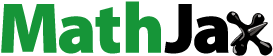
ABSTRACT
A simple, sensitive and specific chemiluminescence enzyme immunoassay based on biotin–streptavidin system (BASA-CLEIA) for diclazuril was established, which was about 2 and 4 times more sensitive than the traditional chemiluminescence enzyme immunoassay (CLEIA) and enzyme-linked immunosorbent assay. In the optimized generic assay, the half maximal inhibitory concentration (IC50) of the BASA-CLEIA for diclazuril was 0.48 μg/kg. The limit of detection for diclazuril was 0.02 μg/kg. This method showed no cross reactivity (<0.01%) with toltrazuril, robenidine hydrochloride, nicarbazin, halofuginone, amprolium, monensin, and maduramycin. In chicken muscles, the average recoveries ranged from 90.3% to 120.4%. A further comparison between the developed BSAS-CLEIA and high-performance liquid chromatography-tandem mass spectrometry (HPLC-MS/MS) method for real samples showed good correlation (p < 0.01). The results indicated that the simple, sensitive and specific BASA-CLEIA method could be used for the analysis of diclazuril in real samples.
1. Introduction
Coccidiosis is a contagious disease caused by single-celled parasites belonging to the genus eimeria, affecting numerous domestic animals, especially poultry and rabbits (Chapman, Citation2014; Shahraki, Shariati-Sharifi, Nabavi, & Jamshidian, Citation2018). The infection of coccidiosis associated with humid conditions and high-intensity rearing environment, may lead to substantial economic losses in animal husbandry. Diclazuril is widely and extensively used to prevent and treat coccidiosis as well as improve breeding efficiency (De Mil et al., Citation2017). However, illegal use and failed to follow the withdrawal period may result in the drug residues in animal edible tissues, which possess a potential health risk to consumers, in addition, the extensive use of these drugs can result in the development of resistance of the parasite to all available medications (Adenaike et al., Citation2018). Considering public safety, Codex Alimentarius Commission has set the maximum residue limits (MRLs) of diclazuril in poultry: 500 μg/kg in muscle, 2000 μg/kg in kidney and 3000 μg/kg in liver.
Numerous methods for the determination of diclazuril in feed, animal tissue and eggs have been reported, which included high-performance liquid chromatography with ultraviolet detection (Kock, Smet, & Sneyers, Citation1992), gas chromatography–mass spectrometry (Blanchflower, Hughes, & Kennedy, Citation1997), and liquid chromatography-tandem mass spectrometry (Barreto, Ribeiro, Hoff, & Costa, Citation2017; Mortier, Daeseleire, Huyghebaert, Grijspeerdt, & Peteghem, Citation2005a; Mortier, Daeseleire, Huyghebaert, Grijspeerdt, & Peteghem, Citation2005b). But diclazuril (4-chlorophenyl [2,6-dichloro-4-(4,5-dihydro-3H3, 5-dioxo-1, 2, 4-triazin-2-yl) phenyl] acetonitrile), is a non-polar compound, the determination of diclazuril using Hydrophilic Interaction Liquid Chromatography (HILIC) goes beyond the typical applications. Although the increased sensitivity and the short retention times have achieved using HILIC-MS/MS (Dasenaki & Thomaidis, Citation2019) and liquid chromatography-quadrupole linear ion trap-tandem mass spectrometry (HPLC-QTrap-MS/MS) (Barreto et al., Citation2017), these optimized methods are rather time-consuming, sample pretreatment-complicated and need expensive instruments, thus are not suitable for the screening of a large number of samples. Therefore, there is a great demand for developing simple, inexpensive, high throughput and reliable assay methods to monitor diclazuril in food products.
Immunoassays, which are rapid, sensitive, low-cost, and capable of high-throughput preferred for the monitoring of various drug residues. Immunoassays contain various assay forms such as enzyme-linked immunosorbent assay (ELISA), chemiluminescence immunoassays (CLEIA), radioimmunoassay (RIA) and so on. ELISA is a method based on the absorbance for analysing the target compound in samples. However, the sensitivity of ELISA is relatively low compared with CLEIA which is based on the chemiluminescence (Firouz et al., Citation2014; Gloria, Contri, Carluccio, & Robbe, Citation2018; Zamora, Hartung, Hildebrandt, & Käsbohrer, Citation2010). During these years, the CLEIA with good sensitivity, broad linear range and excellent stability has received more attention from clinical diagnose, environmental analysis and food safety. While, as far as we know, there were little studies about the CLEIA of diclazuril. Meanwhile, as the requirements become more and more strict, and actively respond to the great challenge to China agricultural products export trade brought by the implementation of Japan's “positive list system” and the European Union’s 2012 revised MRLs for anticoccidial drug residues in livestock and poultry products, we have to develop a more sensitive CLEIA for diclazuril as the technology reserve.
