ABSTRACT
Food contamination with antibiotic residues has become a worldwide problem due to the heavy and improper use of antibiotics. The development of fast, high-throughput, easy, and economical screening methods for the detection of antibiotic residues is an important requirement as an alternative to the traditional assays. Immunoassays are widespread promising methods that can achieve the conditions of an analytical method in the evaluation of food and environmental protection. This review offers an overview of the preparation of antibodies and the currently established immune-assays for the antibiotic residues detection, including enzyme-linked immunosorbent assay, fluorescence immunoassay, radioimmunoassay, colloidal gold immunoassay, and chemiluminescence immunoassay. Furthermore, it will elaborate on the future perspective on the performance and improvement of these assays for the determination of antibiotic residues in food samples. These assays include a simple sample preparation and high-sensitive detection that offer a significant assurance for the screening of antibiotic residues.
GRAPHICAL ABSTRACT
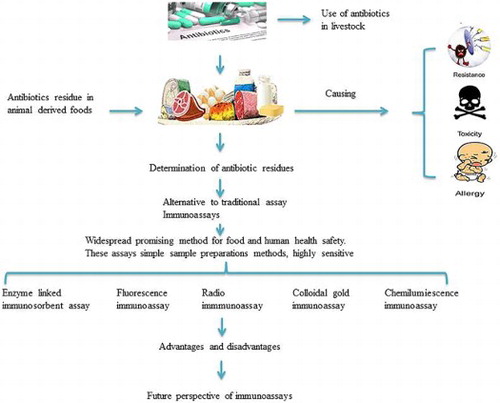
1. Introduction
Antibiotics are widely used in the prevention and treatment of animal diseases. However, the remaining residues in animal-derived food related to the improper or heavy use of antibiotics are harmful to food safety and human health. Thus, countries all over the world assign great significance to antibiotic residue screening. In the development of animal feeding, production, and sale, a range of appropriate rules and regulations are settled to standardize the use of antibiotics and related residues. Screening of these residues requires a simple, rapid, accurate, and sensitive method for a safe and operative guarantee of food safety and human health (Ahmed, Ning et al., Citation2017).
Immunoassays are greatly selective biochemical methods which are based on the specific antigen–antibody interaction (Zhang & Cheng, Citation2017). Currently, immunoassays increase in the sensitivity by labelling one of the reagents: either the antigen or antibody. The labelling agents can be a radioisotope, enzyme, fluorescence, or chemical (Mitchell, Griffiths, McEwen, McNab, & Yee, Citation1998). The reaction is catalyzed by labelling enzymes and substrate degradation to form the coloured product for visual and spectrophotometrical observation (Cullor, Citation1993; Cullor et al., Citation1992). Regarding the detection label, immunoassays applied to antibiotic determination have been classified. Numerous immunoassays have been developed for the screening of antibiotic residues (Ahmed et al., Citation2017; Cao, Yang, et al., Citation2013; Cháfer-Pericás, Maquieira, Puchades, Miralles, & Moreno, Citation2011). Numerous screening assays generally take a pretty long reaction time. So, there is a growing need to introduce the immunoassays developed for the screening of antibiotic residues. Many of the immunochemical tests are commercially available in a kit format such as a card format and one step strip test for many drugs. Previously, a dipstick format (lateral flow devices) had been used as a rapid antibiotic residue-finding tool (Van Herwijnen & Baumgartner, Citation2006). Immunoassays are sensitive, class-specific, and accurate and can provide a means for rapid and efficient screening of the samples for antibiotic residue detection (NaVrátiloVá, Citation2008). Formerly, a study had been conducted for the determination of antibiotic residues in a fish and feed sample to compare different modes of detection immunoassay and Liquid Chromatography with tandem mass spectrometry LC-MS-MS. The achieved limit of detections (LODs) by the immunoassay were lower than those of LC-MS-MS (Cháfer-Pericás, Maquieira et al., Citation2011).
Currently, the immunoassay of antibiotic residue detection is convenient, fast and some can achieve high throughput, while the methods have some of the limitations such as fewer numbers of drug detection, expensive instruments, time-consuming, and so on. This review offers an overview of currently developed immune-based screening assays for the antibiotic residues detection in food samples to ensure the safety of public health and animal-derived foods. The specific main focus is on the currently developed immune-based screening assays for the antibiotic residue detection, such as enzyme-linked immunosorbent assay (ELISA), chemiluminescence immunoassay (CLIA), radioimmunoassay (RIA), colloidal gold immunoassay (CGIA), and fluorescence immunoassay (FIA).
2. Immune-based antibiotic screening assays
2.1. Preparation of antibodies
Immunoassay is based on the specific reaction of antigens and antibodies including polymers-proteins, polypeptides, polysaccharides, and nucleoproteins. Antigens are immunogenic and reactive and are initiated in the entire immunogen and antigen, which are made of macromolecular carrier and antigenic determinants epitopes. Low molecular substances can not induce the production of an antibody until it reacts specifically with the hapten. A polyclonal antibody is the total of antibodies in animal serum, which is the initial antibody used in research and application, and its preparation method is simple and economical. In general, the purified antigen-adjuvant is used to immunize the animals, obtain the immune serum, and to purify it. Adjuvants could be used to enhance the immunogenicity in order to expand the specificity of the antibody (Barinova, Khomyakova, Kuravsky, Schmalhausen, & Muronetz, Citation2017). However, due to its low specificity for multiple epitopes, it is mainly used for general antigen detection and has a slight value for the clinical diagnosis and treatment.
Methods for the preparation of monoclonal antibody have also many limitations such as the mAb can induce the human anti-mouse antibody, which restrict its application in the human body; it cannot effectively work a counterpart in the activation and Fc (immunoglobulin Fc) receptor-associated effect. The biological half-life of mAb is short and due to its large size it is difficult to penetrate through the tumour capillaries. Numerous other limitations are as follows: Low target specificity, difficult-to-obtain rare antibodies, large antigen usage, long immunization procedures, expensive, and difficult to produce in large quantities. By the progress of molecular biology and molecular immunology, a genetically engineered antibody is obtained by refining the preparation method. The genetically engineered antibody is the use of genetic engineering techniques to recombine the target antibody gene, clone it into an expression vector, and express and fold into a functional antibody molecule in a suitable host. The genetically engineered antibody has overcome the limitation of the traditional monoclonal antibody to a great extent and significantly expanded the scope of antibody application. At present, mainly the genetically engineered antibodies are a chimeric antibody, a humanized antibody, a Fab antibody (Crivianu-Gaita & Thompson, Citation2016), a variable fragment antibody, and a single-chain variable fragment antibody (Mala, Puthong et al., Citation2017). In addition, the short-chain antibodies are also commonly used.
2.2. Enzyme-linked immunosorbent assay
ELISA is a broadly documented method for the screening of antibiotic residues. Regarding their high-throughput sample, these assays significantly increase numeral studies necessary to characterize the food samples for drug contamination (Cao, He, et al., Citation2013 Franek, Kolar, Deng, & Crooks, Citation1999; Renson, Degand, Maghuin-Rogister, & Delahaut, Citation1993; Spinks, Schut, Wyatt, Spinks, & Morgan, Citation2001). Detection sensitivity depends on the strength of the signals during the reaction. In some of the reactions, the signal is produced by the enzyme which is connected to the detection element in a known amount to permit the precise quantification. The mainly used assay for the fast screening of a veterinary drug residue is the enzyme immune assay in which the glucose oxidase, horseradish peroxidase (HRP), pyruvate dehydrogenase, alkaline phosphatase (ALP), and recombinant β-galactosidase are used as labelling enzymes. The colour reaction occurs once the enzyme catalyses the substrate, while the quantitative or qualitative analysis is carried out according to the colour depth of the enzyme. The substrates for the glucose oxidase are an agreement of the basic communication services, HRP, and glucose, while the tetramethylbenzidine (TMB) was generally used as a substrate for HRP. The substrate for ALP is p-nitrophenyl phosphate, while the substrate for β-galactosidase is 4-nitrophenyl-N-acetyl-beta-d-galactosamine. Therefore, during the last decades, various ELISA methods were developed for antibiotic residue detection, such as competitive ELISA ((A)) and sandwich ELISA, as shown in .
Figure 1. Principles of the immunoassays for the detection of antibiotics. (A) ELISA; (B) CLIA; (C) RIA; (D) FIA; (E) CGIA.
