBS-BSA solution was added to each well as the blocking solution and incubated at 37°C for 2 h. The wells were then washed with PBS three times before the addition of the purified scFv. Substitution of PBS (pH 7.4) for the primary antibody served as the negative control. Following incubation for 1.5 h at 37°C, the plate was washed three times with 0.05% Tween PBS, and 100 µL of the c-Myc (9E10) HRP antibody (1:2000 dilution) was added to each well. The plate was incubated at 37°C for 1 h and then washed five times with 0.05% Tween PBS. Then, 100 µL of TMB substrate solution was added to each well, and the plate was incubated for 10 min. After 15 min, the enzymatic reaction was terminated by adding 1 M H2SO4, and absorbance was measured at 450 nm using a microplate reader.
4.8. Cell growth assay
The HeLa cells were plated as quadruplicates in 96-well microliter plates (Costar, Cambridge, MA, USA) at a density of 5 × 104 cells/well and then treated with RT and the purified scFv at a dose ratio from 1:1 to 1:1000 at 37°C in a humidified 5% CO2/95% air incubator or as equal volumes of RT without the scFv (negative control). After 24 h, the cells were incubated with MTT at 37°C for 4 h. After removing the supernatant, 150 µL of DMSO (dimethyl sulfoxide) was added to each well, and the mixture was shaken at a low speed for 10 min on a shaker to fully dissolve the crystals. The absorbance of the samples against a background control (medium alone), which was used as a blank, was measured at 490 nm using a microplate reader.
4.9. In vivo protective experiment
Male BALB/c mice(4 weeks of age) used in the experiment were purchased from Shenyang Research Institute of Animal Experiment (Shenyang, China), animal Certificate No.: SCXK (L) 2015-0001. The animals were weighing 22 ± 2 grams and maintained under specific pathogen-free conditions.
4.10. Computerized simulation to predict the RT regions and residues bound by JE11
The nucleotide sequences of the RT-bound HuscFvs were determined by DNA sequencing. The HuscFvs and their complementarity determining regions (CDRs) and the immunoglobulin framework regions (FRs) were predicted using the IMGT software in www.imgt.org. The deduced amino acid sequences of the HuscFvs were submitted to Swiss-Model (swissmodel.expasy.org) online service for protein modelling. The available crystal structures were used as specific templates to guide the Swiss model to model the 3D structure of proteins from the deduced amino acid sequences. The modelled protein structures were subsequently refined by Discovery Studio 4.5 (Acclery, Inc.). The protein structure model was visualized using the Discovery Studio Client, and the molecular interactions were calculated using the ZDOCK algorithm. The results of the molecular docking were analyzed, and some binding configurations for optimization were selected with RDOCK.
4.11. Statistical analysis
One-way ANOVA followed by a post hoc comparison using the least significant difference (LSD) and independent t-test were performed for data comparison using SPSS 11.0 statistical software(IBM, Armonk, NY, USA). All graphical illustrations were generated in Graph Pad Prism 6. Significance was indicated as follows: p ≤ 0.05 (*); p ≤ 0.01 (**); p ≤ 0.001 (***); p ≤ 0.0001 (****).
Disclosure statement
No potential conflict of interest was reported by the authors.
Correction Statement
This article has been republished with minor changes. These changes do not impact the academic content of the article.
Additional information
Funding
References
- Bigalke, H., & Rummel, A. (2005). Medical aspects of toxin weapons. Toxicology, 214, 210–220. doi:10.1016/j.tox.2005.06.015. PMID: 16087285.
- Endo, Y., & Tsurugi, K. (1987). Rna n-glycosidase activity of ricin a-chain. Mechanism of action of the toxic lectin ricin on eukaryotic ribosomes. J. Biol. Chem, 262, 8128–8130. PMID: 3036799.
- Gal, Y., Mazor, O., Falach, R., Sapoznikov, A., Kronman, C., & Sabo, T. (2017). Treatments for pulmonary ricin intoxication: Current aspects and future prospects. Toxins (Basel), 9(10), 311. doi:10.3390/toxins9100311. PMID: 28972558.
- Hu, W.-G., Yin, J., Chau, D., Negrych, L. M., & Cherwonogrodzky, J. W. (2012). Humanization and characterization of an anti-ricin neutralization monoclonal antibody. PLoS One, 7(9), e45595. doi:10.1371/journal.pone.0045595. PMID: 23049820.
- Hwang, W. Y., & Foote, J. (2005). Immunogenicity of engineered antibodies. Methods, 36(1), 3–10. doi:10.1016/j.ymeth.2005.01.001. PMID:15848070.
- Jolliffe, N. A., Craddock, C. P., & Frigerio, L. (2005). Pathways for protein transport to seed storage vacuoles. Biochemical Society Transactions, 33, 1016–1018. doi:10.1042/BST20051016. PMID: 16246035. doi: 10.1042/BST0331016
- Lord, J. M. (1985). Precursors of ricin and ricinus communis agglutinin. Glycosylation and processing during synthesis and intracellular transport. European Journal of Biochemistry, 146(2), 411–416. doi:10.1111/j.1432-1033.1985.tb08667.x. PMID: 3967664.
- Ossysek, K., Uchański, T., Kulesza, M., Bzowska, M., Klaus, T., Woś, K., … Bereta, J. (2015). A new expression vector facilitating production and functional analysis of scFv antibody fragments selected from Tomlinson I + J phagemid libraries. Immunology Letters, 167(2), 95–102. doi:10.1016/j.imlet.2015.07.005. PMID: 26219832.
- Pincus, S. H., Das, A., Song, K., Maresh, G. A., Corti, M., & Berry, J. (2014). Role of fc in antibody-mediated protection from ricin toxin. Toxins, 6, 1512–1525. doi:10.3390/toxins6051512. PMID: 24811206.
- Respaud, R., Marchand, D., Pelat, T., Tchou-Wong, K. M., Roy, C. J., Parent, C., … Heuzé-Vourc’h, N. (2016). Development of a drug delivery system for efficient alveolar delivery of a neutralizing monoclonal antibody to treat pulmonary intoxication to ricin. Journal of Controlled Release, 234, 21–32. doi:10.1016/j.jconrel.2016.05.018. PMID: 27173943.
- Schrot, J., Weng, A., & Melzig, M. F. (2015). Ribosome-inactivating and related proteins. Toxins (Basel), 7(5), 1556–1615. doi:10.3390/toxins7051556. PMID: 26008228.
- Shuntao, W., Jiannan, F., Jianwei, G., Leiming, G., Yan, L., Yingxun, S., … Beifen, S. (2006). A novel designed single domain antibody on 3-D structure of ricin a chain remarkably blocked ricin-induced cytotoxicity. Molecular Immunology, 43, 1912–1919. doi:10.1016/j.molimm.2005.10.012. PMID: 16343623.
- Tsurushita, N., Hinton, P. R., & Kumar, S. (2005). Design of humanized antibodies: From anti-Tac to Zenapax. Methods, 36(1), 69–83. doi:10.1016/j.ymeth.2005.01.007. PMID: 15848076.