Avidin, a glycoprotein found in egg with molecular weight of 67 kDa, contains four binding sites with an extraordinary affinity (dissociation constant: about 10–15 M) for the small molecule vitamin, biotin (Kendall, Ionescu-Matiu, & Dreesman, Citation1983). Biotin could be easily covalent coupled to avidin by forming a solid binding, thus it has been widely used in immunoassay because of its high specificity and strong affinity (Chen et al., Citation2013; Chen et al., Citation2013; Pei, Jiming, Liu, Yi, & Zeng, Citation1999). The application of biotin–streptavidin system has been proved that it could further increase the sensitivity of traditional CLEIA mentioned above (Li-Bo et al., Citation2013; Ming et al., Citation2018; Zhang, Liu, & Goldys, Citation2018).
Hence, the aim of this study is to establish a sensitive BASA-CLEIA for the detection of the diclazuril in chicken muscles. After optimization and comparison, the BASA-CLEIA presented better sensitive than the traditional CLEIA and ELISA. We believe that the developed CLEIA and BASA-CLEIA has potential applications in the future.
2. Materials and methods
2.1. Apparatus
White opaque high binding plates and transparent 96-well microtiter ELISA plates were purchased from Costar (Cambridge, MA, USA). The solutions and buffers were prepared with water purified with a Milli-Q system (EMD Millipore Corporation, Belleria, MA, USA). ELISA result was measured by Sunrise microtiter plate reader (TECAN, Groedig, Austria) and CL-ELISA result was measured by Veritas Microplate Luminometer (Turner BioSystems, Sunny Vale, CA, USA).
2.2. Reagents
Super Signal ELISA chemiluminescence substrate solution was purchased from Thermofisher Scientific Pierce (Rockford II). The peroxidase-conjugated goat anti-mouse IgG (IgG-HRP) was obtained from Jackson Immuno-Research Laboratories, Inc. (West Grove, PA, USA). Biotin N-Hydroxysuccinimide Ester (NHSB) was purchased from Sigma (St. Louis, USA). The peroxidase-conjugated streptavidin (HRP-SA) was obtained from Beyotime Biotechnology, Inc. (Jiangsu, China). Diclazuril, toltrazuril, robenidine hydrochloride, nicarbazin, halofuginone, amprolium, monensin, and maduramycin were obtained from China Institute of Veterinary Drug Control (Beijing, China). All other chemicals and organic solvents were of reagent grade and acquired from Beijing Chemical Co. (Beijing, China). Diclazuril-oxime-ovalbumin and anti-diclazuril monoclonal antibody (MAb) were prepared previously in our laboratory (Wang et al., Citation2013).
2.3. Buffers
The following buffers were used: (1) coating buffer was 0.05 M carbonate buffer (CB), pH 9.6; (2) antibody and fusion protein dilution buffer were both 0.01 M phosphate-buffered saline (PBS) consisting of 137 mM NaCl, 2.7 mM KCl, 10 mM Na2HPO4 and 2 mM KH2PO4, pH 7.4; (3) blocking buffer was 0.01 M PBS containing 5% skim milk; (4) washing buffer was 0.01 M PBS containing 0.1% Tween 20.
2.4. Preparation of biotinylated-antibody
The NHSB was dissolved in dimethyl sulphoxide (DMSO) at a final concentration of 20 mg/mL. The anti-diclazuril MAb was diluted to 2 mg/mL with borate saline buffer (0.1 M, pH 8.8). Then the NHSB was added to antibody solution (NHSB: MAb = 50:1) and reacted at room temperature for 2 h. Biotinylated antibody was obtained after 3 times washing by ultrafiltration and diluted with 10 mM PBS and then stored in −20°C. The biotinylated antibody was identified with ultraviolet scanning analysis and ELISA analysis.
