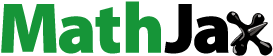
ABSTRACT
The optimum conditions of a direct competitive enzyme-linked immunosorbent assay (dcELISA) in regard to different monoclonal antibodies (MAbs), coating conditions, blocking conditions and physicochemical factors of the assay buffer were investigated to develop a broad-specific and sensitive immunoassay for detection of antibacterial synergists (ASGs) in milk, eggs, chicken and pork. Under optimized conditions, a highly broad-specific and sensitive dcELISA for ASGs was obtained based on 5C4 and hapten-C-HRP, demonstrating a 50% specific binding (IC50) for five ASGs of 0.208–9.24 ng mL−1 with cross-reactivity (CR) of 138.2–7.5%. The optimized dcELISA was used to quantify the five ASGs in milk, egg, chicken muscle and beef with the average recoveries of 79.5–105.4% and coefficients of variation (CV) less than 13.4%. The established method with broad specificity and uniform affinity, offered a simple, sensitive, and high-throughput screening tool for the detection of multi- ASGs in animal-derived food.
1. Introduction
Antibacterial synergists (ASGs), also known as sulfonamide potentiators, are a group of dihydrofolate- reductase inhibitors that function by inhibit bacterial dihydrofolate reductase (Yang et al., Citation2016). To date, the ASG family consists of nine drugs, including trimethoprim (TMP), baquiloprim (BQP), brodimoprim (BDP), ormetoprim (OMP), diaveridine (DVD), tetroxoprim (TXP), metioprim (MTP), aditoprim (ADP), and iclaprim (ICL) (Li et al., Citation2018). ASGs are usually used in combination with sulfonamides (SAs), which inhibit bacterial dihydrofolate synthetase, or other antimicrobials, including β-lactams, and aminoglycosides and fluoroquinolones (Chen et al., Citation2017). The potency of ASGs are enhanced 10× when administered with SAs (Chen, Liu, Song, Kuang, & Xu, Citation2016) and ASGs can also enhance the antibacterial activity of other antimicrobials. Thus, ASGs are widely administered in combination with SAs or other antibiotics for the therapy of animal diseases, including fowl cholera, respiratory tract secondary bacterial infection, and pullorum disease (Choi et al., Citation2012). In China, TMP, OMP and DVD are used as therapeutic agents to cure animal diseases after administration of appropriate preparations. However, the physical and chemical properties of ASGs are stable and thus, the excessive use of ASGs could be concentrated in humans through the food chain, which results in antibiotic-resistance, nausea, emesis, headache, pruritus, and rush in consumers (Ardsoongnearn, Boonbanlu, Kittijaruwattana, & Suntornsuk, Citation2014). Additionally, the long term and excessive use of antibacterial synergists reduces serum leukocyte and thrombocyte and induces teratogenesis (Chen et al., Citation2016). Therefore, to protect human health from these potential risks, the maximum residue limits (MRLs) of ASGs in some food matrices have been established. The MRLs for TMP have been set at 50 μg kg−1 in edible tissues of all food- producing species, including milk, muscle, liver, fat, and kidney in most countries, and the MRL for DVD in chicken is 50 μg kg−1 in Japan. The MRL of BQP has been set at 50 μg kg−1 in porcine liver and 30 μg kg−1 in milk by European Union. In light of its adverse effects on consumer health, it is important to develop an effective and rapid detection method of ASGs that can be used to screen a large number of samples.
At present, instrumental methods are the most commonly reported techniques for the quantification of ASGs, and include gas chromatography and mass spectrometry, high-performance liquid chromatography (Qin, Deng, Su, & Wang, Citation2012) and high-performance liquid chromatography−tandem mass spectrometry (Yang et al., Citation2016). These instrumental methods have high sensitivity and specificity, but requires expensive instruments and professional operators. The sample preparation is relatively complicated and is not suitable for screening large samples. More importantly, most of these instrumental methods are used for the simultaneous detection of TMP (Han et al., Citation2019) and SAs (Li et al., Citation2019) rather than ASGs alone. As an alternative to instrumental methods, antibody-based assays, i.e. immunoassays, are relatively inexpensive and rapid screening methods to detect targets of interest in a variety of samples (Li et al., Citation2019). Now, class-specific immunoassays that can detect one class of analytes in a single run have gained increased attention because of their short assay time, low sample consumption, high sample throughput and reduced detection cost per assay. Until now, extensive effort has been devoted to developing antibodies that are class-specific to chemical contaminants, such as SAs, fluoroquinolones, and organophosphates.
