ABSTRACT
A rapid immunochromatographic lateral flow strip test (ILFST) of competitive format for the detection of diclofenac (DCF) in medicinal wine samples was developed. The half-inhibitory concentration (IC50) values of the ILFST for DCF and the limit of detection (LOD) in DCF-spiked samples were 0.66 and 0.15 ng/mL, respectively. Recoveries of DCF from spiked medicinal wine samples were from 84.4% to 85.9% within an assay (intra-assay) and from 83.7% to 88.4% between assays (inter-assay). The coefficients of variation (CV) for intra-assay and inter-assay were 4.10–7.56% and 4.71–8.94%, respectively. These results indicated that the ILFST was highly sensitive and re-producible. The same authentic medicinal wine samples were tested by both ILFST and high-performance liquid chromatography (HPLC) respectively. The results showed no significant differences between the two methods. Therefore, the ILFST can be widely used as a convenient tool for both qualitative and quantitative detection of DCF residues in medicinal wine samples.
Introduction
Diclofenac [DCF, 1-(2,6-Dichlorophenyl)-2-indolinone], a nonsteroidal anti-inflammatory drug used to alleviate pain, fever, and inflammation associated with arthritis, rheumatoid arthritis, osteoarthritis, acute gout, and dysmenorrhea (Savaşer, Ozkan, & Işimer, Citation2005), was commonly used antipyretic analgesic for the rapid onset and low cost of treating rheumatic diseases. In China, some unscrupulous manufacturers have added diclofenac sodium to medicinal wine to enhance efficacy and gain illegitimate benefits. DCF is mainly absorbed in the gastrointestinal tract and long-term use of medicinal wine containing diclofenac causes adverse health effects in humans such as gastrointestinal ulceration, renal toxicity, and platelet dysfunction (Aygün, Kaplan, Odaci, Onger, & Altunkaynak, Citation2012). On the other hand, because of its polar nature, DCF cannot be effectively removed by wastewater treatment and subsequently continue to endanger the health of fish and birds (Zhang, Geissen, & Gal, Citation2008; Ziylan, Koltypin, Gedanken, & Ince, Citation2013).
There are many analytical methods for the determination of DCF in biological samples, such as high-performance liquid chromatography (HPLC) (Madikizela & Chimuka, Citation2017), HPLC with ultra-violet detection (Payán et al., Citation2009) or electrochemical detection (Chmielewska, Konieczna, Plenis, Bieniecki, & Lamparczyk, Citation2006), liquid chromatography-mass spectrometry (LC-MS) (Botitsi, Frosyni, & Tsipi, Citation2007), and gas chromatographic-mass spectrometry (GC-MS) (Shah, Barker, Naughton, Barton, & Ashraf, Citation2016). Although these methods are rather sensitive and specific for detecting DCF, they are time-consuming, laborious, and limited to laboratory use due to their dependency on sample pretreatment (separation, extraction, and derivatization), expensive instruments, and skilled professionals. In contrast, immunoassays, based on the specific reaction between antigen and antibody, can overcome some of these drawbacks. Thus, the enzyme-linked immunosorbent assays (ELISAs) (Huebner, Weber, Niessner, Boujday, & Knopp, Citation2015) and Upconversion-Linked immunosorbent assays (ULISAs) (Hlaváček et al., Citation2016) were developed for the detection of DCF in wastewater, livestock carcasses, and other biological samples (Saini et al., Citation2012). ELISAs eliminate sophisticated sample pretreatment and can tolerate certain matrix interferences because of washes between steps. Compared with ELISAs, immunochromatographic lateral flow strip test (ILFST) is simpler and rapid immunoassay that has been used in detecting pesticides (Byzova, Urusov, Zherdev, & Dzantiev, Citation2018; Ismail et al., Citation2016), veterinary drugs (Chen et al., Citation2017; Shelver & Smith, Citation2018), mycotoxins (Di Nardo et al., Citation2019; Majdinasab, Zareian, Zhang, & Li, Citation2019), bacteria (Canver et al., Citation2019; Saleh, Göttig, & Hamprecht, Citation2018), and viruses (Lyoo et al., Citation2017; Senthilkumaran et al., Citation2017). ILFST integrates all the required reagents into itself and can provide the results within 10 min that can be determined both qualitatively and quantitatively with unaided eyes and a strip reader, respectively. To our knowledge, no ILFST has been reported for the DCF residue so far.