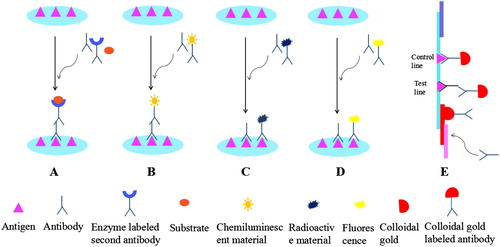
Table 1. ELISA for the screening of antibiotics in foods.
Many ELISA methods were applied for the detection of numerous antibiotic residues such as for fluoroquinolones (FQs) and chloramphenicol (CAP). The competitive ELISA methods for detecting the FQs were mainly based on drug-bovine serum albumin (BSA) conjugate and specific polyclonal antibody (Fan, Yang et al., Citation2012; Huang, Citation2014). While for the CAP and tetracycline (TC), mainly the principle of specific monoclonal antibody was followed (Gao, Zhao, Zhang, Wang, & Wang, Citation2013; Le, Yu, Zhao, & Wei, Citation2012; Liu et al., Citation2014). The ELISA method has also been used for the multi-analysis of antibiotic residues in different food matrices. Wang, Xu, Zhang, and He (Citation2009) and Jiang et al. (Citation2013) developed a novel colorimetric and dual-colorimetric ELISA for the simultaneous detection of different groups of antibiotic residues. This assay was applied to detect 13 FQ and 22 sulphonamide (SA) residues in different food matrices (Jiang et al., Citation2013; Wang et al., Citation2009). Another indirect competitive ELISA had been developed for the screening of sulphonamide residues which showed that the developed ELISA will be successfully applied for the detection of class-specific sulphonamides in feed and food sample such as muscle, milk, egg, and honey. This assay was based on indirect competitive ELISA and the immunoreagents were correctly shaped to screen a large number of sulphonamide residue congeners, finding the IC50 values beneath 10 mg L−1 for 11 sulphonamides (Galarini, Diana et al., Citation2014). The sensitivity of the ELISA method has been increased by the addition of a new system of biotin–streptavidin–amplified ELISA (BA-ELISA). This system is based on the strong affinity and higher specificity between streptavidin and biotin to facilitate more molecules of the enzyme to catalyse the substrate. Jeon and Paeng (Citation2008), Wang, Zhang, Gao, Duan, and Wang (Citation2010), Jiang et al. (Citation2015) established a sensitive BA-ELISA method for the determination of TC, chloramphenicol, and lincosamide (Jeon & Paeng, Citation2008; Jiang et al., Citation2015; Wu, Zhang, & Zhou, Citation2010). Another ELISA method has been developed for the detection of penicillin in the milk sample. This assay is based on open-ringed forms of penicillin which were treated by hybrid magnetic particles which permit the detection of two types of penicillin simultaneously (Broto, Matas, Babington, Marco, & Galve, Citation2015). Additionally, a specific and sensitive ELISA has been established to detect the 1-amino-hydantoin (AHD) residues in fish, shrimp, pork, and chicken samples, a specific antibody was prepared based on the monoclonal antibody knowledge against AHD. The developed method was rapid, simple, and precise to detect the residue of AHD in edible animal tissues (Jiang et al., Citation2012). Regarding the residual consequences of banned antibacterial drugs, another ELISA has been developed for the detection in animal feed samples. This assay was based on the qualitative analysis of bacitracin and virginiamycin and has been suggested as a suitable screening method for the routine use. Some of the other ELISA methods have also been established for the detection of several illegal antibiotic usage in aquaculture and feed samples (Conti et al., Citation2015; Squadrone et al., Citation2015).
In addition to antigen and antibody, antibiotics can also be detected by the competition between receptors and drugs. Currently, competitive receptor-based ELISA has been established for detecting β-lactam antibiotics from numerous food samples. This assay is grounded on the principle of direct competitive protein activity by HRP-AMP (ampicillin) and free β-lactam (Peng et al., Citation2013). Furthermore, the chemiluminescent technique, combined with ELISA, was used to detect antibiotic residues. Tao et al. (Citation2012) developed indirect chemiluminescent enzyme-linked immunoassay (CL-ELISA) for screening CAP in milk and chicken muscle (Tao et al., Citation2012). Previously, immunoassays, especially the ELISA, have proved to be an efficient method for the analysis of numerous samples in a timely manner. ELISA is a simple, rapid, sensitive, and high-throughput assay (Jiang et al., Citation2015). It is the most widely used and the time-saving developing method of enzyme immunology. Nevertheless, it has also some disadvantages such as poor reproducibility, prone to false positives by the interference of auto-antibodies, and some of the other interference factors can also become the limitation for the use of these assays. Additionally, PcAb is supposed to be an unsuitable choice for the development of the immunoassay method for the detection of antibiotic residues (Liu et al., Citation2014).
2.3. Chemiluminescent immunoassay
CLIA is applicable in different extents such as clinical diagnoses, pharmaceutical analysis, food safety, and environmental monitoring. It has been widely used because of its simple analysis, rapid, sensitive, and selective approach (Chen, Jie, Chen, Jie, & Huang-Xian, Citation2012). CLIA consists of two parts; the immune response system and chemiluminescence analysis system. The immune reaction system is to directly label luminescent substances or enzymes on antigens or antibodies to form a complex when the antigen reacts with an antibody ((B)). The chemiluminescence analysis system is the substrate adding oxidant or enzyme in the immune response. After the chemiluminescent (CL) substance by oxidation, the formation of an exciting intermediate will take place. When the ground state excited and back to a stable intermediate, and emit photons (hM), using the light-emitting signal measuring instrument for detecting the signal. This method is used to investigate the concentration of analytic agents regarding the strength of the luminescence which is produced by chemical reaction.
CLIA is mainly divided into CLIA and chemiluminescent enzyme immunoassay (CLEIA) based on different labelled substances. CLIA uses CL to label the antibody and by introducing the CL substrates, the system produces CL; therefore, the samples can be screened quantitatively. Acridinium ester derivative was used as a substrate for CL, while CLEIA used peroxidase to label an antibody and luminol as a substrate … ALP and HRP are the most generally used labelling enzymes. The substrate was counted as the main difference between the HRP used in ELISA and CLEIA. The TMB was used as a substrate in ELISA to display the colour while it is luminol in CLEIA which is used for the emission of light. Nanotechnology has been extensively used for the bio-labelling, for example AuNPs are used to label an antibody to improve the luminal-H2O2 system CL (Chen et al., Citation2012).
Previously, an Indirect competitive chemiluminescence enzyme immunoassay (IC-CLEIA) was developed for the detection of CAP residues in shrimp. The limit of detection observed by the assay was 0.01 ng/mL (Chuanlai, Cifang, Kai, Zhengyu, & Wukang, Citation2006), while a competitive CLIA was established for the determination of macrolide in plasma and phosphate-buffered saline. A precise antibody against sirolimus was produced by its well-synthesized immunogen and a competitive CLIA. The macrolide compound was modified with carboxymethoxylamine and succinic anhydride. The observed limit of detection between two samples was 0.2 and 0.25 ng mL−1 (Zhang et al., Citation2016). A novel approach has been established by Tao et al. (Citation2014) for the simultaneous determination of CAP and clenbuterol in milk. This assay is mainly based on the single immunoassay for the determination of both CAP and clenbuterol (Tao et al., Citation2014). Wutz, Niessner, and Seidel (Citation2011) developed another innovative approach of CL multi-analyte chip immunoassay for the screening of four antibiotic residues. This assay is based on the antigen–antibody interaction to be detected by CL (Wu et al., Citation2010). Due to the high specificity and sensitivity, CLIA is broadly applicable in various fields, such as chemical diagnoses, environmental monitoring, and pharmaceutical analysis (Chen et al., Citation2012). However, there are some of the limitations due to the compounds used in this assay, such as acridinium derivatives which have a high-quantum yield even after easy coupling to proteins, they do not need catalysts, background signals are low, and high sensitivities are frequently obtained. The immediate light emission has also been considered to be a disadvantage because of its measuring problems, which permit high rates in automated analysers (Dodeigne, Thunus, & Lejeune, Citation2000) ().
Table 2. Chemiluminescence immunoassay for the screening of antibiotics in foods.
2.4. Radioimmunoassay
RIA uses isotope-labelled and unlabelled antigens to react competitively with antibodies ((C)). It has miscellaneous applications and included both the qualitative and quantitative tools for the detection of antibiotics and hormones in the samples from the different origin such as in veterinary, human, and agriculture field, as shown in (Agarwal, Citation1992). Numerous RIAs have also been established for clinical and medical examinations. Fewer studies have been conducted on the employment of RIAs for the quantitative detection of antibiotic residues in the aquatic environment. (Fan et al., Citation2012) modified the Charm II RIA test, a commercially available RIA method that was developed for the screening of TCs in serum, urine, milk, and tissue, and the screening of TCs in surface water, groundwater, and hog lagoon wastewater. Yang and Carlson (Citation2004) introduced the RIA/solid-phase extraction method for the semiquantitative determination of SA and TC in drinking water (Yang & Carlson, Citation2004).
