ABSTRACT
The development of highly selective analytical methodologies to monitoring phthalates (phthalic acid esters, PAEs) is important for the safety of environment, food and equipment. As one of the most important and classical interactions in biological systems, antigen–antibody interactions constitute a highly specific chemical nature in biological systems, which inspire us to develop highly specific, highly selective and high-throughput immunoassays for monitoring PAEs in different fields. We here attempt to give an introduction of the recent development particularly focusing on the immunoassay based on the specific binding of antigen–antibody for detecting PAEs. We also present an overview of the potential applications of the antibody labelling strategies to immunoassays for PAE investigations. Finally, we present the major challenges and opportunities regarding the high selectivity and specificity of PAE analysis based on the antigen–antibody interaction. We believe that this review provides critical insights into highly selective PAE analysis and gives an understanding of immunoassays.
1. Introduction
Since the 1920s, phthalates or phthalic acid esters (PAEs) have been used as artificial chemicals, which were widely used in the form of plasticizers for the synthesis and processing of plastics (Net, Sempere, Delmont, Paluselli, & Ouddane, Citation2015). Plastic products are still indispensable in human life (Guo & Kannan, Citation2013). Because PAEs could change the flexibility of polyvinyl chloride to achieve the diversification of plastic products (Li, Panchal, Mafi, & Xi, Citation2018), PAEs were widely used in real life (Shi, Cao, Zhang, & Zhao, Citation2018). However, several studies have shown that the existing form between PAEs and PVP was not combined by chemical bonds (Benning, Liu, Tiwari, Little, & Marr, Citation2013; Xu, Xiong, Zhao, Xiang, & Wu, Citation2019). Overtime the plasticizer will detach from the plastic products (Gartner, Balski, Koch, & Nehls, Citation2009; Schettler, Citation2006) and spread widely, including plastic-based containers, ships, packaging materials and other plastic containers, such as clothing, sports equipment, toothbrush and other daily necessities (Gong, Weschler, & Zhang, Citation2016; Guo & Kannan, Citation2013; O'Connell, Kincl, & Anderson, Citation2014). Then it caused pollution to the environment (Net et al., Citation2015; Qiao, Wang, Yan, & Yang, Citation2014). It also harm human health (Katsikantami et al., Citation2016) and even affect fertility (Gao et al., Citation2018; Maitre et al., Citation2018) and hormone secretion (You et al., Citation2015), especially the incident of phthalate-contaminated foodstuffs happened in Taiwan between April and July, 2011 (Hsiao et al., Citation2011). A recent research reveals that plastic teabags release billions of microparticles and nanoparticles into tea, its dose-dependent behavioural and developmental effects were revealed through an invertebrate toxicity assessment (Hernandez et al., Citation2019). Therefore, the development of new methodologies and technologies like the detection of PAEs is of great significance to human health, the environment and food safety.
Thus, it is necessary to develop novel quantitative technology with a high selectivity and specificity towards PAEs. To date, only instrumental analysis method has been most popular in developing strategies for the quantitative analysis of PAEs in samples. This strategy uses the physiochemical properties of PAEs in gradient eluent to conduct a qualitative and quantitative analysis. These instrumental analyses include gas chromatography (Lin, Chen, Zhu, & Wang, Citation2015), high-performance liquid chromatography (Draviam, Kerkay, & Pearson, Citation2006), gas chromatography-mass spectrophotometry detection (Xu et al., Citation2014; Insuan et al., Citation2016) and liquid chromatography-mass spectrometry detection (Hsu, Peng, Li, Lin, & Liao, Citation2011; Vavrous et al., Citation2019). Even though several national standards stated by the instrument analysis that has practical difficulties and it necessitates professional, exquisite operator and complex sample processing steps are essential in performing the analysis. In addition, by a combination of favourable optical properties, separation characteristics and surface modification, the detection systems based on magnetic material, noble metal nanoparticle and aptamers have been designed and successfully applied for the determination of PAEs (Han et al., Citation2017; Yan et al., Citation2018; Yang, Liu, Yan, Liu, & Zheng, Citation2016). Apparently, these strategies have an advantage over conventional design principles of analysis methods; nonetheless, their reproducibility, universality and the stability of the sensor itself are difficult to guarantee. The performance of the novel nanomaterial-based sensor, their characteristics and biodegradability might cause secondary potential safety issues (Wang et al., Citation2019), resulting from size, charge, morphology, chemical composition of nanoparticles and causing it to be hidden in an environmental matrix or biological environmental matrices. Therefore, it is imperative to develop some simple, cheap, accurate, sensitive and specific strategies to detect PAE in samples.
Antigen–antibody interaction is the biochemical essence inherent in the high specificity in life activities, where antibody biological macromolecules (i.e. polyclonal antibody and monoclonal antibodies) play essential roles in many biological activities mainly by binding antigenic determinants (Cooper, Citation2015). Inspired by this idea, the immunoassays have been greatly developed, which are based on the highly specific molecular recognition of antibodies and antigens to achieve the qualitative and quantitative analysis for PAEs. Furthermore, the key to developing an immunoassay for target analyte compounds is to produce an antibody with high affinity and high selectivity. Besides, analytes that do not stimulate the animal to produce directly specific antibodies, often require the introduction of a modifiable functional group and the attachment of the tether to the carrier protein (Chen et al., Citation2015; Tochi et al., Citation2016a). depicts the concept of immunogen and coated antigen design of phthalates. This review summarizes several examples of the immunoassay strategies to detect PAEs, which are demonstrated for studying human health, the environment and food safety. In view of these, we first discuss the selectivity and specificity of enzyme-linked immunoassays by enzyme-labelled antibodies. Then we describe the fluorescent immunoassay by fluorescent-labelled antibody strategies to demonstrate the selectivity and specificity of immunoassay. In addition, we have systematically reviewed the quantitative detection of PAEs in the environment and foodstuff by enzyme-linked immunoassay, biotin–streptavidin enzyme-linked immunoassay, fluorescence immunoassay, chemiluminescence immunoassay and time-resolved immunoassay (). Finally, we demonstrate the strategy to synthesize the probe antibody achieved by self-assembly between the label and the antibody.