2.5. Optimization of conventional CLEIA and BASA-CLEIA
2.5.1. Procedure of conventional CLEIA and BASA-CLEIA
Opaque high binding plates were coated overnight at 4°C with 100 μL diclazuril-oxime-ovalbumin diluention. The plates were washed with 260 μL/well washing buffer manually three times and blocked with 150 μL/well blocking buffer, and the plates were incubated at 37°C for 1 h. After the plates were washed as described above, 50 μL/well of standard or sample solution, followed by 50 μL/well of MAb with proper dilution was added, respectively. The competitive reaction was allowed to take place for 30 min at 37°C. After washing five times, a 100 μL/well peroxidase-labeled goat anti-mouse immunoglobulins (1/5000 dilution) was added, and plates were incubated at 37°C for 30 min. After washing five times, the peroxidase activity was revealed by adding 100 μL/well of a freshly prepared substrate mixture of super signal substrate solution. The intensity of light units was measured at 425 nm using a chemiluminescence reader immediately after the addition of the substrate and the results were expressed in relative light units (RLU).
The whole procedure of BASA-CLEIA was similar with the CLEIA except for the replacement of MAb with biotinylated antibody and HRP-IgG with HRP-SA.
2.5.2. Optimization of conventional CLEIA and BASA-CLEIA
Several physicochemical factors influencing immunoassay performance were studied in CLEIA. Modifications of RLUmax and IC50 parameters of the standard curves were evaluated under different conditions. In order to assess the influence of coating buffer, blocking buffer, buffer ionic strength, pH, Tween-20, organic reagent, competitive time and temperature, the diclazuril standard diluent was prepared as follows: (1) coating buffer: 0.05 M, pH 9.6 CB; 0.01M, pH 7.4 phosphate buffer; 0.05 M, pH 5.0 citrate buffer. (2) blocking buffer: 5% Foetal bovine serum and 5% sucrose in 0.01 M PBS. (3) 0.05, 0.15, 0.30 and 0.50 mol/L NaCl in 0.01 M PBS. (4) pH 5.4, 6.4, 7.4 and 8.0 0.01 M PBS. (5) 1%, 2%, 4%, 8% and 10% acetonitrile in 0.01 M PBS. (6) 2%, 5%, 10%, 20% and 40% methanol in 0.01 M PBS. (7) 0%, 0.01%, 0.05% and 0.1% Tween 20 in 0.01 M PBS. (8) Competitive reaction time: 30, 45, 60 and 75 min at 37°C.
The conditions of BASA-CLEIA were optimized as follows: (1) coating conditions: 1, 2, 3 h at 37°C and overnight at 4°C; (2) diluent of HRP-SA: 0.01 M PBS, 1% gelatin in 0.01 M PBS, 0.5% BSA in 0.01 M PBS, 1% BSA in 0.01 M PBS, 5% calf serum in 0.01 M PBS, 10% calf serum in 0.01 M PBS and 20% calf serum in 0.01M PBS; (3) HRP-SA reaction time: 15, 30 and 45 min at 37°C. Other conditions were the same as CLEIA.
2.6. Specificity of CLEIA and BASA-CLEIA
The specificities of the CLEIA and BASA-CLEIA were assessed by evaluating the cross reactivities (CRs) studies with toltrazuril, robenidine hydrochloride, nicarbazin, halofuginone, amprolium, monensin, and maduramycin. CRs were calculated as follows:
2.7. Sample preparation
Diclazuril free samples were supplied by the National Reference Laboratory for Veterinary Drug Residues (Beijing, People’s Republic of China). Six samples were collected from retail outlets in Beijing. The samples were homogenized and sted at –20°C. And the limit of detection (LOD) was calculated using the following equation:
2.7.1. Samples preparation for immunoassay methods
The 2 g homogenized chicken muscle was weighed into a 50 mL polypropylene centrifuge tube. After the addition of 10 mL acetonitrile, the mixture was immediately vortex-mixed for 3 min and then sonicated for 10 min at ambient temperature. Subsequently, the suspension was centrifuged at 8000 rpm for 10 min at 4°C. Then, 1 mL supernatant was transferred to a clean tube and evaporated to dryness at 40°C under a stream of nitrogen. The residue was dissolved in 1 mL hexane with stirring for 30 s, followed by the addition of 1 mL sample dilution buffer and then stirred for 1 min. After centrifugation at 3500 rpm for 5 min, the lower layer was collected and diluted appropriately for analysis. These above spiked samples were also analysed by HPLC-MS/MS method (Sun et al., Citation2017).
2.8. Evaluation of the BASA-CLEIA
Diclazuril was added into the diclazuril free chicken muscle samples to yield 10, 20, 50 μg/kg, respectively. Each sample was measured five times in duplicate and in three consecutive days to assess the accuracy and precision. The real samples were analysed both by BASA-CLEIA and HPLC-MS/MS for the evaluation of the new method.