Table 3. RIA and CGIA/LFIA for the screening of antibiotics in foods.
A procedure from a commercially available RIA for testing the TC class of antibiotics in a biological medium was modified to screen in water with a LOD of 1 ppb. The data also suggest that a more sensitive method needs to be developed for analysing the water regarding the antibiotic compounds away from the sources (Meyer et al., Citation2000). Al-Mazeedi et al. (Citation2010) announced the survey for determining the TC residue in dairy products by Charm II radioimmunoassay (Al-Mazeedi et al., Citation2010). RIA has been used as a qualitative or semi-quantitative tool and a screening method to reduce the sample load for direct analyses, this assay is thought to be ideal for several samples for the presence of analytic agents (Yang & Carlson, Citation2004). The advantages of RIA are sensitive, specific, simple, and user-friendly. RIA also has some of the drawbacks by using the radioisotopes and scintillation fluids (Wang, Beier, & Shen, Citation2017).
2.5. Fluorescence immunoassay/fluorescence polarization immunoassay
FIA method links the fluorophore to a specific antigen by an immunological method and labels the fluorescent group as a standard reagent to detect and identify the unknown antigen. FPIA is a homogenous assay that has no washing or separation steps. This assay is based on the rise in the polarization of the fluorescence of a small fluorescent-labelled antigen (tracer) by binding with a specific antibody ((D)). The presence of antigen in the sample competes with the tracer for binding with an antibody to decrease the polarization signal. FPIA is a competitive method based on the detection of the fluorescence polarization (FP) of reaction mixtures containing the sample analyte, fluorescent-labelled tracer, and a specific antibody. If the analyte is not present in the sample, the tracer will be bound to the antibody and the FP will be high. When the analyte concentration in the sample is significantly higher than the concentration of the tracer, the antibody-binding site will be preferentially occupied by the analyte and most of the tracer will be free in the solution. The FP of the reaction mixture will then be lower (Smith & Eremin, Citation2008). Many of the FPIAs have been established for antibiotic residue detection, as shown in .
Table 4. FPIA for the screening of antibiotics in foods.
A rapid one-step FPIA has been established for the simultaneous determination of several fluoroquinolones in food samples. This assay is based on the production of a monoclonal antibody (Mi, Wang, Eremin, Shen, & Zhang, Citation2013). Another assay has been established a homogenous FPIA based on the monoclonal antibody and the tracer for the simultaneous determination of cefalexin and cefadroxil in milk samples (Zhang, Wang, Mi, Wenren, & Wen, Citation2014). A heterologous structure of FPIA was established for the determination of clinafloxacin in goat milk which has been observed to be more sensitive than homologous structure (Chen, Shanin, et al., Citation2016). Another sensitive quantitative FPIA immunochemical approach has been reported for the screening of gentamicin in milk samples. The observed limit of detection was 5 μg kg−1 (Beloglazova, Shmelin, & Eremin, Citation2016). Shanin, Shaimardanov, Thai, and Eremin (Citation2015) developed one reagent FPIA for the screening of levofloxacin in urine samples (Shanin et al., Citation2015). Another novel approach for multiplexed FPIA has been established for the determination of SA and FQ which is based on bi-specific single-chain diabody (Chen et al., Citation2014). FIA is considered as a reliable and robust screening method which detects different antibiotics simultaneously in a short period. Different from CL substances, fluorescent dyes can label proteins, while many proteins and dyes can be used for multiple labelling. They are characterized by large signal intensity, fast imaging speed, and low experimental cost. However, this assay has some limitation that it needs to carry out a sample preparation step in order to extract the analyte from the bovine muscle and make the sample colourless (filtration step) because the colouration can interfere in the final fluorescence emission of quantum dots (QDs) (García-Fernández, Trapiella-Alfonso et al. Citation2014).
2.6. Colloidal gold immuno-chromatographic assay (CGIA)
CGIA with colloidal gold as a tracer in the alkaline environment with negative and positive charge groups and antibody protein molecules create a new immune detection method. Various assays have been developed for the detection of antibiotic residues, as shown in (Posthuma-Trumpie, Korf, & van Amerongen, Citation2009). A CGIA has been established for the rapid determination of CAP residues in aquatic products. CAP polyclonal antibody was used as a marker protein, while a nitrocellulose membrane was used as the carrier, the assay was based on the immobilization of CAP antibody on the conjugate pad ((E)) (Zhou, Zhang, et al., Citation2014). An assays had been established with a high-throughput, sensitive, and rapid CGIA for the simultaneous determination of tetracycline, sulphonamide, and quinolone residues in milk. In this study CG, Latex bead LB-based immunochromatographic assay has been developed for the detection of three different class of antibiotic residues. This assay simultaneously detects 36 different structurally antibiotics within 10 min (Wang, Li, et al., Citation2017). However, another CGIA had also been developed for the screening of streptomycin residue in milk and swine urine. This assay based on a protein conjugate of streptomycin conjugated with BSA was produced and was applied as an immunogen to yield monoclonal antibodies. The examined limit of detection was 2.0 and 1.9 ng/mL for milk and urine, respectively (Wang et al., Citation2010). Another novel method had been previously established based on the cysteamine-stabilized gold particle for the detection of tetracycline in the milk sample, this assay was a simple, time-saving with a better selectivity (Luo et al., Citation2015).
2.7. Lateral flow immunoassay
Recently, lateral flow immunoassay (LFIA) has been used for the semi-quantitative, quantitative, and environmental monitoring. A classical LFIA is a strip of carrier substance that contains a dry reagent which was activated once put on to the fluid samples. This assay is important for the diagnostic purposes such as internal organ failure, pregnancy, contamination or infection with a specific pathogen, presence of toxic compound in the environment, or food and drug abuse. Jo et al. (Citation2015) introduced a kit prepared on LFIA for the simultaneous detection of quinolones, beta-lactams, sulphonamides, and TC residues in fish samples which is based on the interaction of antigen and antibody (Jo et al., Citation2015). A QD-labelled LFIA has been developed for the determination of CAP in milk (Berlina, Taranova, Zherdev, Vengerov, & Dzantiev, Citation2013).
LFIA was practical in a wide range of screening and diagnosis purposes. This assay has many advantages such as easily scalable to high-volume production, stable-shelf-lives of 12–24 months often without refrigeration, simple to use, no specific expert is be required: minimum operator-dependent steps and interpretation can handle small volumes of multiple sample types that can be integrated with on-board electronics, reader systems, highly sensitive information system, stable, specific, economical, and time-saving. However, LFIA also have also some of the limitations such as ambiguous results, miniaturization and in the simultaneous investigation of multiple markers are difficult to integrate on-board electronics and to built-in QC functions challenge, the sensitivity issues in some of the systems test-to-test reproducibility challenging (O’Farrell, Citation2009). Each assay has some advantages and disadvantages, as shown in .
Table 5 Advantages and disadvantages of immunoassay.