Figure 1. The schematic diagram of the synthesis of phthalates antigens. Immunogens and coated antigens using different protein, BSA and OVA were selected.
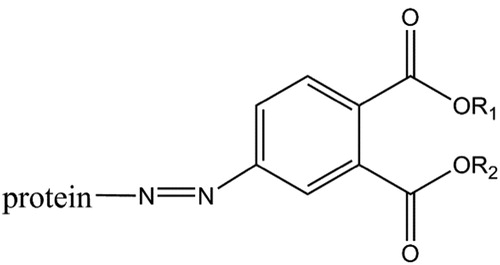
Figure 2. The several strategies of an immunoassay for the quantitative determination of PAEs mainly include enzyme-linked immunoassay (ELISA), biotin-streptavidin enzyme-linked immunoassay (BA-ELISA), fluorescence immunoassay (FIA), chemiluminescence immunoassay (CL-ELISA) and time-resolved immunoassay (TRFIA).
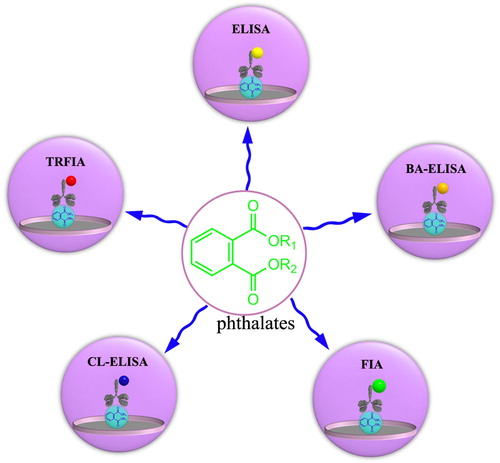
2. Improving the efficiency and specificity of the existing PAE detection strategies
To date, numerous analytical methods for detection of phthalate esters have been developed, and various detection methods have been developed in different samples such as plastic products, cosmetic products and drinking water (Tian et al., Citation2015; Vavrous et al., Citation2019; Yan et al., Citation2018). These existing analytical methods, including instrumental analysis, magnetic material sensor and fluorescent sensor, showed high sensitivity. However, the complexity of the sample pretreatment renders a great challenge to these methods with efficiency and specificity. This part discusses tactics to improve the efficiency and specificity of the enzyme (e.g. Horseradish peroxidase)-based labelled antibody for the detection of PAEs by immunoassays. The method for the construction of high efficiency and specificity immunoassay platform by using different kinds of enzyme-labelled antibodies (e.g. primary antibody and secondary antibody) was mainly discussed. The several strategies of an immunoassay for detecting PAEs are summarized in .
Table 1. Examples of immunoassays for the detection of PAEs.
2.1. Competitive enzyme-based immunoassays strategies for PAE detection
Enzymes, a kind of vital biocatalysts, play an important role in protein design and directed evolution (Zhang, Quin, & Schmidt-Dannert, Citation2018). Numerous biochemical reactions could be carried out efficiently due to the action of the enzyme, specifically under extremely mild conditions (Tran & Balkus, Citation2011). Among all the enzyme-based reactions, the enzyme-labelled protein has attracted much attention since it could form target-biocatalysts with specific functionalization through the form of chemical modification (Kai, Chen, Monterroso, & Zhou, Citation2015). We found that horseradish peroxidase is frequently employed to label antibody and form an enzyme-labelled antibody, which get the utmost out of the characteristics of antigen–antibody-specific reactions and the efficiency of enzyme catalysis (Xu et al., Citation2012), thereby achieving the high efficiency and specificity of the enzyme-based immunoassay for the detection of PAEs. Two kinds of enzyme-based immunoassay strategies (i.e. indirect competitive enzyme-based immunoassay and direct competitive enzyme-based immunoassay) are used for the detection of PAEs with efficiency and specificity, of which the use of horseradish peroxidase as the high biocatalyst antibody provides the high-specificity recognition site. This means generally realized by the specificity of antigen–antibody-specific binding and colour changes based on enzyme catalysed substrate, as first reported by Zhang group (Zhang et al., Citation2010; Zhang & Sheng, Citation2010). In this case, the specificity of the competitive enzyme-based immunoassay was also dominated through the type of antibody. The polyclonal antibody has been widely used as the medium for the specific recognition of an analyte; but, it cannot be simply used an enzyme-based immunoassay for the detection of PAEs because of its low purity. Therefore, it is necessary to control strictly the titre of polyclonal antibodies to prevent nonspecific adsorption and the appearance of false signals. The other strategy is to use monoclonal antibodies conjugated with an enzyme to guarantee the specificity in the competitive enzyme-based immunoassays, as reported by the Xu group (Tochi et al., Citation2016a, Citation2016b). But, the high cost and complex technology increased the difficulty in obtaining monoclonal antibodies. Despite this, both strategies have been demonstrated to be useful for PAE detection with efficiency and specificity.
2.1.1. Direct competitive enzyme-based immunoassay strategies for PAE detection
Usually, a direct competitive enzyme-based immunoassay strategy for PAE detection by direct conjugation between enzyme and antibody was designed to select the enzyme according to the corresponding characteristics of the substrate from the ELISA design principle. The Xu group has developed an enzyme-linked immunoassay for dibutyl phthalate (DBP) in liquor using a specific monoclonal antibody against DBP (Li et al., Citation2013). The Ren group has established a direct competitive immunoassay for the detection of mono-2-ethylhexyl phthalate in human urine (Feng et al., Citation2013). In addition, our group has reported several types of enzyme-linked immunoassay for detection of PAEs including di-(2-ethylhexyl) phthalate in infant supplies, dicyclohexyl phthalate in foods, dipropyl phthalate in plastic food contact materials, dimethyl phthalate (DMP) in water samples and diethyl phthalate (DEP) in spiking water samples (Zhang et al., Citation2010; Zhang, Hu, et al., Citation2013; Zhang, Wang, et al., Citation2013; Zhang et al., Citation2015; Zhang et al., Citation2011). These strategies enhance the efficiency and specificity of enzyme-based immunoassay. However, we have found direct competitive enzyme-based immunoassay strategies for PAE detection using a large amount of antibodies.