In our previous work, five monoclonal antibodies (mAbs), 3B6, 9C9, 5C4, 14G1 and 1F1 were obtained, and 5C4 showed not only uniform broad specificity but also high affinity to all five ASGs used. An indirect competitive ELISA (icELISA)-based 5C4 was established and exhibited IC50 values of 0.067–0.139 μg L−1 with cross-reactivity of 48.2–418.7% for the five ASGs in buffer under optimal conditions. The calculated limits of detection (LOD) of the icELISA for chicken and milk were 0.06–0.8 μg kg−1 and 0.05–0.6 μg L−1, respectively, with the recoveries of 75.2–101.4% in spiked chicken and milk samples and a coefficient of variation less than 14.3%. However, icELISA requires reporter-labeled secondary antibodies and need multiple washes, compared with icELISA the direct format needs fewer assay steps and then more rapid and convenient for screening of large groups of samples (Bai et al., Citation2015). This has been widely used to detect several analyte classes, such as mycotoxin (Zhang et al., Citation2017), toxins (Kawatsu, Kanki, Harada, & Kumeda, Citation2014), veterinary drugs (Song, Yang, Wang, Si, & Deng, Citation2012; Wang et al., Citation2015), herbicides (Kondo et al., Citation2012), pesticides (Liu et al., Citation2012) and endocrine-disrupting compounds (Zhang et al., Citation2013). Therefore, in the present study, an ultra-high sensitive direct competitive enzyme-linked immunosorbent assay (dcELISA) based on mAbs against ASGs was developed for multi-ASGs detection in different animal-derived food samples.
2. Materials and methods
2.1. Reagents and materials
Materials. TMP, BQP, BDP, OMP and DVD () were purchased from from Dr. Ehrenstorfer GmbH (Ausburg, Germany). mAbs, 3B6, 9C9, 5C4, 14G1 and hapten,4-((4-((2,4-Diaminopyrimidin-5-yl)methyl)-2,6-dimethoxyphenoxy) methyl) benzoic Acid (hapten-C) were acquired from China Agricultural University (Beijing, China), and the specific preparation process was detailed in the previous published articles (Li et al., Citation2018). Horseradish Peroxidase (HRP), bovine serum albumin (BSA), skim milk and Newborn calf serum (NBCS) were supplied by Solarbio (Beijing, China). TMB substrate, Tris and Tween-20 were purchased from Beyotime (Shanghai, China). Normal solvents and NaCl were of analytical reagent grade and were supplied by Laiyang Reagent Corporation (Laiyang, China). Flat-bottomed high-binding polystyrene ELISA plates were obtained from Corning Life Sciences (New York, NY, USA).
Instruments. Values of optical density (OD) or B were measured using ELX-800 Multi-Mode Reader (BioTek, USA). The water used in this study was obtained from a Milli-Q synthesis system (Bedford, MA, USA).
Solutions. The assay buffer was phosphate buffer solution (PBS, 0.01 M, pH 7.4), and prepared by dissolving 8.5 g of NaCl, 0.2 g of KCl, 2.9 g of Na2HPO4·12H2O and 0.593 g of NaH2PO4·2H2O in 1 L of purified water. The coating buffer (CB, 0.05 M, pH 9.6) was prepared by dissolving 2.93 g of NaHCO3 and 1.59 g of Na2CO3 in 1 L of purified water. The blocking buffer was 2% skim milk (w/v). The washing buffer (PBST) contained 0.05% Tween-20 (v/v) in 0.01 M PBS. The substrate solution consisted of solutions A and B at the ratio of 1:1 (v/v). The stop solution was 2 M sulphuric acid. Before experiments, standard solutions of ASGs in the range 0.0064–100 ng mL−1 were freshly prepared by dilution of stock solution with PBS.
2.2. Development of a direct competitive ELISA
2.2.1. Synthesis of hapten-C-HRP conjugates
Hapten-C (), contains carboxylic acid group, which was coupled to HRP using the active ester method described. First, 20 mg of hapten-C was dissolved in 0.6 mL of DMF with the addition of NHS (10 mg) and DCC (20 mg). The mixture was reacted using a magnetic stirrer at 4 °C for 5 h followed by the removal of the precipitates. The supernatant of hapten-C was dropwise added into dissolved HRP solution (10 mg of HRP in 2 ml of carbonate buffer, pH 9.6). The solutions containing the hapten-C-HRP conjugates were stirred for 12 h and then dialyzed in PBS for 2 days.
2.2.2. dcELISA procedure
The dcELISA was performed in 96-well polystyrene titration plates, the specificity of 3B6, 9C9, 5C4 and 14G1 mAbs were determined by dcELISA using HRP as a detection marker. Briefly, the ELISA plates were coated with coating antibodies (100 µL well−1) which was diluted with CB buffer and then incubated at 37°C for 2 h. After washing one time with PBST, 150 µL well−1 blocking buffer was added to the plates and incubated at 37°C for 1 h. The blocking buffer in the ELISA plates was then discarded. 50 µL of standards and 50 µL of hapten-C-HRP were added and incubated for 15 min at 37°C. Then the plate was rinsed with PBST for four times and incubated with 100 µL of substrate solution for 30 min at 37°C. Finally 2 M H2SO4 (50 µL well−1) was used to stop the enzymatic reaction. The optical density (OD) values were measured by micro-plate detector at 450 nm. Concentrations were then determined from the standard curve using semi-logarithmic graph paper. The standard curves were fitted using the following equation:(1)
(1)
Where A = response at high asymptote, D = response at low asymptote, X = calibration concentration, C = IC50, and B = slope. The cross-reactivity (CR) of each ASG was calculated based on the IC50 values according to the subsequent formula:(2)
(2)
2.2.3. Optimization of dcELISA
Many factors can influence the performance of an immunoassay. Aiming to improve the performance of dcELISA, the concentration of coating antibody and hapten-C-HRP, blocking conditions (blocking buffer, blocking time), several physicochemical factors of the assay buffer (detergent, pH, NaCl and organic solvent), reaction time and colour development time were examined. In the ELISA optimization, we used TMP as a reference analyte to construct standard curves.