2.8. Other immunoassay
In recent years, some novel immunoassays have been introduced to detect antibiotic residues such as immune chip technology, surface plasmon resonance immune technology, immunosensors, and others. Biochip assay contains an assembly of microarrays organized on a solid substrate that permits several tests to be done at the same time. Biochip assay is based on specific identification and analyte-binding target present in a sample bio-receptor or molecule that was set in an ordered manner, which permits the analyte detection quantitatively or semi-quantitatively. The Bio-receptors include antibodies, nucleic acids, phage display peptides, aptamers, enzymes and cellular components, and synthetic peptides, as well as metal oxides. The probes are planned to offer sensitivity, specificity, and detection of the targeted analyte among the recommended standards settled by regulatory authorities (Thakur et al., Citation2014). Biochip array assay can be practical in a repetitive examination because of sample high-throughput, in addition to the observation databases to control the existence of antibiotic residues. This assay shortens the confirmatory study by offering separable sulphonamide confirmation and semi-quantitative results. This assay also allows a simultaneous wide numeral screening of sulphonamides in a single sample. Miniaturization of this method decreases the volume of the reagent and sample for each test and increases the output. Ionela et al. developed the biochip assay that showed a good specificity, sensitivity, linearity, precision, recovery and LOD beneath 50% of maximum residue limits (MRLs). The LODs ranged from 0.3 to 7.5 µg/kg for sulphonamides). Mohony and his coworker established a biochip array method that can screen all four metabolites below the reference point of 1 g kg−1. The detection ability was beneath 0.5 g kg−1 for the AHD, AOZ, and AMOZ metabolites; it was below 0.9 g kg−1 for SEM. The observed IC50 values ranged from 0.14 to 2.19 g kg−1 (O’Mahony et al., Citation2011). Another biochip-based screening immunoassay was developed for simultaneous screening and quantitation of dissimilar group of six antibiotics in urine, milk, honey, meat, and feed. Generally, this assay was practical in clean oral fluid samples. The sample cleanliness must be measured throughout the establishment of this technology specifically for TCs (Oruc, Rumbeiha, Ensley, Olsen, & Schrunk, Citation2013). AKSEM AKSOY performed the experiment regarding screen and evaluated the presence of possible 12 different antibiotic residue simultaneously in honey based on biochip multi-array technology. Regarding to the detection analyses, the results showed that streptomycin, erythromycin, tylosin B, neomycin, lincomycin, and amikacin residues were observed in honey samples in numerous concentrations (Aksoy, Citation2019). Another study has been conducted by Mi-Sun Ha and for the detection of enrofloxacin by using biochips and microparticles. Enrofloxacin in solution strives with the microparticle-immobilized enrofloxacin (enroMPs) to bind to the antibody on the chip. The enrofloxacin existence has been proved by sensing the fluorescence of enrofloxacin-bound microparticles. The observed limits of detection and limit of quantification for standard solutions ranged from 5 to 20 μg kg−1. Due to the specificity, sensitivity, rapidity, and simplicity, this assay will ease the screening and estimated quantification of enrofloxacin residues in animal-derived foods in a high-throughput way. Regarding the limitations of the current assays which targets most of the necessary groups for the immobilization, the modifications of these groups do not significantly affect the binding affinity toward the corresponding antigen or antibody (Ha, Chung, & Bae, Citation2016). Popa et al. developed a chemiluminescence-based biochip array sensing technique, it can simultaneously detect quinolones, ceftiofur, thiamphenicol, streptomycin, tylosin, and tetracyclines in honey samples (Popa, Schiriac et al. Citation2012). The limits of detection (LODs) were in the range of 1 μg/kg for thiamphenicol and 9 μg/kg for tetracycline.
Xia et al. developed a surface plasmon resonance-based biosensor for the sequential detection of chloramphenicol and gentamicin in milk. The LOD was 5.28 μg/kg for chloramphenicol and 2.26 μg/kg for gentamicin (Xia et al., Citation2017). Pennacchio et al. coupled penicillin G with the carrier protein and then immunized the rabbits to produce polyclonal antibodies, developed surface plasmon resonance method for the detection of penicillin G in which the LOD is lower than the MRL of EU (Pennacchio et al., Citation2015). Losoyaleal et al. developed a surface plasmon resonance-based immunoassay for the detection of amikacin in milk; their LOD was 0.13 μg/kg (Losoya-Leal, Estevez, Martínez-Chapa, & Lechuga, Citation2015).
Conzuelo et al. developed a novel integrated amperometric immune-sensor, based on the immobilization for the detection of sulphonamide and tetracycline antibiotic residues in milk samples with very low LOD (in the low ppb level) (Conzuelo et al., Citation2013). Yang et al. developed a novel sensor based on competitive surface-enhanced Raman scattering immunoassay and magnetic separation for residual CAP detection in the real aquatic environment (Yang, Hu, & Dong, Citation2016). Jornet et al. developed an immune-sensor for the determination of sulfathiazole in water and honey, the LOD was 0.3 μg/kg and only need 2 min (Jornet, González-Martínez, Puchades, & Maquieira, Citation2010).
Additionally, numerous other immunoassays had also been displayed, such as Lingying Zhou and his co-worker developed a label-free, high-throughput, and automatic aptasensor for antibiotic detection that was grounded on a universal microchip electrophoresis (MCE) CAP aptamer was used as a probe deprived of labelling any signal cause substance which can significantly shorten the preparation procedure for aptasensor. The detection time was observed within 3 min. The examined limit of detection for CAP was 0.003 ng/mL. This assay showed a higher sensitivity for CAP than other antibiotics including thiamphenicol (TAP), kanamycin (KANA), and oxytetracycline (OTC). The offered assay can be prolonged for the detection of other targets by exchanging the countering aptamer and CDNA probe (Zhou et al., Citation2017). Another universal MCE array-based aptasensor assay has been developed for multiplex antibiotic residue detection by Lingying zhou. Novel multiple aptamer-functionalized magnetic beads were planned for the development of this assay and PCR was used for signal amplification. The examined detection time was about 1 min for one antibiotic in one sample. Additionally, this assay evidenced to be a competent platform for multiplex antibiotic residue detection for the safety of food. This method can work on other antibiotic residue detection by exchanging the particular aptamer (Zhou et al., Citation2018). Chen and co-worker developed a multiplexed aptasensor based on the universal double-T-type microchip to one step for the simultaneous screening of numerous antibiotics in a very short period including kanamycin and chloramphenicol as a representative. The assay was effectively applied in fish, milk, and samples, the examined limit of detection limits was 0.52 CAP and 0.41 pg mL−1 Kana. Furthermore, the method offers a countless prospective application for the screening of antibiotic residues in food samples (Zhang et al., Citation2019). Another novel label-free and multiplex aptasensor for the simultaneous screening of numerous antibiotic residues has been developed that is grounded on a microchip electrophoresis platform and targets catalysed hairpin assembly for the amplification of the signal. Oxytetracycline and Kanamycin were applied as models. The assay displayed a linear correlation ranging from 0.001 to 10 ngmL−1, with the limit of detection 0.7 and 0.9 pgmL−1 to Kana and OTC, respectively. This assay has some of the advantages such as, the aptamer probes can be invented simply deprived of labelling signal devices for MCE screening; Secondly, the targets can just respond with probes and yield the amplified signal in one-pot. Regarding this examination, it was expected that this assay will be universally functioned as a sensitive, simple, and movable platform for the detection of antibiotic residue in environmental and biological samples (Wang, Gan, et al., Citation2017). Additionally, a ratiometric and homogeneous assay has been developed for kanamycin detection that was based on MCE the LOD was observed as 150 fg mL−1. This assay was effectively applied for the detection of fish and milk samples with reliable outcomes of the enzyme-linked immune sorbent assay. The amount of target can be converted quantitatively to the intensity of DNA deprived of labelling with MCE and attained exonuclease-assisted signal in the solution by the R-DNA probe. This assay offers an important platform for the rapid detection of antibiotic residues in food matrices (Chen et al., Citation2018). Another flow-through chemiluminescence microarray was conducted which can simultaneously detect 13 antibiotics in milk (Meyer et al., Citation2017). The bioluminescence resonance energy transfer homogeneous immunoassay was based on QDs that can detect 7 FQs in milk (Yu et al., Citation2016). The fluorescent microspheres of immune-chromatographic assay can detect lincomycin and macrolides (Li, Shen, et al., Citation2015 Zhou, Zhu, et al., Citation2014). Another novel colorimetric aptasensor was also developed for the sensitive detection of TC in honey (Wang, Liu, et al., Citation2015). Sánchez-Martínezet al. developed a simple and rapid long-wavelength homogeneous enzyme immunoassay for the determination of amikacin in water samples, the LOD was 0.3 μg/kg (Sánchez-Martínez, Aguilar-Caballos, & Gómez-Hens, Citation2009). Another new efficient sensing instrumental for the detection has been observed based on aptasensor. In this assay, the aptamers are widely used as a recognition substance. Aptamers are synthetically produced and they contain single-stranded oligonucleotides that have a strong affinity toward their target. Previously, two main aptasensors have been developed for the detection of chloramphenicol, which consists of electrochemical and optical groups. The examined limit of detection by electrochemical-based aptasensor assay is lower than the optical-based aptasensor assay. Despite its exclusive advantages, it consumes more time for the preparation of fabrication (Khoshbin, Verdian, Housaindokht, Izadyar, & Rouhbakhsh, Citation2018).