2.1.2. Indirect competitive enzyme-based immunoassay strategies for PAE detection
Based on the problems mentioned above, we have developed an indirect competitive enzyme-based immunoassay strategy for PAE detection. Thus, we have summed up several indirect competitive enzyme-based immunoassays for PAE detection by conjugating enzyme with the secondary antibody to combine the primary antibody (Kuang et al., Citation2010, Citation2013; Wei et al., Citation2011). This strategy effectively economizes the amount of the primary antibody and decreases non-specific adsorption and strengthens the sensitivity of the immunoassay (Li et al., Citation2019; Tang et al., Citation2015). Our previous work has developed several indirect competitive enzyme-based immunoassay for detection of DMP in environmental water samples (Zhang, Liu, et al., Citation2012; Zhang, Yu, et al., Citation2012). All these excellent properties of the indirect competitive enzyme-based immunoassay made them particularly attractive for environmental water sample applications. Thereafter, indirect competitive enzyme-based immunoassay for detection of PAEs has received a wide range of applications as reported by another group.
2.2. Biotin–streptavidin enzyme-based immunoassay strategies for PAE detection
In the late 1970s, a new type of bioreactor amplification system has developed based on biotin–avidin-system, which has become a new technology widely used in the quantitative detection of trace analytes (Li et al., Citation2017). On the basis of above, the biotin–streptavidin system has introduced an enzyme-based immunoassay to amplify reaction signal because the biotin–streptavidin enzyme-linked immunoassays (BA-ELISA) has demonstrated that eight-folds higher sensitivity than the traditional ELISA under the same antibody and coating antigen (Wang, Zhang, Gao, Duan, & Wang, Citation2010). This strategy has been reported by the Zhuang group, including the biotin–streptavidin enzyme-based immunoassay for the determination of PAEs mainly in beverages and drinking water, milk and milk products and wine samples (Sun & Zhuang, Citation2014, Citation2015a, Citation2015b, Citation2015c). These studies provide an effective strategy for the determination of PAEs via the biotin–avidin amplification system.
3. Improving the sensitivity and selectivity of the existing PAE detection strategies
Although the use of the enzyme catalysis and biotin–avidin amplification system is an excellent way to construct the highly effective immunoassay platform, the low signal value for some substrate molecules essentially limits the sensitive determination for PAEs in human health, the environment and food safety analysis. Rational selection of the signal probe essentially gives a good solution to constructing a highly sensitive immunoassay platform (Xiong et al., Citation2018; Yang et al., Citation2018). In this part, we demonstrate several examples of fluorescent probe-labelled polyclonal antibody and further construct the highly sensitive immunoassay platform for PAE analysis. The strategy demonstrated here could be applied for other fluorescent probe-labelled antibodies and may open up a new method in the design of the highly sensitive immunoassay platform for PAE analysis.
3.1. Fluorescent-linked immunoassay strategies for PAE detection
Inspired by the high efficiency and specificity of enzyme-based immunoassay strategies, we proposed a novel immunoassay strategy to enhance the sensitivity of immunoassay using new fluorescein derivatives (i.e. fluorescein isothiocyanate, rhodamine isothiocyanate). These fluorescein derivatives could be bound to antibodies spontaneously without any coupling agent in mild reaction conditions (Wei, Hsu, Lee, Lin, & Huang, Citation2012). The fluorescein isothiocyanate (FITC) is one of the most popular fluorescent probes widely applied in protein labelling (Wu, Shanmugam, Tseng, Hiseh, & Tseng, Citation2016). Thus, a novel competitive fluorescence immunoassay for the determination of DBP in environmental samples using a polyclonal antibody, as reported by the Zhuang group and our group (Zhang et al., Citation2006, Citation2007, Citation2010, Citation2011). The fluorescence immunoassay platform sensitively determinates PAEs after fluorescent probe-labelled antibody specificity combined with coated antigen (Cui et al., Citation2015). Furthermore, we also systematically studied the two strategies: the sensitivity and accuracy of the enzyme-linked immunoassay and fluorescence immunoassay using the same antibody and coating antigen and found significant differences between them. In this study, we found that the strategy of FITC-immunoassay significantly improves the sensitivity for PAE analysis, unfortunately, it possesses a high background signal.
3.2. Chemiluminescent enzyme immunoassay strategies for PAEs
The chemiluminescence-based enzyme immunoassay (CL-ELISA) improves the sensitivity of the experiment to a certain extent by enhancing the intensity of the signal value (Yao et al., Citation2019). The CL-ELISA resembles ELISA, in its basic principle except that the luminescent properties produced by the enzyme catalysing the substrate are different. CL-ELISA is by definition the chemiluminescence intensity produced by a chemical reaction is directly proportional to the amount of analyte present in a sample (Zeng et al., Citation2016). Once the chemiluminescence enhancer is introduced into the peroxidase-luminol-H2O2 system that drives the chemiluminescence and light is produced (Chen et al., Citation2017). Thus, this approach increases the sensitivity of immunoassays based on a low background. Based on the above, we believe that chemiluminescence strategy would effectively overcome the above weakness. This idea was initially inspired by the properties of chemiluminescence; luminol catalysed to the ground state by HRP-labelled antibody and then an enhancer in oxidant condition was accompanied by a similar fluorescence emission (Zhu et al., Citation2015). More importantly, the chemiluminescence immunoassay strategies showed significantly higher sensitivity, as demonstrated in our previous work (Zhang, Hu, et al., Citation2012). This work provides a highly sensitive strategy for detecting PAEs in foodstuffs.