2.3. Analysis of samples
2.3.1 Matrix effect
The optimized dcELISA was applied to whole milk (fat content 3.6%), skim milk (no fat), eggs, chicken and pork. All these negative samples were provided by the National Veterinary Drug Safety Evaluation Center (Beijing, China) and kept frozen until use. Before recovery study, the effect of animal-derived food matrix on the performance of dcELISA was firstly evaluated by comparing the standard curve of TMP obtained in assay buffer with those in diluted sample extract.
Whole milk and skim milk: The whole milk samples were degreased by centrifugation for 20 min at 8000 g and then the upper fat layer was discarded. The skim milk was directly diluted 5, 10, 15, 20 and 25 times with assay buffer, respectively. The liquid layer was diluted 0-, 5-, 10-, and 50-fold with assay buffer analysis.
Eggs: One gram of egg samples were weighed into a 50 mL centrifuge tube, then diluted with 5 mL assay buffer. The mixture was vortex mixed for 1 min, shaken for 10 min and then centrifuged for 15 min at 5000 g. The upper layer was filtered with filter paper, and then diluted 3-, 6- and 12- fold respectively with assay buffer for dcELISA.
Chicken and pork: The edible animal tissue samples including chicken and pork were minced and finely homogenized (Ni et al., Citation2019). One gram of chicken or pork were weighed into 50 mL centrifuge tubes and then 5 mL of assay buffer containing 0.1% BSA was added. The mixture was vortexed for approximately 2 min followed by centrifuging for 15 min at 10000 g. Finally, the supernatant liquid layer was separated for the dcELISA.
2.3.2. Recovery
For recovery studies, whole milk samples were fortified with five analytes, TMP, BQP, BDP, OMP and DVD at 0.45, 0.75 and 1.00 ng mL−1 respectively; skim milk samples were spiked with these five ASGs at 0.50, 1.50 and 4.00 ng mL−1 respectively; egg samples were spiked with these five ASGs at 0.30, 0.60 and 1.20 ng g−1; chicken samples were spiked with these five ASGs at 0.50, 1.00 and 2.50 ng g−1; and pork samples were spiked with these five ASGs at 0.10, 0.25 and 1.00 ng g−1. OD values or B determined at each concentration by the optimized dcELISA were interpolated with a standard curve prepared in assay buffer with each data point being an average of four replicates.
3. Results and Discussion
3.1. dcELISA optimization
The effect of these parameters were determined by measuring the maximum absorbance (Amax, the absorbance value at zero analyte concentration) and IC50 Values. In this study, the IC50/ Amax ratio was used as the primary criteria to evaluate the immunoassay performance and the lowest ratio value indicated the highest sensitivity.
Dilutions of coating antibodies and hapten-C-HRP. The checkerboard assays were used to study the effect of different dilutions of hapten-C-HRP and coating antibodies (3B6, 9C9, 5C4, 14G1) on the Amax and IC50 of dcELISA. As can be observed in Table S1-S4, the results showed that the optimal 3B6 and hapten-C-HRP dilution were at 1:1000 and 1:12500 respectively at which the Amax of the dcELISA was 1.253 and IC50/ Amax, 0.141, was the lowest; the optimal 9C9 and hapten-C-HRP dilution were at 1:500 and 1:62500 respectively at which the Amax of the dc-ELISA was 1.182 and IC50/ Amax, 0.186, was the lowest; the optimal 5C4 and hapten-C-HRP dilution were at 1:1000 and 1:62500 respectively at which the Amax of the dcELISA was 1.187 and IC50/ Amax, 0.079, was the lowest; the optimal 14G1 and hapten-C-HRP dilution were at 1:1000 and 1:62500 respectively at which the Amax of the dcELISA was 1.202 and IC50/ Amax, 0.106, was the lowest. The above results indicated that the four antibodies could bind with the hapten-C-HRP with varied affinities, which also demonstrated that hapten-C has successfully linked to HRP.