3. Results and discussion
3.1. Biotinylated-antibody conjugate and identification
The structure and activity of the antibody were characterized by UV scanning and ELISA reaction. As was shown in (A), the UV absorption spectra of biotinylated antibodies were significantly different from those of antibodies and NHS-activated biotin. The absorption peaks of protein at 280 nm changed in the polar environment after the reaction. ELISA reaction was used to evaluate the loss of activity before and after biotinylated of the antibody. As was shown in (B), the antibody activity was reduced by about 28% after biotinylated, but the OD 450 nm value remained around 1.0, which still met the requirement of the experiment.
3.2. Optimization of the CLEIA and BASA-CLEIA assay
There are many parameters that may affect the analytical performance and sensitivity of the assay. In this study, buffer types, ions strength, temperatures, component concentrations, dynamics and qualities of immune-components always brought significant influences on the antibody–antigen reaction rate (Dong et al., Citation2010; Sauer, Foulkes, & O'Neill, Citation1989). The optimal antigen coating concentration, antibody and biotinylated antibody dilution were determined by square matrix titration depending on the RLUmax/IC50 for the later experiment. Firstly, according to the results of optimization, the concentration of antibody and coating antigen for CLEIA were both 1:4000, as was shown in . Then, the later experiment was based on the optimized concentration of antibody and coating antigen. Three coating buffers such as CB (pH 9.6), phosphate buffer (pH 7.4) and citrate buffer (pH 6.6). According to the calculated RLUmax/IC50 ratio values, CB had the best sensitivity and thus was selected as the coating buffer in CLEIA and BASA-CLEIA. The blocking buffer also could affect the immunoassay, thus, 5% FBS + 5% sucrose/PBS, 5% skimmed milk powder/PBS, 0.5% gelatin + 25% sucrose/PBS, and 1%BSA/PBS were chosen as blocking buffer. The 5% FBS + 5% sucrose/PBS was the optimal blocking buffer.
Table 1. Optimization of coating antigen and antibody concentration of CLEIA.
To our knowledge, buffer ionic strength, pH, Tween-20, organic reagent could affect the binding of antigen and antibody. To evaluate the influence of these factors, standard analyte, antigen and antibody were diluted with different buffers. Immunoassays were carried out at an increasing PBS concentration ((A)); pH ((B)), acetonitrile concentrations ((C)), methanol concentrations ((D)) and increasing Tween-20 concentrations ((E)). The optimal conditions of CLEIA were at pH 8.0, ionic strength 0.15 mol/L and reaction time 60 min ((F)). The result indicated that the content of organic solvent in this experiment had a great influence on the sensitivity of chemiluminescent enzyme immunoassay, especially methanol, which seriously inhibited the immunoassay. The results showed that Tween-20 could improve the sensitivity of the method at the appropriate content of 0.01%.
Figure 2. Effect of different parameters on CLEIA performance. (A): salt concentrations; (B): pH values; (C): methanol concentrations; (D): acetonitrile concentrations; (E): Tween-20 concentrations; (F): Competition time.
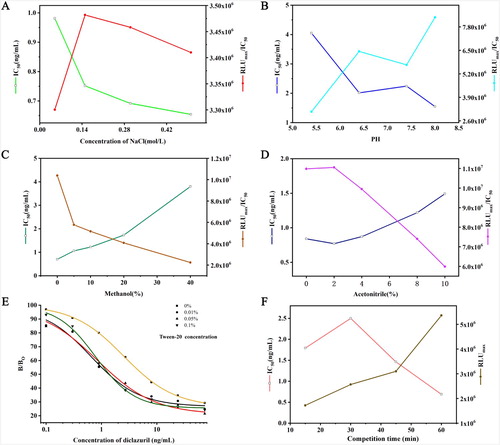
The concentration of biotinylated antibody and coating antigen for BASA-CLEIA were 1:8000 and 1:1000, respectively (). BASA-CLEIA with different coating conditions had no significant difference. The coating conditions were finally determined to be 4°C overnight ((A)). Excessive biotinylated antibodies, enzyme-labeled streptavidin and inappropriate diluents can cause an increase in non-specific adsorption, and resulting in a decrease in reaction sensitivity. To evaluate the influence of dilution buffer, 20% BSA/ PBS was selected as the best dilution buffer ((B)). The series of optimal working concentration of HRP-streptavidin and biotinylated antibody was shown in . When the dilution ratio of HRP-streptavidin and biotinylated antibody were 1:5000 and 1:8000, the corresponding RLUmax/IC50 value was the largest ((C)) and the optimal HRP-SA incubation time was 15 min at 37°C ((D)).