In this study, we aimed to develop an ILFST for the rapid detection of DCF in medicinal wines. DCF was conjugated with bovine serum albumin (BSA) and ovalbumin (OVA) through the activated ester (AE) method and the mixed anhydride (MA) method. Anti-DCF monoclonal antibody (mAb) was produced and then conjugated with colloidal gold nanoparticles for the probe. The ILFST was developed based on the competitive format and validated in terms of specificity, sensitivity, accuracy, and precision in spike recovery experiments, and compared with HPLC for detection in authentic medicinal wine samples.
Materials and methods
Chemicals and materials
DCF, N-Hydroxy succinimide (NHS), N,N-dimethylformamide (DMF), Na2B4O7, tetramethylene oxide, 1-(3-(dimethylamino) propyl)-3-ethylcarbodiimide hydrochloricde (EDC), tributylamine, isobutyl chlorocarbonate, and a mouse monoclonal antibody isotyping kit were purchased from Sigma (St Louis, MO, USA). Ketoprofen, aspirin, indomethacin, ibuprofen, sulindac, naproxen, rofecoxib were purchased from Macklin Biochemical Co., Ltd (Shanghai, China), Bovine serum albumin (BSA) and ovalbumin (OVA) were purchased from Yuanye Biotechnology Co., Ltd (Shanghai, China). HRP conjugated goat-anti-mouse immunoglobulin G antibody was obtained from Sino-American Biotechnology Co., Ltd (Luoyang, China).
Preparation of immunogen and coating antigen
DCF-BSA and DCF-OVA conjugates were prepared using two methods: the AE method and the MA method. In the AE method, according to a previous report (Pinacho, Sánchez-Baeza, & Marco, Citation2012), 15 mg DCF was dissolved in 3 mL DMF and mixed with 6 mg NHS and 10 mg EDC at room temperature (RT) for 12 h. The mixture was centrifuged at 4000 rpm for 5 min to collect the supernatant. The active final products were harvested through rotary evaporation and dissolved in 1 mL DMSO before the addition of 2 mL BSA solution (42 mg dissolved in 0.01 M PBS) or OVA solution (28 mg dissolved in 0.01 M PBS) in a dropwise manner. In the MA method (Wainer, Fitch, Rothberg, & Fried, Citation1972), 15 mg DCF was dissolved in 2 mL tetramethylene oxide and stirred in an ice bath for 20 min after the addition of 27 μL tributylamine. Then the mixture was supplemented with 16 μL isobutyl chlorocarbonate and further stirred at RT for 3 h. Finally, 3 mL pre-cooled BSA solution (42 mg dissolved in 0.1 M Na2B4O7) or OVA solution (28 mg dissolved in 0.1 M Na2B4O7) was added dropwise to the mixture and stirred at RT for 24 h. In both methods, the final mixture was dialysed against PBS and centrifuged at 3000 rpm for 20 min at 4°C. The supernatant was collected and stored at–20°C. Ultra-violet scanning was used to judge the conjugation ratio between DCF with BSA or OVA, respectively. DCF-AE-BSA and DCF-MA-BSA, prepared by the two methods, were used as immunogen to immunize BALB/c mice respectively. Indirect and indirect competitive ELISAs were performed by microplate readers (Multiskan FC, Thermo Fisher Scientific Instrument Co., Ltd., Shanghai, China) using the corresponding DCF-OVA conjugates as coating antigens.
Production of anti-DCF mAb
BALB/c mice were subcutaneously immunized with 65 µg immunogen five times at intervals of 3 weeks. Serum antibodies were collected and detected by indirect and competitive indirect ELISAs as previously described (Sun et al., Citation2014). The mouse giving the best half-maximal inhibitory concentration (IC50) in competitive indirect ELISA was selected to be vaccinated intraperitoneally with 100 µg immunogen before cell fusion. Hybridomas secreting anti-DCF mAbs were selected by competitive indirect ELISA and cultured to prepare ascites in paraffin-primed mice. Affinity and isotype of the mAbs were characterized using ELISA and a mouse mAb isotyping kit (Sigma, USA).