In addition, some improvement like improving the affinity of the receptor protein has also been done by conducting a study with a new aspect. A study has been conducted by Saeed and his co-worker to develop an enzyme-linked receptor assay based on the BlaR-CTD mutant protein for the detection of 40 beta-lactam antibiotics in different animal-derived foods. In the study they designed different mutants to enhance the affinity of receptor protein for binding with numerous beta-lactam antibiotics. The selected mutant shows higher affinity to 33 beta-lactams antibiotic than wild type BlaR-CTD protein, and the ELRA method developed by it observed an admirable limit of detection lower than MRL set by the authorities (Ahmed et al., Citation2020; Ning et al., Citation2019). Another promising technique for the improvement of the affinity of detection assay was observed as aptamers sensing method. The study was based on high sensitivity, rapidity, selectivity to specific antibiotics. A multi-plexed aptasensor by the FRET strategy using DNase I-assisted cyclic enzymatic resonance signal amplification together with aptamer oxide complex has been used. Regarding this assay, the observed limit of detection for kanamycin, sulfadimethoxine, and ampicillin was 2.66, 1.99, and 2.33, respectively. The apatasensor assay offers high specificity and permits the simultaneous detection and multi-colour sensing for the detection of multiple antibiotic residues at the same time (Youn et al., Citation2019).
3. Perspective
The mainly documented screening methods are microbiological and immunological assays, while the most considered immunoassays at present are ELISA, FPIA, RIA, and CGIA/LFIA. The immunoassays are characterized by their high specificity and sensitivity. In general, the LODs achieved are lower and the analysis time is significantly shorter than that required for some of the microbiological tests. Based on the manipulation of assay formats and the use of broad-specificity antibodies, the multi-analysis of several targets in one single test is available. It is believed that more and more techniques will be used in immunoassay with the advancement of science.
Furthermore, the designing of antibodies still plays a very important role in the efficiency enhancement of the screening assay. The design that is used to identify more drug sites with antigens or hapten is more helpful for drug identification (Zeng et al., Citation2016). Designing of hapten is important to highlight the common parent structure of the drug so that the antibodies can identify as many structurally similar drugs as they can. The sensitivity of the detection method can also be improved by labelling methods. For example, in enzyme immunoassay, the enzyme acts on the substrate to raise the colour or luminescence. Moreover, the substrate can be enlarged to increase the detection sensitivity. So, the substrate amplification system can be used in the immunoassay where the sensitivity can be improved by improving the quality of the signal. Additionally MCE and HPLC methods can be reflected as a new outlook to establish the able aptasensors because of their short detection time. The MCE method is a competent approach for the antibiotic detection at picomole level. Furthermore, finding of the novel aptamers for the drugs with an improved affinity should be a significant future to establish effective aptasensors. However, it is anticipated that further effort should be made to develop the research and overcome the limitations regarding the applicability, validation from regulatory agencies, and upgrading these assays in the routine monitoring of antibiotic residues in the environment and food samples. Low cost, stable antigen, antibodies, and likewise simple synthesis assays should be established to save the time, easy tracer preparation, and screening of antibiotic residues. More efforts with immense attention should be exerted on the assay automation, selection of specific proteins, high-quality antibody production, screening of a multi-drug, designing, and construction of metal–organic coordination polymers to enhance the application of these assays for real sample in future.
In practical applications, the combination, abuse, and ignorance of applications can be the source of many types of antibiotic residues in animal-derived food, so the detection of antibiotic residues can be in the direction of future research of multi-residue detection. If the residues of antibiotics are detected simultaneously the efficiency can be upgraded and the cost of detection will be significantly reduced. For different kinds of antibiotics, a multi-residue detection study will have to design a broad specificity antibody by using a chip technology to design a multiple immune chip detection method and simultaneous labelling of multiple antibodies by multi-probe labelling. Moreover, with the progress of knowledge, new technologies and methods can be used for the detection of multiple antibiotic residues.
Ethical approval
This article does not contain any studies with human participants or animals performed by any of the authors.
Disclosure statement
No potential conflict of interest was reported by the author(s).
Additional information
Funding
References
- Agarwal, V. K. (1992). Analysis of antibiotic/drug residues in food products of animal origin. New York, London: Springer.
- Ahmed, S., Ning, J., Cheng, G., Ahmad, I., Li, J., Mingyue, L., … Yuan, Z. (2017). Receptor-based screening assays for the detection of antibiotics residues – a review. Talanta, 166, 176–186.
- Ahmed, S., Ning, J., Cheng, G., Maan, M. K., Chen, T., Ahmad, I., … Yuan, Z. (2020). Development and validation of an enzyme-linked receptor assay based on mutant protein I188 K/S19C/G24C for 40 beta-lactams antibiotics detection in 13 food samples. Microchemical Journal, 152, 104354.
- Aksoy, A. (2019). Simultaneous screening of antibiotic residues in honey by biochip multi-array technology. Medycyna Weterynaryjna-Veterinary Medicine-Science And Practice, 75(9), 567–571.
- Al-Mazeedi, H. M., Abbas, A. B., Alomirah, H. F., Al-Jouhar, W. Y., Al-Mufty, S. A., Ezzelregal, M. M., & Al-Owaish, R. A. (2010). Screening for tetracycline residues in food products of animal origin in the State of Kuwait using Charm II radio-immunoassay and LC/MS/MS methods. Food Additives and Contaminants: Part A, 27(3), 291–301.
- Barinova, K., Khomyakova, E., Kuravsky, M., Schmalhausen, E., & Muronetz, V. (2017). Denaturing action of adjuvant affects specificity of polyclonal antibodies. Biochemical and Biophysical Research Communications, 482(4), 1265–1270.
- Beloglazova, N., Shmelin, P., & Eremin, S. (2016). Sensitive immunochemical approaches for quantitative (FPIA) and qualitative (lateral flow tests) determination of gentamicin in milk. Talanta, 149, 217–224.
- Benito-Peña, E., Moreno-Bondi, M. C., Orellana, G., Maquieira, Á, & van Amerongen, A. (2005). Development of a novel and automated fluorescent immunoassay for the analysis of β-lactam antibiotics. Journal of Agricultural and Food Chemistry, 53(17), 6635–6642.
- Berlina, A. N., Taranova, N. A., Zherdev, A. V., Vengerov, Y. Y., & Dzantiev, B. B. (2013). Quantum dot-based lateral flow immunoassay for detection of chloramphenicol in milk. Analytical and Bioanalytical Chemistry, 405(14), 4997–5000.
- Broto, M., Matas, S., Babington, R., Marco, M.-P., & Galve, R. (2015). Immunochemical detection of penicillins by using biohybrid magnetic particles. Food Control, 51, 381–389.
- Byzova, N. A., Smirnova, N. I., Zherdev, A. V., Eremin, S. A., Shanin, I. A., Lei, H.-T., … Dzantiev, B. B. (2014). Rapid immunochromatographic assay for ofloxacin in animal original foodstuffs using native antisera labeled by colloidal gold. Talanta, 119, 125–132.
- Cao, B., He, G., Yang, H., Chang, H., Li, S., & Deng, A. (2013). Development of a highly sensitive and specific enzyme-linked immunosorbent assay (ELISA) for the detection of phenylethanolamine A in tissue and feed samples and confirmed by liquid chromatography tandem mass spectrometry (LC–MS/MS). Talanta, 115, 624–630.
- Cao, B., Yang, H., Song, J., Chang, H., Li, S., & Deng, A. (2013). Sensitivity and specificity enhanced enzyme-linked immunosorbent assay by rational hapten modification and heterogeneous antibody/coating antigen combinations for the detection of melamine in milk, milk powder and feed samples. Talanta, 116, 173–180.
- Cháfer-Pericás, C., Maquieira, Á, Puchades, R., Miralles, J., & Moreno, A. (2011). Multiresidue determination of antibiotics in feed and fish samples for food safety evaluation. Comparison of immunoassay vs LC-MS-MS. Food Control, 22(6), 993–999.
- Chen, J., Shanin, I. A., Lv, S., Wang, Q., Mao, C., Xu, Z., … Lei, H. (2016). Heterologous strategy enhancing the sensitivity of the fluorescence polarization immunoassay of clinafloxacin in goat milk. Journal of the Science of Food and Agriculture, 96(4), 1341–1346.
- Chen, M., Wen, K., Tao, X., Ding, S., Xie, J., Yu, X., … Xie, S. (2014). A novel multiplexed fluorescence polarisation immunoassay based on a recombinant bi-specific single-chain diabody for simultaneous detection of fluoroquinolones and sulfonamides in milk. Food Additives & Contaminants: Part A, 31(12), 1959–1967.
- Chen, W., Jie, W., Chen, Z., Jie, X., & Huang-Xian, J. (2012). Chemiluminescent immunoassay and its applications. Chinese Journal of Analytical Chemistry, 40(1), 3–10.