3.3. Time-resolved fluorescence immunoassay strategies for PAEs
Time-resolved fluorescence immunoassay (TRFIA) is a special kind of fluorescence analysis that uses a lanthanide-labelled antigen or antibody to measure fluorescence (Deng et al., Citation2016). This strategy could effectively eliminate the interference of non-specific fluorescence and greatly improve the sensitivity of analysis due to the luminescence properties of the lanthanide chelates. A non-radioactive trace analysis method is formed based on the combination of time-resolved fluorescence and specific immune response. This strategy is applied to environmental monitoring of esters. Sun et al. developed a Eu3+ labelling TRFIA for analysing food samples (Zhu et al., Citation2015). Du et al. developed a sensitive direct competitive TRFIA for the quantitative determination of trace DEP in environmental waters. Furthermore, a dual-labelled TRFIA for the sensitive detection of DEP and DBP in aquatic environments using europium (Eu3+) and samarium (Sm3+) as fluorescent labels (Zhu et al., Citation2017, Citation2019). The most commonly used rare earth metals are mainly Eu and Tb. Although Eu has a long fluorescence lifetime, its instability in water requires the addition of an enhancer. On the contrary, Tb has good stability, but its short fluorescence wavelength, severe scattering and easy decomposition limit its application. Therefore, the selection of the fluorescent probe in the fluorescent immunoassay for PAE detection requires not only a fluorescent probe having high photobleaching resistance but also a green, stable and high luminous intensity.
4. Self-assembly strategies for labelling antibody preparation
In addition to the attention paid to the development of immunoassay based on the signal probe engineering of the polyclonal antibody, we were also interested in the extension of the strategies demonstrated above to prepare new materials with emerging antibody labelling strategies based on another probe. We have prepared a new kind of carbon dot fluorescent probe that was formed from glutaraldehyde and carbon dots very recently, which first usually form carbon nanoclusters and self-assemble to form carbon nanocrystals, nanospheres or nanofibres in different ionic strength solutions at room temperature. The aldehyde functionalized polymorphic carbon dot fluorescent probe can covalently bond easily with amino groups on proteins (i.e. antibody). The formed polymorphic carbon dot fluorescent probes not only show good stability and unique optical properties that are originated from the precursors of the carbon dots but also exhibit good water-solubility, photostability and widely applied in the biomedical field easily due to the connecting proteins (Li, Lian, Yan, Xia, & Zhang, Citation2018). These unique properties essentially made the materials particularly attractive for electronic equipment, labelled probes and imaging reagent development (Lim, Shen, & Gao, Citation2015) for example, by the self-assembly of carbon dot for applications in direct imaging and biosensing (Jiang et al., Citation2015), disease diagnosis, print ink, photocatalysis and photovoltaic devices (Sun, Zhang, Jiang, Wu, & Lin, Citation2016; Wang, Chen, & Zhou, Citation2018). The evaporation-induced carbon dot self-assembly gives rise to different morphology fluorescent probes under different ionic strength conditions. Crucially, these different morphology fluorescent probes can further bind to the amino groups on the protein under approximately neutral conditions. This goes especially well with antibody labelling and bioanalytical conditions. This makes carbon dot to a powerful fluorescent labelling tool in the bioanalysis field. Based on this, we also developed a carbon dot-based immunoassay to demonstrate the utility of the fluorescent probe, where the experiment group fluorescence intensity is about 10 times that of the blank control group (Li, Lian, et al., Citation2018). By contrast, when only carbon dots were examined, no significant fluorescence signal was observed, indicating good specificity and selectivity of the proposed fluorescent immunoassay. We believe that this kind of new materials would be very useful for the development of fluorescent immunoassay, by this strategy integrating excellent protein labelling probe and further improving the sensitivity and selectivity of fluorescent immunoassay.
5. Conclusions and prospects
The development of high efficiency and specificity immunoassays for the sample analysis has become a hot topic between food chemistry and environmental science because of its excellent properties in understanding the immunoassay for the determination of PAEs. The development over several years ago has demonstrated that changing the type of the signal probe could develop high efficiency and specificity immunoassay for the determination of PAEs in food chemistry and environmental science applications. Based on this immunoassay protocol, numerous novel immunoassays were developed for PAE analysis in samples by selecting different signal probes. Generally, in the immunoassay protocol, high background for plate is always accompanied by the entire measurement process. Therefore, how to rationally design fluorescently labelled probes in these processes becomes the key point to achieve high efficiency and specificity for the determination of PAEs. To realize the high selectivity, efficiency and specificity for the determination of PAEs, the strategies for labelling antibodies should be rationally designed and fluorescent probes with excellent performance should be selected. On this basis, this review also provides insights into the labelled highly active biomolecules (i.e. antibody). We investigated the importance of self-assembly labelled proteins strategy in immunoassay. At present, the self-assembly labelled antibodies/antigens strategy is the most widely used method for the labelling of antibodies/antigens due to the complete reservation of binding sites for antibodies/antigens. Compared to covalent conjugation, the coupling agent molecules often lack the ability of specifically binding the proteins, which lead to the loss of covered active-binding sites. Thus, the way to conjugation labelling has a huge impact on the affinity of antibodies and is essentially the most critical step in the whole immunoassay. Although the review in this paper is not comprehensive, we have demonstrated that the strategies and future trends described here can be further developed into a common immunoassays platform for PAEs in food and environmental science.
Disclosure statement
No potential conflict of interest was reported by the author(s).
Additional information
Funding
References
- Benning, J. L., Liu, Z., Tiwari, A., Little, J. C., & Marr, L. C. (2013). Characterizing gas-particle interactions of phthalate plasticizer emitted from vinyl flooring. Environmental Science & Technology, 47(6), 2696–2703. doi: 10.1021/es304725b.