Figure 3. Effect of different parameters on BASA-CLEIA performance. (A): coating conditions; (B): HRP-labeled streptavidin dilution buffers; (C): HRP-labeled streptavidin concentrations; (D): HRP-labeled streptavidin incubation time.
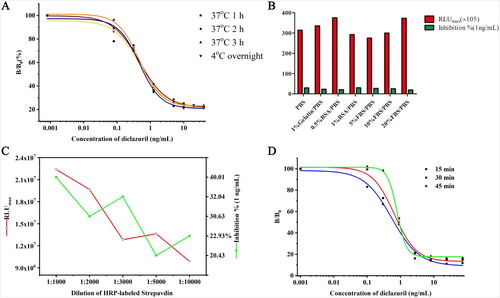
Table 2. Optimization of coating antigen and biotinylated antibody concentration for BSAS-CLEIA.
3.3. Validation of the BASA-CLEIA method
3.3.1. Sensitivity
The IC50 values of conventional CLEIA and BASA-CLEIA were about 0.99 and 0.48 ng/mL ((A,B)), respectively. In view of IC50, the sensitivity of BASA-CLEIA was improved 2-folds comparing with conventional CLEIA and 4-folds comparing with conventional ELISA (Wang et al., Citation2013).
The CLEIA is similar to the conventional ELISA, with the specific reaction of anti-diclazuril primary antibody and HRP-labeled goat anti-mouse secondary antibody, with catalysed luminol chemiluminescence system instead of TMB absorbance in ELISA. BASA-CLEIA also catalysed the chemiluminescence of the luminol, but improved the sensitivity by the use of biotin–streptavidin system. A schematic representation of the CLEIA and BASA-CLEIA of diclazuril was shown in . In this study, the immunological activity of the biotinylated anti-diclazuril antibody might have some loss in the preparation process, but it still met the requirements of experiment. In view of the higher isoelectric point value of avidin, between 10 and 10.5, the neutral environment of immunoassay might lead to the non-specific adsorption, and high background interference (Haun & Wasi, Citation1990). The isoelectric point of streptavidin is close to neutral, the surface band has less positive charge, the molecular weight of streptavidin is small, and also does not contain any glycosyl group, so the electrostatic adsorption with the polyelectrolyte microplate is weak and the background signal is little (Olafsen et al., Citation2004).
3.3.2. Specificity
Cross-reactivity (CR) is an important parameter to evaluate the specificity in immunoassay. The selectivity of CLEIA and BASA-CLEIA were evaluated by measuring the cross-reactivities (CRs) with other anticoccidial drugs, including toltrazuril, robenidine hydrochloride, amprolium hydrochloride, halofuginone hydrobromide, nicarbazin, monensin, and maduramycin. It showed negligible cross reaction with the other anticoccidial drugs (CR < 0.1%). These results indicated that the optimized CLEIA and BASA-CLEIA had a high selectivity, and might be applied for determination of diclazuril.
The combination of the specificity and sensitivity in the detection method could lead to the multi-stage amplification of biological signals (Meyer, Sauerwein, & Mutayoba, Citation1990; Sai et al., Citation2010). Streptavidin–biotin system also used to combine with materials like nanoparticles in flow immunoassay (Serebrennikova, Samsonova, & Osipov, Citation2018), immunomagnetic beads (Ming et al., Citation2018; Shan et al., Citation2014) and sandwich immunosensor (Zhang et al., Citation2018) to improve the specificity and sensitivity of the detection methods.
3.3.3. Recovery
The recovery test was carried out by fortifying known negative chicken muscle samples in five replicates with diclazuril at levels of 10, 20 and 50 μg/kg, respectively. This was repeated in three replicates on three days to examine the accuracy and precision of the method. Mean CRs of diclazuril from chicken muscle ranged from 90.3% to 120.4%, with the intra-day coefficients of variation of 2.1–9.5% and inter-day coefficients of variation of 5.4–16.3%. Mean recoveries of diclazuril from chicken muscle were in a range from 77.6% to 93.1% with the intra-day CVs of 3.7–21.0% and inter-day CVs of 5.4–16.3%. Therefore, the results suggested that BASA-CLEIA performed higher precision ().