Coating and blocking conditions. As shown in (a), the influence of coating conditions on the four MAbs followed similar tendencies. Of all coating conditions evaluated, the lowest IC50/ Amax ratio was obtained at 25 °C for 2 h for all the four antibodies. The choice of blocking buffer was sometimes critical in optimizing assay sensitivity. In this study, three typical blocking buffers, 2% skim milk, 5% calf serum and 1% BSA were evaluated for the dcELISA (b). Unlike the influence of coating conditions, substantial differences were observed among the performance of the four MAbs. The lowest IC50/ Amax ratios, 0.192, 0.301 and 0.402 for 3B6, 5C4 and 14G1 respectively were obtained using 1% BSA as blocking buffer, whereas the lowest IC50/ Amax ratio, 0.214, for 9C9 was obtained using 2% skim milk. The effects of blocking time, 1, 2 and 3 h, on the assay performance were presented in (c). Obviously, blocking time of 3 h for 3B6 and 14G1, with the lowest IC50/ Amax of respectively was optical. However, the lowest IC50/ Amax ratios of for 9C9 and 5C4 respectively were observed with blocking time of 1 h.
Figure 2. Effects of physicochemical factors on analytical characteristics of the competitive standard curve for TMP based on mAb 3B6, 9C9, 5C4 and 14G1 in dcELISA. (a) coating condition; (b) blocking buffer; (c) blocking time; (d) concentration of Tween-20; (e) concentration of BSA; (f) concentration of skim milk; (g) pH; (h) concentration of NaCl; (i) methanol; (j) acetonitrile; (k) reaction time; (l) colour development time.
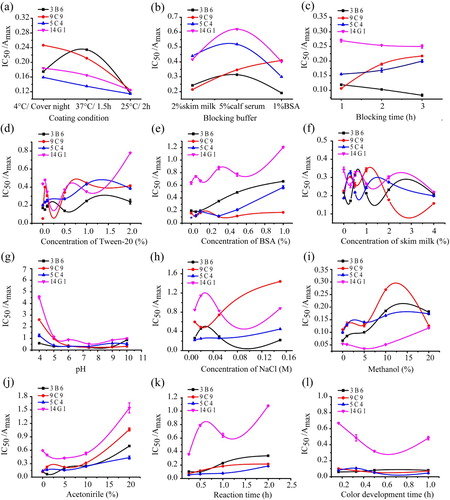
Reaction conditions. The reaction conditions of dcELISA might have a direct effect on the sensitivity of the assay. Therefore, the optimum reaction conditions of dcELISA in regard to concentrations of Tween-20, BSA, skim milk and NaCl in assay buffer, pH, solvent, reaction time and colour development time were investigated to develop a broad-specific and sensitive immunoassay for detection of ASGs in animal-derived food.
The inclusion of detergents in assay buffers for the reduction of nonspecific interactions is a very common practice when developing immunoassays, and Tween-20 is perhaps the most extensively used detergent. As shown in (d), the lowest IC50/ Amax ratio for MAb 14G1 was obtained in the presence of 0.1% Tween-20, indicating that the detergent could reduce nonspecific binding and improve assay sensitivity. However, the lowest IC50/ Amax ratios for MAb 3B6, 9C9 and 5C4 were obtained without Tween-20 in the assay buffer (d). Based on the IC50/ Amax ratios, 0.1% Tween-20 were added to the assay buffer for MAb 14G1 and assay buffers without Tween-20 were used for MAb 3B6, 9C9 and 5C4. In addition, sometimes, BSA instead of Tween-20 included in assay buffer could obtain satisfactory result. Skimmed milk is usually added to the assay buffer to enhance the sensitivity, reduce well to well variability, or to lower background for immunoassays (Watanabe et al., Citation2002). Therefore, their influence on AGSs immunoassay was also studied in this work. As shown in (e) and (f), BSA and skim milk do have effects on AGSs assay. That was, the lowest IC50/ Amax ratios for MAb 3B6, 9C9, 5C4 and 14G1 were obtained with 0.05%, 0.3%, 0% and 0% BSA respectively added in assay buffer; the lowest IC50/ Amax ratios for MAb 3B6, 9C9, 5C4 and 14G1 were obtained with 0.3%, 4%, 0% and 4% skim milk added in assay buffer respectively. Thus, 0.05% BSA and 0.3% skim milk, 0.3% BSA and 4% skim milk, 0% BSA and 0% skim milk, and 0% BSA and 4% skim milk were added into the assay buffer for dcELISA based on MAb 3B6, 9C9, 5C4 and 14G1 respectively.