Conjugation of anti-DCF mAb with colloidal gold and preparation of ILFST
Anti-DCF mAb 4B9 was labelled with colloidal gold produced via reduction of HAuCl4 with 1% sodium citrate as reducing agent (Wang et al., Citation2019b). Antibody concentration that can prevent the aggregation of gold nanoparticles in the presence of 10% NaCl was selected for use and pH value was adjusted with 0.2 M K2CO3. In the labelling, 1 ml mAb 4B9 solution (2 μg/mL) and 5 mL colloidal gold solution (pH 9.0) were incubated for 20 min at RT. The mixture was incubated at RT for another 10 min with 1 mL of 10% BSA. The labelled mAb was then washed twice, and then centrifugated at 15,000 × g for 30 min at 4°C. The precipitant was finally resuspended in aqueous solutions of trehalose plus BSA and stored at 4°C before use.
Test procedure and principle
The ILFST was assembled as previously described (Khaemba et al., Citation2016; Wang et al., Citation2019a). The sample solution loads onto the sample pad, and then migrates toward the absorbent pad by capillary effect. If DCF is present in the sample, it may inhibit the binding of colloidal gold-labelled 4B9 with DCF-BSA on the test line (T line), thus, the colour intensity of the T line is reduced. Within the linear range, the concentration of DCF in the sample is negative correlated with the intensity of the red of the T line. One way or another, a visible red of the control line (C line) should always appear to indicate the validation of the ILFST. If no C line appear, the test is considered as invalid and should be performed again with another test strip ().
Sample pretreatment for the LFIST
Anti-rheumatic medicinal wine was purchased from a local market, and was tested to be negative by HPLC. The stock solution was prepared by diluting 2 mL medicinal wine in 8 mL PBS and storing at 4°C. Before the strip test, the stock solution was filtered by 0.22 um of microporous membrane.
Evaluation of the performance of LFIST
The sensitivity of the LFIST was judged by detecting DCF-free medicinal wine samples spiked with 0, 0.25, 0.5, 1.0, 2.0, 4.0 and 8.0 ng/mL of DCF. Each test was repeated in triplicate. Qualitative detection was realized by judging the presence of the T line by two people. For quantitative detection, relative optical density (ROD) of the T line was read by a TSR3000 membrane strip reader (Bio-Rad, USA). A standard curve was constructed by plotting the B/B0 value toward the concentrations of the spiked samples. B and B0 represented the ROD values obtained from testing spiked samples and the blank sample, respectively. The value of IC50 was extrapolated by the linear regression equation.
Specificity of the LFIST was evaluated by testing structural analogues of DCF including ketoprofen, aspirin, indomethacin, ibuprofen, sulindac, naproxen, and rofecoxib. DCF and the structural analogues in the negative wine samples were tested at a concentration of 1000 ng/mL, respectively. IC50 and cross-reactivity (CR) for each analyte were calculated and compared. CR was calculated using the equation: CR (%) = (IC50 of DCF)/(IC50 of the competitor) × 100.
The accuracy and precision of the ILFST were evaluated by detecting in medicinal wine samples containing 1.8, 3.6, and 7.2 ng/ml of DCF using the test strip, and were expressed as recovery and coefficient of variation (CV, %), respectively. For inter-assay precision, six batches of the ILFST were used to test samples of different concentrations in sextuplicate.
Authenticity of ILFST
Health wine samples, with five different DCF concentrations (2.4, 4.8, 9.6, 19.2, and 38.4 ng/ mL), were authenticated to be negative by LC-MS. These samples were detected in parallel by ILFST and HPLC, separately. HPLC was performed for instrumental analysis of DCF using Diamonsil C18 (250 × 4.6 mm × 5 μm) obtained from Dima Technology Co., Ltd. (Beijing, China). The mobile phases were an acetonitrile: 0.2% formic acid (60:40, v/v), flow rate of 0.8 mL/min, column temperature was 25°C, and DCF was monitored at 200 nm. Tested values obtained from the ILFST and HPLC were compared to the given concentrations using a one-sample T-test, and the values between the ILFST and HPLC, presented as means SE, were analysed by independent sample T-test. Differences between the test values were considered statistically significant at P < 0.05.