3.4. Assay performance in chicken muscle
To assess the applicability and validity of the BASA-CLEIA, the HPLC-MS/MS was employed to detect diclazuril in chicken samples (n = 6) purchased from supermarkets in Beijing. From the results shown in , it was observed that determination of diclazuril measured by two methods had no significant difference and had a good correspondence (p < 0.1). It was demonstrated that the BASA-CLEIA could be used for the determination of diclazuril in real samples.
Numerous methods for the determination of diclazuril in feed, animal tissues and eggs have been reported. HPLC-MS/MS was found to be a fast, reliable, and practical method (Gong, Sun, Dong, Yu, & Wang, Citation2011; Jeong et al., Citation2017) with good sensitivity and specificity (Gong et al., Citation2011). The detection limits of diclazuril were 0.005 mg/kg and mean recoveries (n = 5) of diclazuril in spiked chicken tissues were 92.0–102.0% (CV 5.9–12.2%) (Shi, Zhu, Yun-Zhan, Ke-Zong, & Peng, Citation2009). LOD of diclazuril in real samples was 1.2 μg/kg in HPLC-MS/MS coupled to an electrospray ionization tandem mass spectrometer operating in the negative ion mode (Ai, Sun, Wang, Chen, & Guo, Citation2011). HPLC-MS/MS methods are rather time-consuming and require complicated sample preparation and expensive instruments. Thus, we took this method for the confirmation of our new developed method. The results of the established BASA-CLEIA not only provides a theoretical basis for quantitative detecting the residues of agricultural and veterinary drugs and other chemical pollutants in animal food (Zhang et al., Citation2018), but also protects the consumer safety and effectively controls the residues of diclazuril in animal-derived food ( and ).
Table 3. Recoveries and CVs of spiked chicken muscle samples.
Table 4. The confirmation of the BASA-CLEIA with HPLC-MS/MS.
4. Conclusion
In summary, we successfully established the CLEIA and BASA-CLEIA with specificity and high sensitivity for diclazuril. It is first reported of BASA-CLEIA detecting the diclazuril in food safety field. The IC50 value of BASA-CLEIA was improved 2 folds compared with conventional CLEIA and 4 folds compared with conventional ELISA. The mean recoveries of diclazuril from chicken muscle ranged from 90.3% to 120.4%. It is indicated that the BASA-CLEIA is a reliable analytical method and could be widely used in chicken tissue analysis.
Acknowledgements
The authors would like to thank all the people who provided valuable advice and technical support.
Disclosure statement
No potential conflict of interest was reported by the authors.
Additional information
Funding
References
- Adenaike, A. S., Peters, S. O., Adeleke, M. A., Fafiolu, A. O., Takeet, M. I., & Ikeobi, C. O. N. (2018). Use of discriminant analysis for the evaluation of coccidiosis resistance parameters in chickens raised in hot humid tropical environment. Tropical Animal Health & Production, 50(5), 1161–1166. doi: 10.1007/s11250-018-1547-1
- Ai, L., Sun, H., Wang, F., Chen, R., & Guo, C. (2011). Determination of diclazuril, toltrazuril and its two metabolites in poultry tissues and eggs by gel permeation chromatography–liquid chromatography–tandem mass spectrometry. Journal of Chromatography B, 879(20), 1757–1763. doi: 10.1016/j.jchromb.2011.04.021
- Barreto, F., Ribeiro, C., Hoff, R. B., & Costa, T. D. (2017). A simple and high-throughput method for determination and confirmation of 14 coccidiostats in poultry muscle and eggs using liquid chromatography – quadrupole linear ion trap – tandem mass spectrometry (HPLC–QqLIT-MS/MS): validation according to Europe. Talanta, 168, 43–51. doi: 10.1016/j.talanta.2017.02.007
- Blanchflower, W. J., Hughes, P. J., & Kennedy, D. G. (1997). Determination of nicarbazin in eggs by liquid chromatography-atmospheric pressure chemical ionization mass spectrometry. Journal of AOAC International, 80(6), 1177–1182. doi: 10.1093/jaoac/80.6.1177
- Chapman, H. D. (2014). Milestones in avian coccidiosis research: A review. Poultry Science, 93(3), 501–511. doi: 10.3382/ps.2013-03634