- Chen, X., Hong, F., Cao, Y., Hu, F., Wu, Y., Wu, D., … Gan, N. (2018). A microchip electrophoresis-based assay for ratiometric detection of kanamycin by R-shape probe and exonuclease-assisted signal amplification. Talanta, 189, 494–501.
- Chen, Y., Chen, Q., Han, M., Liu, J., Zhao, P., He, L., … Zhang, L. (2016). Near-infrared fluorescence-based multiplex lateral flow immunoassay for the simultaneous detection of four antibiotic residue families in milk. Biosensors and Bioelectronics, 79, 430–434.
- Chen, Y., Wang, Y., Liu, L., Wu, X., Xu, L., Kuang, H., … Xu, C. (2015). A gold immunochromatographic assay for the rapid and simultaneous detection of fifteen β-lactams. Nanoscale, 7(39), 16381–16388.
- Chuanlai, X., Cifang, P., Kai, H., Zhengyu, J., & Wukang, W. (2006). Chemiluminescence enzyme immunoassay (CLEIA) for the determination of chloramphenicol residues in aquatic tissues. Luminescence, 21(2), 126–128.
- Conti, G. O., Copat, C., Wang, Z., D'Agati, P., Cristaldi, A., & Ferrante, M. (2015). Determination of illegal antimicrobials in aquaculture feed and fish: An ELISA study. Food Control, 50, 937–941.
- Conzuelo, F., Campuzano, S., Gamella, M., Pinacho, D. G., Reviejo, A. J., Marco, M. P., & Pingarrón, J. M. (2013). Integrated disposable electrochemical immunosensors for the simultaneous determination of sulfonamide and tetracycline antibiotics residues in milk. Biosensors and Bioelectronics, 50, 100–105.
- Crivianu-Gaita, V., & Thompson, M. (2016). Aptamers, antibody scFv, and antibody Fab'fragments: An overview and comparison of three of the most versatile biosensor biorecognition elements. Biosensors and Bioelectronics, 85, 32–45.
- Cullor, J. (1993). Antibiotic residue tests for mammary gland secretions. Veterinary Clinics of North America: Food Animal Practice, 9(3), 609–620.
- Cullor, J., Van Eenennaam, A., Dellinger, J., Perani, L., Smith, W., & Jensen, L. (1992). Antibiotic residue assays: Can they be used to test milk from individual cows? Veterinary Medicine (USA), 87(5), 477–494.
- Dodeigne, C., Thunus, L., & Lejeune, R. (2000). Chemiluminescence as diagnostic tool. A review. Talanta, 51(3), 415–439.
- Du, X., Zhang, F., Zhang, H., Wen, Y., & Saren, T. (2014). Substitution of antibody with molecularly imprinted 96-well plate in chemiluminescence enzyme immunoassay for the determination of chloramphenicol residues. Food and Agricultural Immunology, 25(3), 411–422.
- Fan, G.-y., Yang, R.-s., Jiang, J.-q., Chang, X.-y., Chen, J.-j., Qi, Y.-h., … Yang, X.-f. (2012). Development of a class-specific polyclonal antibody-based indirect competitive ELISA for detecting fluoroquinolone residues in milk. Journal of Zhejiang University Science B, 13(7), 545–554.
- Franek, M., Kolar, V., Deng, A., & Crooks, S. (1999). Determination of sulphadimidine (sulfamethazine) residues in milk, plasma, urine and edible tissues by sensitive ELISA. Food and Agricultural Immunology, 11(4), 339–349.
- Galarini, R., Diana, F., Moretti, S., Puppini, B., Saluti, G., & Persic, L. (2014). Development and validation of a new qualitative eLISA screening for multiresidue detection of sulfonamides in food and feed. Food Control, 35(1), 300–310.
- Gao, F., Zhao, G. X., Zhang, H. C., Wang, P., & Wang, J. P. (2013). Production of monoclonal antibody against doxycycline for immunoassay of seven tetracyclines in bovine muscle and milk. Journal of Environmental Science and Health, Part B, 48(2), 92–100.
- García-Fernández, J., Trapiella-Alfonso, L., Costa-Fernández, J. M., Pereiro, R., & Sanz-Medel, A. (2014). A quantum dot-based immunoassay for screening of tetracyclines in bovine muscle. Journal of Agricultural and Food Chemistry, 62(7), 1733–1740.
- Ha, M.-S., Chung, M.-S., & Bae, D.-H. (2016). Simple detection of residual enrofloxacin in meat products using microparticles and biochips. Food Additives & Contaminants: Part A, 33(5), 817–823.
- Han, S., Zhou, T., Yin, B., & He, P. (2016). A sensitive and semi-quantitative method for determination of multi-drug residues in animal body fluids using multiplex dipstick immunoassay. Analytica Chimica Acta, 927, 64–71.
- He, X., Duan, C. F., Qi, Y. H., Dong, J., Wang, G. N., Zhao, G. X., … Liu, J. (2017). Virtual mutation and directional evolution of anti-amoxicillin scFv antibody for immunoassay of penicillins in milk. Analytical Biochemistry, 517, 9–17.
- He, J., Wu, N., Luo, P., Guo, P., Qu, J., Zhang, S., … Wang, C. (2017). Development of a heterologous enzyme-linked immunosorbent assay for the detection of clindamycin and lincomycin residues in edible animal tissues. Meat Science, 125, 137–142.
- Hu, G., Sheng, W., Zhang, Y., Wu, X., & Wang, S. (2015). A novel and sensitive fluorescence immunoassay for the detection of fluoroquinolones in animal-derived foods using upconversion nanoparticles as labels. Analytical and Bioanalytical Chemistry, 407(28), 8487–8496.
- Huang, Z. (2014). Development of an indirect competitive eLISA for detection of danofloxacin residue in milk. International Food Research Journal, 21(4), 1419–1424.
- Jeon, M., & Paeng, I. R. (2008). Quantitative detection of tetracycline residues in honey by a simple sensitive immunoassay. analytica Chimica Acta, 626(2), 180–185.
- Jiang, W., Beier, R. C., Luo, P., Zhai, P., Wu, N., Lin, G., … Xu, G. (2015). Analysis of pirlimycin residues in beef muscle, milk, and honey by a biotin–streptavidin-amplified enzyme-linked immunosorbent assay. Journal of Agricultural and Food Chemistry, 64(1), 364–370.
- Jiang, W., Luo, P., Wang, X., Chen, X., Zhao, Y., Shi, W., … Shen, J. (2012). Development of an enzyme-linked immunosorbent assay for the detection of nitrofurantoin metabolite, 1-amino-hydantoin, in animal tissues. Food Control, 23(1), 20–25.
- Jiang, W., Wang, Z., Beier, R. C., Jiang, H., Wu, Y., & Shen, J. (2013). Simultaneous determination of 13 fluoroquinolone and 22 sulfonamide residues in milk by a dual-colorimetric enzyme-linked immunosorbent assay. Analytical Chemistry, 85(4), 1995–1999.
- Jin-Bo, D., Zhen-Lin, X., Feng-Yin, L., Jin-Yi, Y., Yuan-Ming, S., Hong, W., … Yu-Dong, S. (2015). Determination of furaltadone metabolite in fish by chemiluminescence enzyme immunoassay. Chinese Journal of Analytical Chemistry, 43(6), 871–875.
- Jo, M. R., Son, K. T., Kwon, J. Y., Mok, J. S., Park, H. J., Kim, H. Y., … Lee, T. S. (2015). A lateral flow immunoassay kit for detecting residues of four groups of antibiotics in farmed fish. Korean Journal of Fisheries and Aquatic Sciences, 48(2), 158–167.
- Jornet, D., González-Martínez, M. A., Puchades, R., & Maquieira, A. (2010). Antibiotic immunosensing: Determination of sulfathiazole in water and honey. Talanta, 81(4-5), 1585–1592.
- Khoshbin, Z., Verdian, A., Housaindokht, M. R., Izadyar, M., & Rouhbakhsh, Z. (2018). Aptasensors as the future of antibiotics test kits-a case study of the aptamer application in the chloramphenicol detection. Biosensors and Bioelectronics, 122, 263–283.
- Kim, S., & Lim, H. (2015). Chemiluminescence immunoassay using magnetic nanoparticles with targeted inhibition for the determination of ochratoxin A. Talanta, 140, 183–188.
- Le, T., Yi, S.-H., Zhao, Z.-W., & Wei, W. (2011). Rapid and sensitive enzyme-linked immunosorbent assay and immunochromatographic assay for the detection of chlortetracycline residues in edible animal tissues. Food Additives & Contaminants: Part A, 28(11), 1516–1523.