- Chen, G., Jin, M., Du, P., Zhang, C., Cui, X., Zhang, Y., … Zheng, L. (2017). A review of enhancers for chemiluminescence enzyme immunoassay. Food and Agricultural Immunology, 28(2), 315–327. doi: 10.1080/09540105.2016.1272550
- Chen, Y., Kong, D., Liu, L., Song, S., Kuang, H., & Xu, C. (2015). Development of an ELISA and immunochromatographic assay for tetracycline, oxytetracycline, and chlortetracycline residues in milk and honey based on the class-specific monoclonal antibody. Food Analytical Methods, 9(4), 905–914. doi: 10.1007/s12161-015-0262-z
- Cooper, M. D. (2015). The early history of B cells. Nature Reviews Immunology, 15(3), 191–197. doi: 10.1038/nri3801
- Cui, X., Wu, P., Lai, D., Zheng, S., Chen, Y., Eremin, S. A., & Zhao, S. (2015). Development of a highly specific fluorescence immunoassay for detection of diisobutyl phthalate in edible oil samples. Journal of Agricultural and Food Chemistry, 63(42), 9372–9378. doi: 10.1021/acs.jafc.5b03922
- Deng, L.-H., Dai, J.-B., Xu, Z.-L., Yang, J.-Y., Wang, H., Xiao, Z.-L., … Shen, Y.-D. (2016). Application of time-resolved fluroimmunoassay for determination of furaltadone metabolite 3-amino-5-morpholinomethyl-2-oxazolidinone. Chinese Journal of Analytical Chemistry, 44(8), 1286–1290. doi: 10.1016/s1872-2040(16)60951-9
- Draviam, E. J., Kerkay, J., & Pearson, K. H. (2006). Separation and quantitation of urinary phthalates by HPLC. Analytical Letters, 13(13), 1137–1155. doi: 10.1080/00032718008055721
- Feng, X. L., Lu, S. Y., Liu, D., Li, L., Wu, X. Z., Song, J., … Ren, H. L. (2013). Direct competitive immunosorbent assay for detection of MEHP in human urine. Chemosphere, 92(1), 150–155. doi: 10.1016/j.chemosphere.2013.02.062
- Gao, H., Zhu, B. B., Tao, X. Y., Zhu, Y. D., Tao, X. G., & Tao, F. B. (2018). Temporal variability of cumulative risk assessment on phthalates in Chinese pregnant women: Repeated measurement analysis. Environmental Science & Technology, 52(11), 6585–6591. doi: 10.1021/acs.est.7b06681
- Gartner, S., Balski, M., Koch, M., & Nehls, I. (2009). Analysis and migration of phthalates in infant food packed in recycled paperboard. Journal of Agricultural and Food Chemistry, 57(22), 10675–10681. doi: 10.1021/jf902683m
- Gong, M., Weschler, C. J., & Zhang, Y. (2016). Impact of clothing on dermal exposure to phthalates: Observations and insights from sampling both skin and clothing. Environmental Science & Technology, 50(8), 4350–4357. doi: 10.1021/acs.est.6b00113
- Guo, Y., & Kannan, K. (2013). A survey of phthalates and parabens in personal care products from the United States and its implications for human exposure. Environmental Science & Technology, 47(24), 14442–14449. doi: 10.1021/es4042034
- Han, Y., Diao, D., Lu, Z., Li, X., Guo, Q., Huo, Y., … Lou, X. (2017). Selection of group-specific phthalic acid esters binding DNA aptamers via rationally designed target immobilization and applications for ultrasensitive and highly selective detection of phthalic acid esters. Analytical Chemistry, 89(10), 5270–5277. doi: 10.1021/acs.analchem.6b04808
- Hernandez, L. M., Xu, E. G., Larsson, H. C. E., Tahara, R., Maisuria, V. B., & Tufenkji, N. (2019). Plastic teabags release billions of microparticles and nanoparticles into tea. Environmental Science & Technology, 53(21), 12300–12310. doi: 10.1021/acs.est.9b02540
- Hsiao, N.-C., Wu, Y.-M., Zhao, L., Chen, D.-Y., Huang, W.-T., Kuo, K.-H., … Leu, P.-L. (2011). A new prototype system for earthquake early warning in Taiwan. Soil Dynamics and Earthquake Engineering, 31(2), 201–208. doi: 10.1016/j.soildyn.2010.01.008
- Hsu, J. F., Peng, L. W., Li, Y. J., Lin, L. C., & Liao, P. C. (2011). Identification of di-isononyl phthalate metabolites for exposure marker discovery using in vitro/in vivo metabolism and signal mining strategy with LC-MS data. Analytical Chemistry, 83(22), 8725–8731. doi: 10.1021/ac202034k
- Insuan, W., Khawmodjod, P., Whitlow, H. J., Soonthondecha, P., Malem, F., & Chienthavorn, O. (2016). High-throughput and low-cost analysis of trace volatile phthalates in seafood by online coupling of monolithic capillary adsorbent with GC-MS. Journal of Agricultural and Food Chemistry, 64(16), 3287–3292. doi: 10.1021/acs.jafc.6b00742
- Jiang, K., Sun, S., Zhang, L., Wang, Y., Cai, C., & Lin, H. (2015). Bright-yellow-emissive N-doped carbon dots: Preparation, cellular imaging, and bifunctional sensing. ACS Applied Materials & Interfaces, 7(41), 23231–23238. doi: 10.1021/acsami.5b07255
- Kai, T., Chen, S., Monterroso, E., & Zhou, F. (2015). Continuous nanoflow-scanning electrochemical microscopy: Voltammetric characterization and application for accurate and reproducible imaging of enzyme-labeled protein microarrays. Analytical Chemistry, 87(8), 4523–4529. doi: 10.1021/acs.analchem.5b00625
- Katsikantami, I., Sifakis, S., Tzatzarakis, M. N., Vakonaki, E., Kalantzi, O. I., Tsatsakis, A. M., & Rizos, A. K. (2016). A global assessment of phthalates burden and related links to health effects. Environment International, 97, 212–236. doi: 10.1016/j.envint.2016.09.013
- Kuang, H., Liu, L., Xu, L., Ma, W., Guo, L., Wang, L., & Xu, C. (2013). Development of an enzyme-linked immunosorbent assay for dibutyl phthalate in liquor. Sensors (Basel), 13(7), 8331–8339. doi: 10.3390/s130708331