- Chen, Y. P., Zou, M. Q., Qi, C., Xie, M.-X., Wang, D.-N., Wang, Y.-F., … Chen, Y. (2013). Immunosensor based on magnetic relaxation switch and biotin-streptavidin system for the detection of Kanamycin in milk. Biosensors & Bioelectronics, 39(1), 112–117. doi: 10.1016/j.bios.2012.06.056
- Dasenaki, M. E., & Thomaidis, N. S. (2019). Multi-residue methodology for the determination of 16 coccidiostats in animal tissues and eggs by hydrophilic interaction liquid chromatography-tandem mass spectrometry. Food Chemistry, 275(1), 668–680. doi: 10.1016/j.foodchem.2018.09.138
- De Mil, T., Devreese, M., Maes, A., De Saeger, S., De Backer, P., & Croubels, S. (2017). Influence of mycotoxin binders on the oral bioavailability of tylosin, doxycycline, diclazuril, and salinomycin in fed broiler chickens. Poultry Science, 96(7), 2137–2144. doi: 10.3382/ps/pew503
- Dong, T., Sun, J., Liu, B., Zhang, Y., Song, Y., & Wang, S. (2010). Development of a sensitivity-improved immunoassay for the determination of carbaryl in food samples. Journal of the Science of Food & Agriculture, 90(7), 1106–1112. doi: 10.1002/jsfa.3917
- Firouz, Z. E., Kaboosi, H., Nasiri, A. F., Tabatabaie, S. S., Golhasanikeshtan, F., & Zaboli, F. (2014). A comparative serological study of toxoplasmosis in pregnant women by CLIA and ELISA methods in Chalus City Iran. Vocational Guidance Quarterly, 16(4), e15115.
- Gloria, A., Contri, A., Carluccio, A., & Robbe, D. (2018). Blood periovulatory progesterone quantification using different techniques in the dog. Animal Reproduction Science, 192, 179–184. doi: 10.1016/j.anireprosci.2018.03.006
- Gong, X., Sun, J., Dong, J., Yu, J., & Wang, H. (2011). Determination of avermectin, diclazuril, toltrazuril and metabolite residues in pork by high performance liquid chromatography-tandem mass spectrometry. Chinese Journal of Chromatography, 29(3), 217–222. doi: 10.3724/SP.J.1123.2011.00217
- Haun, M., & Wasi, S. (1990). Biotinylated antibodies bound to streptavidin beads: A versatile solid matrix for immunoassays. Analytical Biochemistry, 191(2), 337–342. doi: 10.1016/0003-2697(90)90228-2
- Jeong, K., Jeong, M., Park, H., Hossain, M. A., Kim, D., Lee, K., & Kang, J. (2017). Development of high-performance liquid chromatography methods for the anticoccidials: Toltrazuril and diclazuril. Korean Journal of Veterinary Research, 57(4), 223–226.
- Kendall, C., Ionescu-Matiu, I., & Dreesman, G. R. (1983). Utilization of the biotin/avidin system to amplify the sensitivity of the enzyme-linked immunosorbent assay (ELISA). Journal of Immunological Methods, 56(3), 329–339. doi: 10.1016/S0022-1759(83)80022-2
- Kock, J. D., Smet, M. D., & Sneyers, R. (1992). Determination of diclazuril in animal feed by liquid chromatography. Journal of Chromatography A, 606(1), 141–146. doi: 10.1016/0021-9673(92)85268-X
- Li-Bo, L. I., Liu, Y. B., Guan, G. Y., Yao, Y., Liu, Z. R., Hou, H. R., & Liang, R. P. (2013). Chemiluminescence immunoassay for thyroid stimulating hormone by using biotin-avidin system. Labeled Immunoassays & Clinical Medicine, 20(1), 31–35.
- Meyer, H. H., Sauerwein, H., & Mutayoba, B. M. (1990). Immunoaffinity chromatography and a biotin-streptavidin amplified enzymeimmunoassay for sensitive and specific estimation of estradiol-17 beta. Journal of Steroid Biochemistry, 35(2), 263–269. doi: 10.1016/0022-4731(90)90283-X
- Ming, L., Zhang, Y., Zhao, R., Liu, Z., Xia, H., Yin, C., & Du, D. (2018). Immunomagnetic bead-based biotin-streptavidin system for highly efficient detection of aflatoxin B1 in agricultural products. RSC Advances, 8, 26029–26035. doi: 10.1039/C8RA04460K
- Mortier, L., Daeseleire, E., Huyghebaert, G., Grijspeerdt, K., & Peteghem, C. V. (2005a). Detection of residues of the anticoccidial diclazuril in poultry tissues by LC-MS/MS after withdrawal of medicated feed. Abstracts Book of the “17th European Symposium on the Quality of Poultry Meat” and the 11th European Symposium on the Quality of Eggs and Egg Products, pp. 192–193.