Results and discussion
Preparation of complete antigen
The immunogen DCF-AE-BSA and coating antigen DCF-AE-OVA were both synthesized using the AE method ((a)), and DCF-MA-BSA and coating antigen DCF-MA-OVA synthesized by the MA method ((b)). After UV scanning, the Coupling rates of DCF with BSA and OVA were calculated as 21.6:1 and 16.5:1, respectively, in the AE (EDC/NHS) preparation. The Coupling rates of DCF with BSA and OVA were calculated as 17.2:1 and 15.9:1, respectively, in the MA preparation. Upon comparison of serum antibody titre and IC50 value (), the mouse that showed an antibody titre of 1:5.12 × 104 and an IC50 value of 7.89 ng/ mL was chosen for subsequent cell fusion, which was immunized with DCF-AE-BSA prepared using the AE method.
Figure 2. (a) Synthesis of DCF-BSA using activated ester method. (b) Synthesis of DCF-BSA using mixed anhydride method.
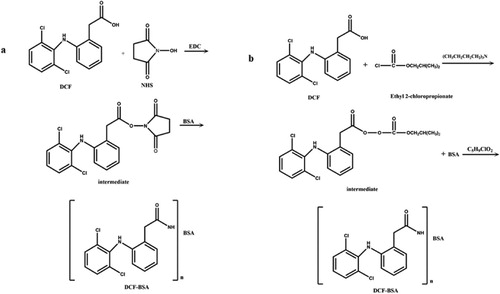
Table 1. Characterization of the optimal antiserum produced by MA and AE methods toward free DCF and coating antigens.
Characterization of mAb against DCF
After cell fusion and screening by direct and indirect competitive ELISAs, four hybridomas cell lines named 2F4, 3A7, 3D4, and 4B9 were selected. Affinity constant, antibody titre, and IC50 value of each mAb were determined by ELISA (). mAb 4B9, which showed an antibody titre of 1:1,024,000 and an IC50 value of 0.61 ng/mL, was selected to be labelled with colloidal gold nanoparticles. The affinity constant (Ka) and the subtype of 4B9 was determined to be 1.5 × 1010 L/mol and immunoglobulin G 1, respectively.
Table 2. Characterization of anti-DCF mAbs by ELISA.
Sensitivity of the ILFST
Reference solutions of DCF at concentrations of 0, 0.25, 0.5, 1.0, 2.0, 4.0 and 8.0 ng/mL were analysed using the ILFST. The ROD value for each test was obtained by scanning the T line with a TSR3000 membrane strip reader () and are quantified in . A quantitative calibration curve was constructed by drawing the value of B/B0 against the concentrations of DCF in medicinal wine samples (). ROD values decreased in a linear manner as the final DCF concentration in spiked samples increased from 0.0 to 8.0 ng/mL. According to the linear equation, IC50 was calculated as 0.66 ng/mL, with the coefficient of determination (R2) = 0.9933. The limit of detection (LOD) of the ILFST was determined to be 0.15 and 1.0 ng/mL with unaided visual assessment (). High sensitivity of the ILFST reflected the high binding affinity between mAb 4B9 and DCF.
Figure 3. ROD curves from detections of different spiked health wine samples. Blank health wine samples were spiked with DCF to final concentrations of 0, 0.25, 0.50, 1.0, 2.0, 4.0 and 8.0 ng/ml and tested using the ILFST. Relative optical density (ROD) of the test line was read by a TSR3000 membrane strip reader (Bio-Dot).
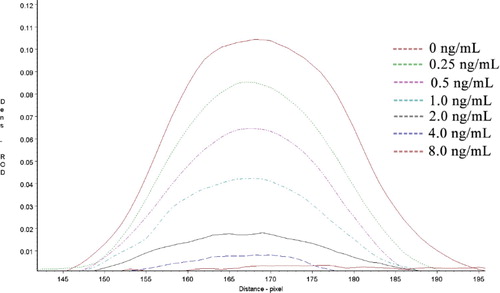
Figure 4. The quantitative calibration curve drawn from testing different DCF standard solutions using ILFST. The X-axis represents the concentrations of DCF in spiked samples. B/B0 represents the percentage of ROD values obtained from spiked samples divided by that of the blank sample.