- Kuang, H., Xu, L., Cui, G., Ma, W., & Xu, C. (2010). Development of determination of di-n-octyl phthalate (DOP) residue by an indirect enzyme-linked immunosorbent assay. Food and Agricultural Immunology, 21(3), 265–277. doi: 10.1080/09540101003758962
- Li, M., Cui, Y., Liu, Z., Xue, Y., Zhao, R., Li, Y., & Du, D. (2019). Sensitive and selective determination of butyl benzyl phthalate from environmental samples using an enzyme immunoassay. Science of The Total Environment, 687, 849–857. doi: 10.1016/j.scitotenv.2019.06.037
- Li, D., Cui, Y., Morisseau, C., Gee, S. J., Bever, C. S., Liu, X., … Ying, Y. (2017). Nanobody based immunoassay for human soluble epoxide hydrolase detection using polymeric horseradish peroxidase (PolyHRP) for signal enhancement: The rediscovery of PolyHRP? Analytical Chemistry, 89(11), 6248–6256. doi: 10.1021/acs.analchem.7b01247
- Li, L., Lian, Z., Yan, X., Xia, M., & Zhang, M. (2018). An evaporation induced self-assembly approach to prepare polymorphic carbon dot fluorescent nanoprobes for protein labelling. Chemical Communications, 54(93), 13123–13126. doi: 10.1039/c8cc05860a
- Li, D., Panchal, K., Mafi, R., & Xi, L. (2018). An atomistic evaluation of the compatibility and plasticization efficacy of phthalates in poly(vinyl chloride). Macromolecules, 51(18), 6997–7012. doi: 10.1021/acs.macromol.8b00756
- Li, L., Zhou, Y., Li, Y.-S., Feng, X.-L., Song, J., Liu, Y.-Y., … Ren, H.-L. (2013). Preparation of an antigen and development of a monoclonal antibody against mono-butyl phthalate (MBP). Food and Agricultural Immunology, 24(2), 193–202. doi: 10.1080/09540105.2012.677010
- Lim, S. Y., Shen, W., & Gao, Z. (2015). Carbon quantum dots and their applications. Chemical Society Reviews, 44(1), 362–381. doi: 10.1039/c4cs00269e
- Lin, J., Chen, W., Zhu, H., & Wang, C. (2015). Determination of free and total phthalates in commercial whole milk products in different packaging materials by gas chromatography-mass spectrometry. Journal of Dairy Science, 98(12), 8278–8284. doi: 10.3168/jds.2015-10066
- Maitre, L., Robinson, O., Martinez, D., Toledano, M. B., Ibarluzea, J., Marina, L. S., … Coen, M. (2018). Urine metabolic signatures of multiple environmental pollutants in pregnant women: An exposome approach. Environmental Science & Technology, 52(22), 13469–13480. doi: 10.1021/acs.est.8b02215
- Net, S., Sempere, R., Delmont, A., Paluselli, A., & Ouddane, B. (2015). Occurrence, fate, behavior and ecotoxicological state of phthalates in different environmental matrices. Environmental Science & Technology, 49(7), 4019–4035. doi: 10.1021/es505233b
- O'Connell, S. G., Kincl, L. D., & Anderson, K. A. (2014). Silicone wristbands as personal passive samplers. Environmental Science & Technology, 48(6), 3327–3335. doi: 10.1021/es405022f
- Qiao, J., Wang, M., Yan, H., & Yang, G. (2014). Dispersive solid-phase extraction based on magnetic dummy molecularly imprinted microspheres for selective screening of phthalates in plastic bottled beverages. Journal of Agricultural and Food Chemistry, 62(13), 2782–2789. doi: 10.1021/jf4051705
- Schettler, T. (2006). Human exposure to phthalates via consumer products. International Journal of Andrology, 29(1), 134–139; discussion 181–135. doi: 10.1111/j.1365-2605.2005.00567.x
- Shi, S., Cao, J., Zhang, Y., & Zhao, B. (2018). Emissions of phthalates from indoor flat materials in Chinese residences. Environmental Science & Technology, 52(22), 13166–13173. doi: 10.1021/acs.est.8b03580
- Sun, S., Zhang, L., Jiang, K., Wu, A., & Lin, H. (2016). Toward high-efficient Red Emissive carbon dots: Facile preparation, unique properties, and applications as multifunctional Theranostic agents. Chemistry of Materials, 28(23), 8659–8668. doi: 10.1021/acs.chemmater.6b03695
- Sun, R., & Zhuang, H. (2014). A sensitive heterogeneous biotin–streptavidin enzyme-linked immunosorbent assay for the determination of di-(2-ethylhexyl)phthalate (DEHP) in beverages using a specific polyclonal antibody. Analytical Methods, 6(24), 9807–9815. doi: 10.1039/c4ay02088j
- Sun, R., & Zhuang, H. (2015a). Biotin-streptavidin enzyme-linked Immunosorbent Assay for the determination of dibutyl phthalate in beverages and drinking water using a specific polyclonal antibody. Food Analytical Methods, 8(8), 1990–1999. doi: 10.1007/s12161-014-0085-3
- Sun, R., & Zhuang, H. (2015b). Development of a highly sensitive biotin–streptavidin enzyme-linked immunosorbent assay for detecting diethyl phthalate based on a specific polyclonal antibody. Food and Agricultural Immunology, 26(5), 746–760. doi: 10.1080/09540105.2015.1027666
- Sun, R., & Zhuang, H. (2015c). Biotin–streptavidin-amplified real-time immune-PCR assay for detecting dimethyl phthalate in beverage and drinking water samples. Analytical and Bioanalytical Chemistry, 407(4), 1261–1265. doi: 10.1007/s00216-014-8329-z
- Sun, R. Y., & Zhuang, H. S. (2015d). An indirect competitive biotin-streptavidin enzyme-linked immunosorbent assay for the determination of dimethyl phthalate (DMP) in milk and milk products. Journal of Environmental Science and Health, Part B, 50(4), 275–284. doi:10.1080/03601234.2015.999601.