- Mortier, L., Daeseleire, E., Huyghebaert, G., Grijspeerdt, K., & Peteghem, C. V. (2005b). Detection of residues of the coccidiostat diclazuril in poultry tissues by liquid chromatography-tandem mass spectrometry after withdrawal of medicated feed. Journal of Agricultural & Food Chemistry, 53(4), 905–911. doi: 10.1021/jf048468z
- Olafsen, T., Tan, G. J., Cheung, C.-w., Yazaki, P. J., Park, J. M., Shively, J. E., … Wu, A. M. (2004). Characterization of engineered anti-p185HER-2 (scFv-CH3)2 antibody fragments (minibodies) for tumor targeting. Protein Engineering Design & Selection, 17(4), 315–323. doi: 10.1093/protein/gzh040
- Pei, R. J., Jiming, H. U., Liu, L. J., Yi, H. U., & Zeng, Y. (1999). Mass amplification of biotinavidin system in piezoelectric immunosensor. Chemical Research in Chinese Universities, 20(6), 879–880.
- Sai, N., Chen, Y., Liu, N., Yu, G., Su, P., Feng, Y., … Gao, Z. (2010). A sensitive immunoassay based on direct hapten coated format and biotin-streptavidin system for the detection of chloramphenicol. Talanta, 82(4), 1113–1121. doi: 10.1016/j.talanta.2010.06.018
- Sauer, M. J., Foulkes, J. A., & O'Neill, P. M. (1989). The influence of heterology, enzyme label and assay conditions on the sensitivity of microtitre plate enzymeimmunoassays for progesterone in milk. Journal of Steroid Biochemistry, 33(3), 433–438. doi: 10.1016/0022-4731(89)90334-8
- Serebrennikova, K. V., Samsonova, J. V., & Osipov, A. P. (2018). Enhancement of the sensitivity of a lateral flow immunoassay by using the biotin–streptavidin system. Moscow University Chemistry Bulletin, 73(3), 131–134. doi: 10.3103/S0027131418030070
- Shahraki, F., Shariati-Sharifi, F., Nabavi, R., & Jamshidian, A. (2018). Coccidiosis in Sistan: The prevalence of Eimeria species in native chicken and its histopathological changes. Comparative Clinical Pathology, 27(4), 1–7.
- Shan, S., Zhong, Z., Lai, W., Xiong, Y., Cui, X., & Liu, D. (2014). Immunomagnetic nanobeads based on a streptavidin-biotin system for the highly efficient and specific separation of Listeria monocytogenes. Food Control, 45(45), 138–142. doi: 10.1016/j.foodcont.2014.04.036
- Shi, Z. H., Zhu, L. Q., Yun-Zhan, L. U., Ke-Zong, Q. I., & Peng, K. S. (2009). Simultaneous determination of residues of diclazuril and toltrazuril in chicken tissues by high performance liquid chromatography. Chinese Journal of Veterinary Science, 23(1), 73–84.
- Sun, F., Yang, S., Zhang, H., Zhou, J., Li, Y., Zhang, J., & Shen, J. (2017). Comprehensive analysis of tiamulin metabolites in various species of farm animals using ultra-high-performance liquid chromatography coupled to quadrupole/time-of-flight. Journal of Agricultural and Food Chemistry, 65(1), 199–207. doi: 10.1021/acs.jafc.6b04377
- Wang, Y., Wang, Z., Jiang, H., Xi, X., Shen, J., & Ding, S. (2013). Development of a monoclonal antibody-based enzyme-linked immunosorbent assay for the analysis of diclazuril in chicken tissues. Food Analytical Methods, 6(6), 1685–1692. doi: 10.1007/s12161-013-9582-z
- Zamora, B. M., Hartung, M., Hildebrandt, G., & Käsbohrer, A. (2010). Detection of antibodies to S. Enteritidis in broilers by means of Indirect ELISA and chemiluminescent immunoassay (CLIA). Zentralblatt Für Veterinärmedizin.Reihe B. Journal of Veterinary Medicine, 46(1), 9–24.
- Zhang, K., Liu, G., & Goldys, E. M. (2018). Robust immunosensing system based on biotin-streptavidin coupling for spatially localized femtogram mL−1 level detection of interleukin-6. Biosensors & Bioelectronics, 102(4), 80–86. doi: 10.1016/j.bios.2017.11.023