In case of pH, of all pH values evaluated in the range from 4 to 10, the lowest IC50 /Amax ratio was obtained at pH 7.4 of the assay buffer for all four MAbs. As shown in (g), slight differences in assay buffer pH would not severely affect MAb performance. pH 7.4 were chosen for all four MAbs as the optimum assay buffer pH. As to immunoassay, the key binding step depends mainly on van der waals forces and hydrophobic interactions, which were affected by the pH and the ionic strength (Zhang, Liu, Zhuang, & Hu, Citation2012). After choosing the optimal pH of the assay buffer, the influence of the different ionic strength of assay buffer solution for our determination was studied; we changed the concentration of NaCl in assay buffer solution (such as 0.025, 0.05 and 0.15 M). A plot of the ratio IC50/ Amax as a function of ionic strength was depicted in (h). Interestingly, substantial differences were observed among the performance of MAbs 3B6, 5C4, 9C9 and 14G1. As observed, as the concentration of NaCl began to increase, the IC50/ Amax ratio was initially decreased and then increased as the NaCl increased for MAbs 3B6 and 14G1, while IC50/ Amax ratio was increased with the increasing of NaCl for MAbs 3B6 and 14G1. The optimum concentration of NaCl was selected as 0.01 M for MAb 3B6, 5C4, and 14G1, and 0.02 M for MAb 9C9, which led to the lowest IC50/ Amax. The above results suggest that variable NaCl concentrations may interfere with the MAbs performance and there were different effects for different MAbs. Moreover, enhanced hydrophobic interactions could significantly affect or even completely inhibit both antibody/antigen interaction and enzyme activity.
The use of organic solvents in the ELISA assay must be tested since the extraction procedure is often carried out in organic medium and antibodies, generally, are not very tolerant to them (Chafer-Pericas, Maquieira, Puchades, Miralles, & Moreno, Citation2010). Several different concentrations of methanol and acetonitrile were used to prepare different assay buffers for testing. (i) showed the evolution of IC50/ Amax ratios with methanol percentage, a better tolerated organic solvent for all four MAbs. The results showed that the IC50/ Amax ratios were increased sharply at methanol concentrations greater than 5%. As shown in (j), a clear increase of IC50/ Amax ratios for all four MAbs were observed when the amounts of acetonitrile were increased in the assay buffer and the IC50/ Amax ratios were also increased sharply at acetonitrile concentrations greater than 5% for all four MAbs. On the basis of these results, and the fact that methanol and acetonitrile were commonly used to extract AGSs from food matrices, methanol and acetonitrile were chosen as the extraction solvent and the concentrations of methanol and acetonitrile were reduced to 5% or lower by dilution with assay buffer prior to dcELISA analysis.
With the optimal coating, blocking and assay buffer conditions, the reaction time of MAbs with hapten-C or antigen have been investigated. As shown in (k), the reaction time was 15 min for all four MAbs when the lowest IC50/ Amax ratios and a higher sensitivity were obtained. In addition, we also investigated the colour development times (such as 10, 20, 30 and 60 min), and the results indicated that a colour development time of 10 min was suitable for MAb 3B6, while 30 min was suitable for MAbs 9C9, 5C4 and 14G1((l)). Finally, the main parameters used in dcELISA for the four MAbs 3B6, 9C9, 5C4 and 14G1 were listed in Table S5.
3.2. Sensitivity and cross-reactivity of the dcELISA
Under the optimum conditions of the dcELISA based on MAb 3B6, 9C9, 5C4 and 14G1 respectively, the sensitivity and specificity for the four MAbs were evaluated by performing competitive assays for 5 AGSs, TMP, BQP, BDP, OMP and DVD, the obtained IC50 values were used to calculate the CRs. (a–d) shows standard curves, based on MAbs 3B6, 9C9, 5C4 and 14G1 respectively, for the five ASGs and the all IC50 values and the CRs were summarized in . All data showed that in the assay based on 3B6, the IC50 values for TMP, BQP, BDP, OMP and DVD were 0.35, 0.22, 5.37, 9.24 and 0.96 ng mL−1, respectively; in the assay based on 9C9, the IC50 values for TMP, BQP, BDP, OMP and DVD were 0.51, 0.22, 0.22, 5.97 and 0.98 ng mL−1, respectively; in the assay based on 5C4 the IC50 values for TMP, BQP, BDP, OMP and DVD were 0.26, 0.18, 0.20, 3.49 and 0.31 ng mL−1, respectively; in the assay based on 14G1 the IC50 values for TMP, BQP, BDP, OMP and DVD were 1.35, 0.75, 1.17, 7.31 and 0.67 ng mL−1, respectively. Compared the four dcELISAs based on MAbs 3B6, 9C9, 5C4 and 14G1 respectively, the sensitivity of the assay using MAb 5C4 was the highest. Moreover, the MAb 5C4 recognized all ASGs (TMP, BQP, BDP, OMP and DVD) with similar high affinity (TMP, 100%; BQP, 138.2%; BDP, 125.9%; OMP, 7.5%; DVD, 83.87%) and appeared to be a more suitable antibody than the other three MAbs to be used for a multi-ASGs screening assay test, which was in agreement with our previous works (Li et al., Citation2018). The results also indicated that the key reagent in immunoassays was the antibody, and the quality of this antibody determines the immunoassay performance. The IC50 values of dcELISA we developed were 3.88-, 4.08-, 11.88-, 31.16- and 1.36-fold higher than IC50 values of icELISA based on 5C4 developed in our previous works. However, the dcELISA based on 5C4 developed in this study was more cost effective and time saving for multi-ASGs in animal-derived food. Thus, the loss in assay sensitivity was acceptable. Taking into account all these factors, dcELISA based on 5C4 developed in this study appeared to be a more suitable method than icELISA based on 5C4 developed in our previous works to be used for a multi-ASGs screening assay test.