- Tang, M., Wei, J., Du, H., Zhang, J., Yang, D., & Peng, Y. (2015). Synthesis of an artificial antigen and preparation of a polyclonal antibody for the sensitive determination of phthalate esters by enzyme-linked immunoassay. Analytical Methods, 7(8), 3402–3410. doi: 10.1039/c5ay00079c
- Tian, X., Dong, Y., Wang, Y., Song, Z., Meng, M., Eremin, S. A., … Xi, R. (2015). Quantification of diethyl phthalate by a rapid and homogenous fluorescence polarization immunoassay. Analytical Letters, 48(18), 2843–2855. doi: 10.1080/00032719.2015.1060600
- Tochi, B. N., Peng, J., Song, S., Liu, L., Kuang, H., & Xu, C. (2016a). Determination of sarafloxacin and its analogues in milk using an enzyme-linked immunosorbent assay based on a monoclonal antibody. Analytical Methods, 8(7), 1626–1636. doi: 10.1039/c5ay02702k
- Tochi, B. N., Peng, J., Song, S., Liu, L., Kuang, H., & Xu, C. (2016b). Production and application of a monoclonal antibody (mAb) against ofloxacin in milk, chicken and pork. Food and Agricultural Immunology, 27(5), 643–656. doi: 10.1080/09540105.2016.1148125
- Tran, D. N., & Balkus, K. J. (2011). Perspective of recent Progress in immobilization of enzymes. ACS Catalysis, 1(8), 956–968. doi: 10.1021/cs200124a
- Vavrous, A., Sevcik, V., Dvorakova, M., Cabala, R., Moulisova, A., & Vrbik, K. (2019). Easy and inexpensive method for multiclass analysis of 41 food contact related contaminants in fatty food by liquid chromatography-tandem mass spectrometry. Journal of Agricultural and Food Chemistry, 67(39), 10968–10976. doi: 10.1021/acs.jafc.9b02544
- Wang, H., Chen, Q., & Zhou, S. (2018). Carbon-based hybrid nanogels: A synergistic nanoplatform for combined biosensing, bioimaging, and responsive drug delivery. Chemical Society Reviews, 47(11), 4198–4232. doi: 10.1039/c7cs00399d
- Wang, P. L., Xie, L. H., Joseph, E. A., Li, J. R., Su, X. O., & Zhou, H. C. (2019). Metal-Organic Frameworks for food safety. Chemical Reviews, 119(18), 10638–10690. doi: 10.1021/acs.chemrev.9b00257
- Wang, L., Zhang, Y., Gao, X., Duan, Z., & Wang, S. (2010). Determination of chloramphenicol residues in milk by enzyme-linked immunosorbent assay: Improvement by biotin-streptavidin-amplified system. Journal of Agricultural and Food Chemistry, 58(6), 3265–3270. doi: 10.1021/jf903940h
- Wei, C., Ding, S., You, H., Zhang, Y., Wang, Y., Yang, X., & Yuan, J. (2011). An immunoassay for dibutyl phthalate based on direct hapten linkage to the polystyrene surface of microtiter plates. Plos One, 6(12), e29196. doi: 10.1371/journal.pone.0029196
- Wei, S. C., Hsu, P. H., Lee, Y. F., Lin, Y. W., & Huang, C. C. (2012). Selective detection of iodide and cyanide anions using gold-nanoparticle-based fluorescent probes. ACS Applied Materials & Interfaces, 4(5), 2652–2658. doi: 10.1021/am3003044
- Wu, Y. T., Shanmugam, C., Tseng, W. B., Hiseh, M. M., & Tseng, W. L. (2016). A gold nanocluster-based fluorescent probe for simultaneous pH and temperature sensing and its application to cellular imaging and logic gates. Nanoscale, 8(21), 11210–11216. doi: 10.1039/c6nr02341j
- Xiong, L. H., He, X., Zhao, Z., Kwok, R. T. K., Xiong, Y., Gao, P. F., … Tang, B. Z. (2018). Ultrasensitive virion immunoassay platform with dual-modality based on a multifunctional aggregation-induced emission luminogen. ACS Nano, 12(9), 9549–9557. doi: 10.1021/acsnano.8b05270
- Xu, Z. L., Sun, W. J., Yang, J. Y., Jiang, Y. M., Campbell, K., Shen, Y. D., … Sun, Y. M. (2012). Development of a solid-phase extraction coupling chemiluminescent enzyme immunoassay for determination of organophosphorus pesticides in environmental water samples. Journal of Agricultural and Food Chemistry, 60(9), 2069–2075. doi: 10.1021/jf300225b
- Xu, F., Wang, W., Jiang, H., Wang, Z., Wang, Z., Guo, P., … Ding, S. (2014). Indirect competitive enzyme-linked immunosorbent assay for the detection of dibutyl phthalate in White wine, compared with GC-MS. Food Analytical Methods, 7(8), 1619–1626. doi: 10.1007/s12161-014-9797-7
- Xu, Z., Xiong, X., Zhao, Y., Xiang, W., & Wu, C. (2019). Pollutants delivered every day: Phthalates in plastic express packaging bags and their leaching potential. Journal of Hazardous Materials, 384, 121282. doi: 10.1016/j.jhazmat.2019.121282
- Yan, Y., Lu, Y., Wang, B., Gao, Y., Zhao, L., Liang, H., & Wu, D. (2018). Self-assembling hydrophilic magnetic covalent organic framework nanospheres as a novel matrix for phthalate ester recognition. ACS Applied Materials & Interfaces, 10(31), 26539–26545. doi: 10.1021/acsami.8b08934
- Yang, T., Li, C. M., He, J. H., Chen, B., Li, Y. F., & Huang, C. Z. (2018). Ratiometrically fluorescent electrospun nanofibrous film as a Cu(2+)-mediated solid-phase immunoassay platform for biomarkers. Analytical Chemistry, 90(16), 9966–9974. doi: 10.1021/acs.analchem.8b02286