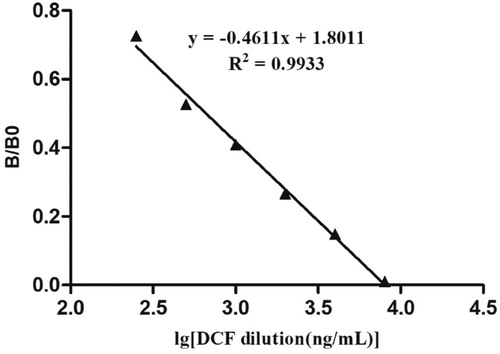
Table 3. Relative optical density from testing spiked health wine samples using ILFST
Specificity of the ILFST
To determine the specificity of the ILFST, CR was evaluated by testing structural analogues of DCF including ketoprofen, aspirin, indomethacin, ibuprofen, sulindac, naproxen, and rofecoxib. When these structural analogues were added at a final concentration of 1000 ng/ml, the colour of T lines remained negative. Determinations of the IC50 values for each competitor and calculations of CR showed that the mAb 4B9 based ILFST had less than 0.1% CR with these competitors ().
Table 4. CR of ILFST with competitors.
A DCF-thyroglobulin conjugate was synthesized by EDC/NHS method, and four mice were immunized to obtain antisera. An immunized mouse giving the highest antisera titre was used for hybridization, and its spleen cells were fused with myeloma cells. A generic mAb 12G5 against DCF was prepared. The LOD, IC50 and the range of detection of competitive indirect ELISA were 7.8, 44 and 11–180 ng/L, respectively (Huebner et al., Citation2015). The CR of mAb 12G5 was 8.5–13% for DCF metabolites, but less than 1% for the structural analogues. The competitive indirect ELISA was applied for detection of DCF residues in river and lake water. Although this ELISA is highly sensitive and specific, it requires too many operation steps comparing to ILFST.
Accuracy and precision of the ILFST
The accuracy and precision of the ILFST were determined by detecting in medicinal wine samples containing 1.8, 3.6, and 7.2 ng/ mL of DCF using the test strip and expressed as recovery and CV, respectively. As shown in , recoveries ranged from 84.4 to 86.8% within an assay (intra-assay) and from 83.7 to 88.4% between assays (inter-assay), respectively. Both assay CV of the ILFST were less than 9.0%.
Table 5. Accuracy and precision of the ILFST.
Comparison of ILFST with HPLC
A comparison between ILFST and HPLC was performed by testing medicinal wine samples. The results showed that there was no significant difference between the ILFST with HPLC (), which means that the values tested by ILFST were reliable.
Table 6. Comparison between the ILFST and HPLC.
Conclusions
An ILFST was established using a high-affinity, specificity mAbs 4B9 against DCF for detecting DCF residues. For DCF, the IC50 was 0.66 ng/mL, and the LOD was 0.15 ng/mL in the reference solutions. The recoveries from medicinal wine samples were found to be from 84.4 to 85.9% within an assay (intra-assay) and from 83.7% to 88.4% between assays (inter-assay). The major advantages of the ILFST are relative cost-effectiveness, it is faster than chromatographic instrument analysis, and is easy to operate by professionals. Therefore, the ILFST is a convenient screening tool for detecting DCF residues in medicinal wine samples.
Ethical statement
The use of all BALB/c mice in this study was approved by the Animal Ethics Committee of Zhoukou Normal University and in accordance with all applicable institutional and governmental regulations concerning the ethical use of animals.
Disclosure statement
No potential conflict of interest was reported by the authors.