Figure 3. Cross-reactivity (CR) for five ASGs under optimized conditions in dcELISA. (a) 3B6; (b) 9C9; (c) 5C4; (d) 14G1
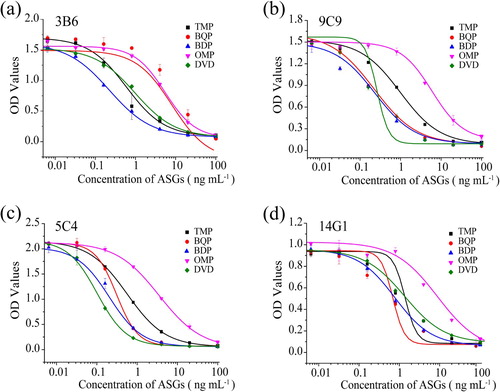
Table 1. Cross-reactivity (CR) for five ASGs under optimized conditions in dcELISA.
3.3. Analysis of samples by dcELISA
Some compounds in animal tissues, such as protein and fat, might affect the binding of antibody and antigen, as well as other aspects of the assay, which is the so-called matrix effect (Wang, Wang, Zhang, Liu, & Zhang, Citation2008). Matrix effects are a common challenge for immunoassays in food analysis and can be removed in a number of ways. Dilution is a commonly adopted and effective method to remove matrix effects (Liu, Fang, Zhang, Zheng, & Wang, Citation2009). To study possible matrix effects from milk, egg, chicken and pork, standard curves were prepared with these samples and with assay buffer as a control. Different dilution-folds with different dilution buffers were tested for reducing matrix effects.
Whole milk and skim milk: milk is a complex system consisting of a large amount of proteins and fat, which can affect the immunoassay results (Zvereva et al., Citation2018). In this study, various dilution ratios, such as 5, 10, 50 and 100 folds with assay buffer, were used to reduce the matrix effects. As shown in (a) and (b), standard curves produced in whole milk and skim milk after 5-fold dilution and 10-fold dilution with assay buffer respectively were superimposed, indicating that the effect of the matrix was not significant. The accuracy and precision of the assay, represented by recovery and coefficient of variation (CV), were assessed by the five ASGs. Recoveries in whole milk were observed in the range of 83.1% to 100.2% with the CV lower than 12.1%. The recoveries ranged from 79.6% to 105.4% with a CV lower than 11.6% in skim milk ().
Figure 4. The dcELISA calibration curves for TMP in assay buffer and assay buffe diluted samples of (a) whole milk, (b) skim milk, (c) eggs, (d) chicken, (e) pork. Parallels were found between the standard curve prepared in assay buffer and diluted samples, respectively (n = 3).
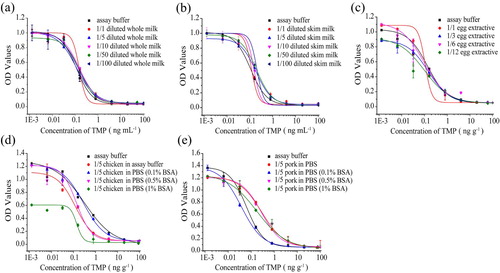
Table 2. The recovery and CV of five ASGs from spiked samples using the dcELISA. (n = 3).
Eggs: according to previous studies, egg samples are often extracted with ethyl acetate and acetonitrile. However, in this study, when ethyl acetate and acetonitrile were used to extract ASGs from egg samples, the recoveries were lower than 60% and OD values decreased sharply. Therefore, assay buffer was used to dissolve egg and matrix effects of eggs were avoided by 6-fold dilution using assay buffer (c). The obtained recoveries were ranging from 87.5%∼104.5% with the CV lower than 13.1% ().
Chicken and pork: in case of chicken and pork, dilution of chicken and pork extract with assay buffer did not eliminate matrix effects in our study. Thus, 0.1%, 0.5% and 1% BSA were added to assay buffer for correcting the matrix interferences respectively. Standard curves produced in chicken and pork after 5-fold dilution with the assay buffer (containing 0.1% BSA) respectively were superimposed, indicating that the matrix interferences could be corrected by 0.1% BSA ((c) and (e)). The recoveries ranged from 82.7% to 101.8% with a CV lower than 13.4% in chicken samples. The recoveries were between 79.5% and 104.1% with the CV lower than 10.4% in pork samples. The results above confirmed that the dcELISA based on MAb 5C4 was ultrasensitive, class-selective, cost effective, time saving and reliable for screening of ASGs in milk, eggs, chicken and pork.