- Le, T., Yu, H., Zhao, Z., & Wei, W. (2012). Development of a monoclonal antibody-based eLISA for the detection of oxytetracycline and 4-epi-oxytetracycline residues in chicken tissues. Analytical Letters, 45(4), 386–394.
- Li, C., Zhang, Y., Eremin, S. A., Yakup, O., Yao, G., & Zhang, X. (2017). Detection of kanamycin and gentamicin residues in animal-derived food using IgY antibody based ic-eLISA and fPIA. Food Chemistry, 227, 48–54.
- Li, X., Shen, J., Wang, Q., Gao, S., Pei, X., Jiang, H., & Wen, K. (2015). Multi-residue fluorescent microspheres immunochromatographic assay for simultaneous determination of macrolides in raw milk. Analytical and Bioanalytical Chemistry, 407(30), 9125–9133.
- Li, X., Wen, K., Chen, Y., Wu, X., Pei, X., Wang, Q., … Shen, J. (2015). Multiplex immunogold chromatographic assay for simultaneous determination of macrolide antibiotics in raw milk. Food Analytical Methods, 8(9), 2368–2375.
- Li, Y.-F., Sun, Y.-M., Beier, R. C., Lei, H.-T., Gee, S., Hammock, B. D., … Shen, Y.-D. (2016). Immunochemical techniques for multianalyte analysis of chemical residues in food and the environment: A review. TrAC Trends in Analytical Chemistry, 88, 25–40.
- Liu, N., Song, S., Lu, L., Nie, D., Han, Z., Yang, X., … Zheng, X. (2014). A rabbit monoclonal antibody-based sensitive competitive indirect enzyme-linked immunoassay for rapid detection of chloramphenicol residue. Food and Agricultural Immunology, 25(4), 523–534.
- Losoya-Leal, A., Estevez, M.-C., Martínez-Chapa, S. O., & Lechuga, L. M. (2015). Design of a surface plasmon resonance immunoassay for therapeutic drug monitoring of amikacin. Talanta, 141, 253–258.
- Luo, Y., Xu, J., Li, Y., Gao, H., Guo, J., Shen, F., & Sun, C. (2015). A novel colorimetric aptasensor using cysteamine-stabilized gold nanoparticles as probe for rapid and specific detection of tetracycline in raw milk. Food Control, 54, 7–15.
- Luo, P. J., Zhang, J. B., Wang, H. L., Chen, X., Y, N. W. U., Zhao, F., … Zhu, L. (2016). Rapid and sensitive chemiluminescent enzyme immunoassay for the determination of neomycin residues in milk. Biomedical and Environmental Sciences, 29(5), 374–378.
- Mala, J., Puthong, S., Maekawa, H., Kaneko, Y., Palaga, T., Komolpis, K., & Sooksai, S. (2017). Construction and sequencing analysis of scFv antibody fragment derived from monoclonal antibody against norfloxacin (Nor155). Journal of Genetic Engineering and Biotechnology, 15(1), 69–76.
- Meyer, M., Bumgarner, J., Varns, J. L., Daughtridge, J., Thurman, E., & Hostetler, K. A. (2000). Use of radioimmunoassay as a screen for antibiotics in confined animal feeding operations and confirmation by liquid chromatography/mass spectrometry. Science of the Total Environment, 248(2), 181–187.
- Meyer, V. K., Meloni, D., Olivo, F., Märtlbauer, E., Dietrich, R., Niessner, R., & Seidel, M. (2017). Validation procedure for multiplex antibiotic immunoassays using flow-based chemiluminescence microarrays. In Small molecule microarrays (Vol. 1518, pp. 195–212). Munich, Germany: Springer.
- Mi, T., Wang, Z., Eremin, S. A., Shen, J., & Zhang, S. (2013). Simultaneous determination of multiple (fluoro) quinolone antibiotics in food samples by a one-step fluorescence polarization immunoassay. Journal of Agricultural and Food Chemistry, 61(39), 9347–9355.
- Mitchell, J., Griffiths, M., McEwen, S., McNab, W., & Yee, A. (1998). Antimicrobial drug residues in milk and meat: Causes, concerns, prevalence, regulations, tests, and test performance. Journal of Food Protection, 61(6), 742–756.
- NaVrátiloVá, P. (2008). Screening methods used for the detection of veterinary drug residues in raw cow milk–a review. Czech Journal of Food Sciences, 26(6), 393–401.
- Ning, J., Ahmed, S., Cheng, G., Chen, T., Wang, Y., Peng, D., & Yuan, Z. (2019). Analysis of the stability and affinity of blaR-CTD protein to β-lactam antibiotics based on docking and mutagenesis studies. Journal of Biological Engineering, 13(1), 27.
- O’Farrell, B. (2009). Evolution in lateral flow–based immunoassay systems. In Lateral flow immunoassay (pp. 1–33). Carlsbad: Springer.
- O’Mahony, J., Moloney, M., McConnell, R. I., Benchikh, E. O., Lowry, P., Furey, A., & Danaher, M. (2011). Simultaneous detection of four nitrofuran metabolites in honey using a multiplexing biochip screening assay. Biosensors and Bioelectronics, 26(10), 4076–4081.
- Oruc, H. H., Rumbeiha, W. K., Ensley, S., Olsen, C., & Schrunk, D. E. (2013). Simultaneous detection of Six different groups of antimicrobial drugs in porcine oral fluids using a biochip array-based immunoassay. Kafkas Universitesi Veteriner Fakultesi Dergisi, 19(3), 407–412.
- Pastor-Navarro, N., Morais, S., Maquieira, A., & Puchades, R. (2007). Synthesis of haptens and development of a sensitive immunoassay for tetracycline residues: application to honey samples. Analytica Chimica Acta, 594(2), 211–218.
- Peng, J., Cheng, G., Huang, L., Wang, Y., Hao, H., Peng, D., … Yuan, Z. (2013). Development of a direct eLISA based on carboxy-terminal of penicillin-binding protein blaR for the detection of β-lactam antibiotics in foods. Analytical and Bioanalytical Chemistry, 405(27), 8925–8933.
- Pennacchio, A., Varriale, A., Esposito, M. G., Scala, A., Marzullo, V. M., Staiano, M., & D’Auria, S. (2015). A rapid and sensitive assay for the detection of benzylpenicillin (penG) in milk. PloS One, 10(7), e0132396.
- Popa, I. D., Schiriac, E. C., & Cuciureanu, R. (2012). Multi-analytic detection of antibiotic residues in honey using a multiplexing biochip assay. Revista Medico-Chirurgicala a Societatii de Medici si Naturalisti din Iasi, 116(1), 324–329.
- Posthuma-Trumpie, G. A., Korf, J., & van Amerongen, A. (2009). Lateral flow (immuno) assay: Its strengths, weaknesses, opportunities and threats. A literature survey. Analytical and Bioanalytical Chemistry, 393(2), 569–582.
- Renson, C., Degand, G., Maghuin-Rogister, G., & Delahaut, P. (1993). Determination of sulphamethazine in animal tissues by enzyme immunoassay. Analytica Chimica Acta, 275(1), 323–328.
- Sánchez-Martínez, M., Aguilar-Caballos, M., & Gómez-Hens, A. (2009). Long-wavelength homogeneous enzyme immunoassay for the determination of amikacin in water samples. Talanta, 78(1), 305–309.
- Shanin, I., Shaimardanov, A., Thai, N. T. D., & Eremin, S. (2015). Determination of fluoroquinolone antibiotic levofloxacin in urine by fluorescence polarization immunoassay. Journal of Analytical Chemistry, 70(6), 712–717.
- Smith, D. S., & Eremin, S. A. (2008). Fluorescence polarization immunoassays and related methods for simple, high-throughput screening of small molecules. Analytical and Bioanalytical Chemistry, 391(5), 1499–1507.
- Song, E., Yu, M., Wang, Y., Hu, W., Cheng, D., Swihart, M. T., & Song, Y. (2015). Multi-color quantum dot-based fluorescence immunoassay array for simultaneous visual detection of multiple antibiotic residues in milk. Biosensors and Bioelectronics, 72, 320–325.
- Spinks, C., Schut, C., Wyatt, G., Spinks, C., & Morgan, C. (2001). Development of an eLISA for sulfachlorpyridazine and investigation of matrix effects from different sample extraction procedures. Food Additives & Contaminants, 18(1), 11–18.
- Squadrone, S., Marchis, D., Loria, A., Amato, G., Ferro, G. L., & Abete, M. C. (2015). Detection of banned antibacterial growth promoter in animal feed by enzyme-linked immunosorbent assay: method validation according to the commission decision 2002/657/EC criteria. Food Control, 47, 66–70.