- Yang, R., Liu, Y., Yan, X., Liu, S., & Zheng, H. (2016). An effective method for the synthesis of yolk–shell magnetic mesoporous carbon-surface molecularly imprinted microspheres. Journal of Materials Chemistry A, 4(25), 9807–9815. doi: 10.1039/c6ta00889e
- Yao, C. Y., Xu, Z. L., Wang, H., Zhu, F., Luo, L., Yang, J. Y., & Shen, Y. D. (2019). High affinity antibody based on a rationally designed hapten and development of a chemiluminescence enzyme immunoassay for quantification of Alternariol in fruit Juice, maize and flour. Food Chemistry, 283, 359–366. doi: 10.1016/j.foodchem.2018.12.127
- You, L., Wang, Y. X., Zeng, Q., Li, M., Huang, Y. H., Hu, Y., … Lu, W. Q. (2015). Semen phthalate metabolites, spermatozoa apoptosis, and DNA damage: A cross-sectional study in China. Environmental Science & Technology, 49(6), 3805–3812. doi: 10.1021/acs.est.5b00588
- Zeng, H., Chen, J., Zhang, C., Huang, X. A., Sun, Y., Xu, Z., & Lei, H. (2016). Broad-specificity chemiluminescence enzyme immunoassay for (fluoro)quinolones: Hapten design and molecular modeling study of antibody recognition. Analytical Chemistry, 88(7), 3909–3916. doi: 10.1021/acs.analchem.6b00082
- Zhang, M., Cong, Y., Sheng, Y., & Liu, B. (2010). A direct competitive enzyme-linked immunosorbent assay by antibody coated for diethyl phthalate analysis. Analytical Biochemistry, 406(1), 24–28. doi: 10.1016/j.ab.2010.06.040
- Zhang, M., Hong, W., Wu, X., Zhang, Y., Li, F., & Zhao, S.-Q. (2015). A highly sensitive and direct competitive enzyme-linked immunosorbent assay for the detection of di-(2-ethylhexyl) phthalate (DEHP) in infant supplies. Analytical Methods, 7(13), 5441–5446. doi: 10.1039/c5ay00207a
- Zhang, M., Hu, Y., Liu, S., Cong, Y., Liu, B., & Wang, L. (2012). Rapid monitoring of dipropyl phthalate in food samples using a chemiluminescent enzyme immunoassay. Food Analytical Methods, 5(5), 1105–1113. doi:10.1007/s12161-011-9346-6.
- Zhang, M., Hu, Y., Liu, S., Cong, Y., Liu, B., & Wang, L. (2013). A highly sensitive enzyme-linked immunosorbent assay for the detection of dipropyl phthalate in plastic food contact materials. Food and Agricultural Immunology, 24(2), 165–177. doi:10.1080/09540105.2012.670615.
- Zhang, M., Liu, B., Cong, Y., Liu, S., & Hu, Y. (2011). Development of highly specific fluorescence immunoassay and enzyme-linked immunosorbent assay for detection of dimethyl phthalate in water samples. Food and Agricultural Immunology, 22(4), 297–309. doi: 10.1080/09540105.2011.575124
- Zhang, M., Liu, S., Zhuang, H., & Hu, Y. (2012). Determination of dimethyl phthalate in environment water samples by a highly sensitive indirect competitive ELISA. Applied Biochemistry and Biotechnology, 166(2), 436–445. doi:10.1007/s12010-011-9439-0.
- Zhang, G., Quin, M. B., & Schmidt-Dannert, C. (2018). Self-assembling protein Scaffold system for easy in vitro coimmobilization of biocatalytic cascade enzymes. ACS Catalysis, 8(6), 5611–5620. doi: 10.1021/acscatal.8b00986
- Zhang, M., & Sheng, Y. (2010). An indirect competitive fluorescence immunoassay for determination of dicyclohexyl phthalate in water samples. Journal of Fluorescence, 20(6), 1167–1173. doi: 10.1007/s10895-010-0663-8
- Zhang, M., Wang, Y., Yu, X., Hu, Y., & Liu, S. (2013). Rapid monitoring of dicyclohexyl phthalate in foods using the direct competitive ELISA. Food and Agricultural Immunology, 25(2), 229–242. doi:10.1080/09540105.2013.768964.
- Zhang, M. C., Wang, Q. E., & Zhuang, H. S. (2006). A novel competitive fluorescence immunoassay for the determination of dibutyl phthalate. Analytical and Bioanalytical Chemistry, 386(5), 1401–1406. doi: 10.1007/s00216-006-0703-z
- Zhang, M. C., Wang, Q. E., & Zhuang, H. S. (2007). Determination of dibutyl o-phthalate by antigen-coated competitive fluorescence immunoassay. Analytical Letters, 40(1), 127–137. doi: 10.1080/00032710600952564
- Zhang, M., Yu, X., Wang, Y., Hu, Y., & Liu, S. (2012). A highly sensitive indirect competitive enzyme-linked immunosorbent Assay (ic-ELISA) by antigen coating for diethyl phthalate analysis in Foods. Food Analytical Methods, 6(4), 1223–1228. doi:10.1007/s12161-012-9529-9.
- Zhu, F., Mao, C., & Du, D. (2017). Time-resolved immunoassay based on magnetic particles for the detection of diethyl phthalate in environmental water samples. Science of The Total Environment, 601–602, 723–731. doi: 10.1016/j.scitotenv.2017.05.111
- Zhu, F., Zhang, H., Qiu, M., Wu, N., Zeng, K., & Du, D. (2019). Dual-label time-resolved fluoroimmunoassay as an advantageous approach for investigation of diethyl phthalate & dibutyl phthalate in surface water. Science of The Total Environment, 695, 133793. doi: 10.1016/j.scitotenv.2019.133793
- Zhu, B., Zhu, F., Xu, Z.-L., Yang, J.-Y., Liu, C.-H., Sun, Y.-M., & Shen, Y.-D. (2015). Indirect competitive chemiluminescent enzyme immunoassay method for determination of dimethyl phthalate in Soy sauce and liquor. Chinese Journal of Analytical Chemistry, 43(7), 1027–1032. doi: 10.1016/s1872-2040(15)60839-8