4. Conclusion
In this study, we have developed a highly sensitive, class-selective, cost effective, time saving and reliable dcELISA for screening of multi-ASGs for the first time. The sensitivity and selectivity of the developed dcELISA were significantly improved by optimizing some physical and chemical conditions, which showed IC50 values for all five ASGs tested in assay buffer ranging from 0.208–9.24 ng mL−1. This method could be utilized in the detection of ASGs in whole milk, skim milk, eggs, chicken and pork. Dilution of the samples with assay buffer or assay buffer containing 0.1% BSA was an effective means of reducing the matrix effect, and furthermore, the recoveries and CVs were also satisfactory indicating that the final developed dcELISA is a good screening method for multi-ASGs detection in animal-derived food.
Supplemental Material
Download MS Word (41 KB)Disclosure statement
No potential conflict of interest was reported by the authors.
Additional information
Funding
References
- Ardsoongnearn, C., Boonbanlu, O., Kittijaruwattana, S., & Suntornsuk, L. (2014). Liquid chromatography and ion trap mass spectrometry for simultaneous and multiclass analysis of antimicrobial residues in feed water. Journal of Chromatography B-Analytical Technologies in the Biomedical and Life Sciences, 945-946, 31–38. doi: 10.1016/j.jchromb.2013.11.034
- Bai, Y. H., Liu, Z. H., Wang, H. J., Bi, Y. F., Huang, Y. L., Sun, L., … Xu, S. X. (2015). Development of a rapid and sensitive enzyme-linked immunosorbent assay to detect clonidine residues in swine urine samples. Food and Agricultural Immunology, 26(1), 1–12. doi: 10.1080/09540105.2013.858663
- Chafer-Pericas, C., Maquieira, A., Puchades, R., Miralles, J., & Moreno, A. (2010). Fast screening immunoassay of sulfonamides in commercial fish samples. Analytical and Bioanalytical Chemistry, 396(2), 911–921. doi: 10.1007/s00216-009-3229-3
- Chen, Y. N., Liu, L. Q., Song, S. S., Kuang, H., & Xu, C. L. (2016). Establishment of a monoclonal antibody-based indirect enzyme-linked immunosorbent assay for the detection of trimethoprim residues in milk, honey, and fish samples. Food and Agricultural Immunology, 27(6), 830–840. doi: 10.1080/09540105.2016.1183599
- Chen, Y. N., Liu, L. Q., Xie, Z. J., Zhu, J. P., Song, S. S., & Kuang, H. (2017). Gold immunochromatographic assay for trimethoprim in milk and honey samples based on a heterogenous monoclonal antibody. Food and Agricultural Immunology, 28(6), 1046–1057. doi: 10.1080/09540105.2017.1325843
- Choi, M. J., Yohannes, S. B., Lee, S. J., Damte, D., Reza, M. A., Rhee, M. H., … Park, S. C. (2012). The in vitro antibacterial activity of enrofloxacin-trimethoprim combination against five bacterial species. Pakistan Veterinary Journal, 32(3), 363–366.
- Han, X. Y., Sheng, F., Kong, D. X., Wang, Y. L., Pan, Y. H., Chen, M., … Peng, D. P. (2019). Broad-spectrum monoclonal antibody and a sensitive multi-residue indirect competitive enzyme-linked immunosorbent assay for the antibacterial synergists in samples of animal origin. Food Chemistry, 280, 20–26. doi: 10.1016/j.foodchem.2018.12.040
- Kawatsu, K., Kanki, M., Harada, T., & Kumeda, Y. (2014). A highly rapid and simple competitive enzyme-linked immunosorbent assay for monitoring paralytic shellfish poisoning toxins in shellfish. Food Chemistry, 162, 94–98. doi: 10.1016/j.foodchem.2014.04.038
- Kondo, M., Tsuzuki, K., Hamada, H., Yamaguchi, Y., Uchigashima, M., Saka, M., … Miyake, S. (2012). Development of an enzyme-linked immunosorbent assay (ELISA) for residue analysis of the fungicide azoxystrobin in agricultural products. Journal of Agricultural and Food Chemistry, 60(4), 904–911. doi: 10.1021/jf203534n
- Li, C. L., Liang, X., Wen, K., Li, Y. H., Zhang, X. Y., Ma, M. F., … Wang, Z. H. (2019). Class-specific monoclonal antibodies and dihydropteroate synthase in bioassays used for the detection of sulfonamides: Structural insights into recognition diversity. Analytical Chemistry, 91(3), 2392–2400. doi: 10.1021/acs.analchem.8b05174
- Li, C., Luo, X., Li, Y., Yang, H., Liang, X., Wen, K., … Wang, Z. (2019). A class-selective immunoassay for sulfonamides residue detection in milk using a superior polyclonal antibody with broad specificity and highly uniform affinity. Molecules, 24(3), 15–30.