Additional information
Funding
References
- Aygün, D., Kaplan, S., Odaci, E., Onger, M. E., & Altunkaynak, M. E. (2012). Toxicity of non-steroidal anti-inflammatory drugs: A review of melatonin and diclofenac sodium association. Histology and Histopathology, 27, 417–436. doi:10.1016/j. cellsig.2011.12.010
- Botitsi, E., Frosyni, C., & Tsipi, D. (2007). Determination of pharmaceuticals from different therapeutic classes in wastewaters by liquid chromatography–electrospray ionization–tandem mass spectrometry. Analytical and Bioanalytical Chemistry, 387, 1317–1327. doi: 10.1007/s00216-006-0804-8
- Byzova, N. A., Urusov, A. E., Zherdev, A. V., & Dzantiev, B. B. (2018). Multiplex highly sensitive immunochromatographic assay based on the use of nonprocessed antisera. Analytical and Bioanalytical Chemistry, 410, 1903–1910. doi:10.1007/s00 216-018-0853-9 doi: 10.1007/s00216-018-0853-9
- Canver, M. C., Gonzalez, M. D., Ford, B. A., Arnold, A. R., Lawhon, S. D., Burnham, C. A., … Westblade, L. F. (2019). Improved performance of a rapid immunochromatographic assay for the detection of PBP2a in non-Staphylococcus aureus Staphylococcal Species. Journal of Clinical Microbiology, 57, e01417–e01418. doi: 10.1128/JCM.01417-18
- Chen, Y., Guo, L., Liu, L., Song, S., Kuang, H., & Xu, C. (2017). Ultrasensitive immunochromatographic strip for Fast screening of 27 Sulfonamides in Honey and Pork Liver samples based on a monoclonal antibody. Journal of Agricultural and Food Chemistry, 65, 8248–8255. 10.1021/acs.jafc.7b03190
- Chmielewska, A., Konieczna, L., Plenis, A., Bieniecki, M., & Lamparczyk, H. (2006). Determination of diclofenac in plasma by high-performance liquid chromatography with electrochemical detection. Biomedical Chromatography, 20, 119–124. doi: 10.1002/bmc.537
- Di Nardo, F., Alladio, E., Baggiani, C., Cavalera, S., Giovannoli, C., Spano, G., & Anfossi, L. (2019). Colour-encoded lateral flow immunoassay for the simultaneous detection of aflatoxin B1 and type-B fumonisins in a single test line. Talanta, 192, 288–294. doi: 10.1016/j.talanta.2018.09.037
- Hlaváček, A., Farka, Z., Hübner, M., Horňáková, V., Němeček, D., Niessner, R., … Gorris, H. H. (2016). Competitive Upconversion-linked immunosorbent assay for the sensitive detection of diclofenac. Analytical Chemistry, 88, 6011–6017. doi: 10.1021/acs.analchem.6b01083
- Huebner, M., Weber, E., Niessner, R., Boujday, S., & Knopp, D. (2015). Rapid analysis of diclofenac in freshwater and wastewater by a monoclonal antibody-based highly sensitive ELISA. Analytical and Bioanalytical Chemistry, 407, 8873–8882. doi: 10.1007/s00216-015-9048-9
- Ismail, H. M., Kumar, V., Singh, R. P., Williams, C., Shivam, P., Ghosh, A., … Paine, M. J. (2016). Development of a Simple Dipstick assay for Operational Monitoring of DDT. PLoS Neglected Tropical Diseases, 10, e0004324. doi: 10.1371/journal.pntd.0004324
- Khaemba, G. W., Tochi, B. N., Mukunzi, D., Joel, I., Lingling Guo, L., & Xu, C. (2016). Development of monoclonal antibody and lateral test strip for sensitive detection of clenbuterol and related β2-agonists in urine samples. Food and Agricultural Immunology, 27, 111–127. doi: 10.1080/09540105.2015.1079598
- Lyoo, K. S., Yeom, M., Kim, J., Kim, D., Ha, G., Na, W., … Song, D. (2017). Development of rapid immunochromatographic strip test for the detection of porcine epidemic diarrhoea virus. Veterinary Record, 181, 596. doi: 10.1136/vr.103959
- Madikizela, L. M., & Chimuka, L. (2017). Occurrence of naproxen, ibuprofen, and diclofenac residues in wastewater and river water of KwaZulu-Natal Province in South Africa. Environmental Monitoring and Assessment, 189, 348. doi: 10.1007/s10661-017-6069-1