- Tao, X., Jiang, H., Zhu, J., Niu, L., Wu, X., Shi, W., … Shen, J. (2012). Detection of ultratrace chloramphenicol residues in milk and chicken muscle samples using a chemiluminescent eLISA. Analytical Letters, 45(10), 1254–1263.
- Tao, X., Shen, J., Cao, X., Wang, Z., Wu, X., & Jiang, H. (2014). Simultaneous determination of chloramphenicol and clenbuterol in milk with hybrid chemiluminescence immunoassays. Analytical Methods, 6(4), 1021–1027.
- Thakur, G., Vishweswaraiah, R. H., Tehri, N., Kumar, N., Yadav, A., & Malik, R. K. (2014). Biochip based detection-An emerging tool for ensuring safe milk: A review. Journal of Innovative Biology, 1(3), 147–154.
- Tufa, R. A., Pinacho, D. G., Pascual, N., Granados, M., Companyó, R., & Marco, M. P. (2015). Development and validation of an enzyme linked immunosorbent assay for fluoroquinolones in animal feeds. Food Control, 57, 195–201.
- Van Herwijnen, R., & Baumgartner, S. (2006). The use of lateral flow devices to detect food allergens. In Koppelman stef j & Hefle Sue L (Eds.), Detecting Allergens in food (pp. 175–181). Cambridge: Woodhead Publishing.
- Wang, Z., Beier, R. C., & Shen, J. (2017). Immunoassays for detection of macrocyclic lactones in food matrices—A review. TrAC Trends in Analytical Chemistry, 92, 42–61.
- Wang, Y., Gan, N., Zhou, Y., Li, T., Hu, F., Cao, Y., & Chen, Y. (2017). Novel label-free and high-throughput microchip electrophoresis platform for multiplex antibiotic residues detection based on aptamer probes and target catalyzed hairpin assembly for signal amplification. Biosensors and Bioelectronics, 97, 100–106.
- Wang, C., Li, X., Peng, T., Wang, Z., Wen, K., & Jiang, H. (2017). Latex bead and colloidal gold applied in a multiplex immunochromatographic assay for high-throughput detection of three classes of antibiotic residues in milk. Food Control, 77, 1–7.
- Wang, W., Liu, L., Xu, L., Ma, W., Kuang, H., & Xu, C. (2013). Detection of β-lactamase residues in milk by sandwich eLISA. International Journal of Environmental Research and Public Health, 10(7), 2688–2698.
- Wang, S., Liu, J., Yong, W., Chen, Q., Zhang, L., Dong, Y., … Tan, T. (2015). A direct competitive assay-based aptasensor for sensitive determination of tetracycline residue in honey. Talanta, 131, 562–569.
- Wang, Z., Mi, T., Beier, R. C., Zhang, H., Sheng, Y., Shi, W., … Shen, J. (2015). Hapten synthesis, monoclonal antibody production and development of a competitive indirect enzyme-linked immunosorbent assay for erythromycin in milk. Food Chemistry, 171, 98–107.
- Wang, S., Xu, B., Zhang, Y., & He, J. (2009). Development of enzyme-linked immunosorbent assay (eLISA) for the detection of neomycin residues in pig muscle, chicken muscle, egg, fish, milk and kidney. Meat Science, 82(1), 53–58.
- Wang, L., Zhang, Y., Gao, X., Duan, Z., & Wang, S. (2010). Determination of chloramphenicol residues in milk by enzyme-linked immunosorbent assay: improvement by biotin− streptavidin-amplified system. Journal of Agricultural and Food Chemistry, 58(6), 3265–3270.
- Wang, Z., Zhang, H., Ni, H., Zhang, S., & Shen, J. (2014). Development of a highly sensitive and specific immunoassay for enrofloxacin based on heterologous coating haptens. Analytica Chimica Acta, 820, 152–158.
- Wu, J.-X., Zhang, S.-e., & Zhou, X.-p. (2010). Monoclonal antibody-based eLISA and colloidal gold-based immunochromatographic assay for streptomycin residue detection in milk and swine urine. Journal of Zhejiang University Science B, 11(1), 52–60.
- Wutz, K., Niessner, R., & Seidel, M. (2011). Simultaneous determination of four different antibiotic residues in honey by chemiluminescence multianalyte chip immunoassays. Microchimica Acta, 173(1-2), 1–9.
- Xia, Y., Su, R., Huang, R., Ding, L., Wang, L., Qi, W., & He, Z. (2017). Design of elution strategy for simultaneous detection of chloramphenicol and gentamicin in complex samples using surface plasmon resonance. Biosensors and Bioelectronics, 92, 266–272.
- Yang, S., & Carlson, K. (2004). Routine monitoring of antibiotics in water and wastewater with a radioimmunoassay technique. Water Research, 38(14-15), 3155–3166.
- Yang, K., Hu, Y., & Dong, N. (2016). A novel biosensor based on competitive sERS immunoassay and magnetic separation for accurate and sensitive detection of chloramphenicol. Biosensors and Bioelectronics, 80, 373–377.
- Youn, H., Lee, K., Her, J., Jeon, J., Mok, J., So, J.-i., … Ban, C. (2019). Aptasensor for multiplex detection of antibiotics based on fRET strategy combined with aptamer/graphene oxide complex. Scientific Reports, 9(1), 1–9.
- Yu, X., Wen, K., Wang, Z., Zhang, X., Li, C., Zhang, S., & Shen, J. (2016). General bioluminescence resonance energy transfer homogeneous immunoassay for small molecules based on quantum dots. Analytical Chemistry, 88(7), 3512–3520.
- Zeng, H., Chen, J., Zhang, C., Huang, X.-a., Sun, Y., Xu, Z., & Lei, H. (2016). Broad-specificity chemiluminescence enzyme immunoassay for (fluoro) quinolones: hapten design and molecular modeling study of antibody recognition. Analytical Chemistry, 88(7), 3909–3916.
- Zhang, J., Wang, Z., Mi, T., Wenren, L., & Wen, K. (2014). A homogeneous fluorescence polarization immunoassay for the determination of cephalexin and cefadroxil in milk. Food Analytical Methods, 7(4), 879–886.
- Zhang, K., Gan, N., Shen, Z., Cao, J., Hu, F., & Li, T. (2019). Microchip electrophoresis based aptasensor for multiplexed detection of antibiotics in foods via a stir-bar assisted multi-arm junctions recycling for signal amplification. Biosensors and Bioelectronics, 130, 139–146.
- Zhang, Z., & Cheng, H. (2016). Recent development in sample preparation and analytical techniques for determination of quinolone residues in food products. Critical Reviews in Analytical Chemistry, 47(3), 223–250.
- Zhang, Z., & Cheng, H. (2017). Recent development in sample preparation and analytical techniques for determination of quinolone residues in food products. Critical Reviews in Analytical Chemistry, 47(3), 223–250.
- Zhang, L., Long, H., Li, X., Xu, K., Meng, M., Yin, Y., & Xi, R. (2016). Production of a sensitive antibody against sirolimus for chemiluminescence immunoassay potential in its therapeutic drug monitoring. Analytical Methods, 8(33), 6298–6304.
- Zhao, L., Sun, L., & Chu, X. (2009). Chemiluminescence immunoassay. TrAC Trends in Analytical Chemistry, 28(4), 404–415.
- Zhou, C., Zhang, X., Huang, X., Guo, X., Cai, Q., & Zhu, S. (2014). Rapid detection of chloramphenicol residues in aquatic products using colloidal gold immunochromatographic assay. Sensors, 14(11), 21872–21888.
- Zhou, J., Zhu, K., Xu, F., Wang, W., Jiang, H., Wang, Z., & Ding, S. (2014). Development of a microsphere-based fluorescence immunochromatographic assay for monitoring lincomycin in milk, honey, beef, and swine urine. Journal of Agricultural and Food Chemistry, 62(49), 12061–12066.
- Zhou, L., Gan, N., Hu, F., Li, T., Cao, Y., & Wu, D. (2018). Microchip electrophoresis array-based aptasensor for multiplex antibiotic detection using functionalized magnetic beads and polymerase chain reaction amplification. Sensors and Actuators B: Chemical, 263, 568–574.
- Zhou, L., Gan, N., Zhou, Y., Li, T., Cao, Y., & Chen, Y. (2017). A label-free and universal platform for antibiotics detection based on microchip electrophoresis using aptamer probes. Talanta, 167, 544–549.