- Li, H. F., Zhang, X. Y., Li, C. L., Dong, B. L., Mujtaba, M. G., Wei, Y. J., … Wang, Z. H. (2018). Generic hapten synthesis, broad-specificity monoclonal antibodies preparation, and ultrasensitive ELISA for five antibacterial synergists in chicken and milk. Journal of Agricultural and Food Chemistry, 66(42), 11170–11179. doi: 10.1021/acs.jafc.8b03834
- Liu, B., Ge, Y., Zhang, Y., Song, Y., Lv, Y. Y., Wang, X. X., & Wang, S. (2012). Production of the class-specific antibody and development of direct competitive ELISA for multi-residue detection of organophosphorus pesticides. Food and Agricultural Immunology, 23(2), 157–168. doi: 10.1080/09540105.2011.608120
- Liu, J., Fang, G., Zhang, Y., Zheng, W., & Wang, S. (2009). Development of a chemiluminescent enzyme-linked immunosorbent assay for five sulfonamide residues in chicken muscle and pig muscle. Journal of the Science of Food and Agriculture, 89(1), 80–87. doi: 10.1002/jsfa.3413
- Ni, T. T., Peng, D. P., Wang, Y. X., Pan, Y. H., Xie, S. Y., Chen, D. M., … Yuan, Z. H. (2019). Development of a broad-spectrum monoclonal antibody-based indirect competitive enzyme-linked immunosorbent assay for the multi-residue detection of avermectins in edible animal tissues and milk. Food Chemistry, 286, 234–240. doi: 10.1016/j.foodchem.2019.02.011
- Qin, S. L., Deng, S., Su, L. Q., & Wang, P. (2012). Simultaneous determination of five sulfonamides in wastewater using group-selective molecularly imprinted solid-phase extraction coupled with HPLC-DAD. Analytical Methods, 4(12), 4278–4283. doi: 10.1039/c2ay26091c
- Song, J., Yang, H., Wang, Y. Z., Si, W. H., & Deng, A. P. (2012). Direct detection of 3-amino-5-methylmorpholino-2-oxazolidinone (AMOZ) in food samples without derivatisation step by a sensitive and specific monoclonal antibody based ELISA. Food Chemistry, 135(3), 1330–1336. doi: 10.1016/j.foodchem.2012.05.107
- Wang, S., Liu, J. H., Yong, W., Chen, Q. L., Zhang, L. Y., Dong, Y. Y., ... Tan, T. W. (2015). A direct competitive assay–based aptasensor for sensitive determination of tetracycline residue in Honey. Talanta, 131, 562–569. doi: 10.1016/j.talanta.2014.08.028
- Wang, L., Wang, S., Zhang, J., Liu, J., & Zhang, Y. (2008). Enzyme-linked immunosorbent assay and colloidal gold immunoassay for sulphamethazine residues in edible animal foods: Investigation of the effects of the analytical conditions and the sample matrix on assay performance. Analytical and Bioanalytical Chemistry, 390(6), 1619–1627. doi: 10.1007/s00216-008-1836-z
- Watanabe, E., Kanzaki, Y., Tokumoto, H., Hoshino, R., Kubo, H., & Nakazawa, H. (2002). Enzyme-linked immunosorbent assay based on a polyclonal antibody for the detection of the insecticide fenitrothion. Evaluation of antiserum and application to the analysis of water samples. Journal of Agricultural and Food Chemistry, 50, 53–58. doi: 10.1021/jf0108359
- Yang, Y. J., Liu, X. W., Li, B., Li, S. H., Kong, X. J., Qin, Z., & Li, J. Y. (2016). Simultaneous determination of diaveridine, trimethoprim and ormetoprim in feed using high performance liquid chromatography tandem mass spectrometry. Food Chemistry, 212, 358–366. doi: 10.1016/j.foodchem.2016.05.184
- Zhang, M. C., Liu, S. H., Zhuang, H. S., & Hu, Y. R. (2012). Determination of dimethyl phthalate in environment water samples by a highly sensitive indirect competitive ELISA. Applied Biochemistry and Biotechnology, 166(2), 436–445. doi: 10.1007/s12010-011-9439-0
- Zhang, Y., Gao, A. H., Liu, B., Sheng, W., Tan, C., Yuan, M., & Wang, S. (2013). A direct competitive enzyme-linked immunosorbent assay for rapid detection of anilofos residues in agricultural products and environmental samples. Journal of Environmental Science and Health Part B-Pesticides Food Contaminants and Agricultural Wastes, 48(1), 1–8.
- Zhang, Y., Yang, J., Lu, Y., Ma, D. Y., Qi, M. G., & Wang, S. (2017). A competitive direct enzyme-linked immunosorbent assay for the rapid detection of deoxynivalenol: Development and application in agricultural products and feedstuff. Food and Agricultural Immunology, 28(3), 516–527. doi: 10.1080/09540105.2017.1306491
- Zvereva, E. A., Zherdev, A. V., Formanovsky, A. A., Abuknesha, R. A., Eremin, S. A., & Dzantiev, B. B. (2018). Fluorescence polarization immunoassay of colchicine. Journal of Pharmaceutical and Biomedical Analysis, 159, 326–330. doi: 10.1016/j.jpba.2018.07.008