- Majdinasab, M., Zareian, M., Zhang, Q., & Li, P. (2019). Development of a new format of competitive immunochromatographic assay using secondary antibody-europium nanoparticle conjugates for ultrasensitive and quantitative determination of ochratoxin A. Food Chemistry, 275, 721–729. doi:10.1016/j.foodchem.2018. 09.112 doi: 10.1016/j.foodchem.2018.09.112
- Payán, R., Bello López, M., Fernández-Torres, M. A., Pérez Bernal, R., L, J., & Callejón Mochón, M. (2009). HPLC determination of ibuprofen, diclofenac and salicylic acid using hollow fiber-based liquid phase microextraction (HF-LPME). Analytica Chimica Acta, 653, 184–190. doi: 10.1016/j.aca.2009.09.018
- Pinacho, D. G., Sánchez-Baeza, F., & Marco, M. P. (2012). Molecular modeling assisted hapten design to produce broad selectivity antibodies for fluoroquinolone antibiotics. Analytical Chemistry, 84, 4527–4534. doi: 10.1021/ac300263m
- Saini, M., Taggart, M. A., Knopp, D., Upreti, S., Swarup, D., Das, A., … Cuthbert, R. J. (2012). Detecting diclofenac in livestock carcasses in India with an ELISA: A tool to prevent widespread vulture poisoning. Environmental Pollution, 160, 11–16. doi: 10.1016/j.envpol.2011.09.011
- Saleh, A., Göttig, S., & Hamprecht, A. G. (2018). Multiplex immunochromatographic detection of OXA-48, KPC, and NDM Carbapenemases: Impact of Inoculum, Antibiotics, and Agar. Journal of Clinical Microbiology, 56, JCM: e00050–18. doi: 10.1128/JCM.00050-18
- Savaşer, A., Ozkan, Y., & Işimer, A. (2005). Preparation and in vitro evaluation of sustained release tablet formulations of diclofenac sodium. IL Farmaco, 60, 171–177. doi: 10.1016/j.farmac.2004.10.001
- Senthilkumaran, C., Yang, M., Bittner, H., Ambagala, A., Lung, O., Zimmerman, J., … Nfon, C. (2017). Detection of genome, antigen, and antibodies in oral fluids from pigs infected with foot-and-mouth disease virus. Canadian Journal of Veterinary Research, 81, 82–90. Pmid: 28408775.
- Shah, I., Barker, J., Naughton, D. P., Barton, S. J., & Ashraf, S. S. (2016). Determination of diclofenac concentrations in human plasma using a sensitive gas chromatography mass spectrometry method. Chemistry Central Journal, 10, 52. doi: 10.1186/s13065-016-0199-3
- Shelver, W. L., & Smith, D. J. (2018). Development of an immunochromatographic assay for the β-adrenergic agonist feed additive zilpaterol. Food Additives & Contaminants: Part A, 35, 1519–1529. doi: 10.1080/19440049.2018.1463568
- Sun, Y., Hu, X., Zhang, Y., Yang, J., Wang, F., Wang, Y., … Zhang, G. (2014). Development of an immunochromatographic strip test for the rapid detection of zearalenone in corn. Journal of Agricultural and Food Chemistry, 62, 11116–11121. doi: 10.1021/jf503092j
- Wainer, B. H., Fitch, F. W., Rothberg, R. M., & Fried, J. (1972). Morphine-3-succinyl–bovine serum albumin: An immunogenic hapten-protein conjugate. Science, 176, 1143–1145. doi: 10.1126/science.176.4039.1143
- Wang, Y., Li, Y., Wu, J., Pei, Y., Chen, X., & Hu, X. (2019a). Development of an immunochromatographic strip test for the rapid detection of soybean BowmanBirk inhibitor. Food and agricultural Immunology. Food and Agricultural Immunology, 30, 1202–1211. doi: 10.1080/09540105.2019.1680613
- Wang, Z., Wu, X., Liu, L., Xu, L., Kuang, H., & Xu, C. (2019b). An immunochromatographic strip sensor for sildenafil and its analogues. Journal of Materials Chemistry B, 7, 6383–6389. doi: 10.1039/c9tb00280d
- Zhang, Y., Geissen, S. U., & Gal, C. (2008). Carbamazepine and diclofenac: Removal in wastewater treatment plants and occurrence in water bodies. Chemosphere, 73, 1151–1161. doi: 10.1016/j.chemosphere.2008.07.086
- Ziylan, A., Koltypin, Y., Gedanken, A., & Ince, N. H. (2013). More on sonolytic and sonocatalytic decomposition of diclofenac using zero-valent iron. Ultrasonics Sonochemistry, 20, 580–586. doi: 10.1016/j.ultsonch.2012.05.005