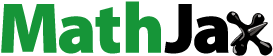
ABSTRACT
In the current study, a rapid and novel biomimetic nano-enzyme-linked immunosorbent assay has been established for the detection of sulfadiazine (SDZ) using Au@SiO2 nanoparticles labelling as a marker. In this system, gold nanoparticles exhibited intrinsic peroxidase and activity with excellent water-solubility, and the SiO2 nanoparticles as ideal carries possessed a large surface area and ease of surface functionalization. The molecularly imprinted polymer films were selected as bionic antibody because of its advantages of selective recognition and reusability. Combine the above advantages, the method exhibited good stability, recognition capability and sensitivity. Under the optimal condition, the limit of detection of the immunosorbent assay was 0.2 mg/L. Beef sample spiked with SDZ was analysed, and the recoveries were in the 78.00% to 90.96% range. The assay could be used to detect SDZ residues in pork and chicken samples, and the results were not significantly different from those obtained by high-performance liquid chromatography.
Introduction
Sulfonamides (SAs) are commonly used in veterinary drugs. Sulfadiazine (SDZ) belongs to the SA class of antibacterial compounds. However, misuse can lead to residues in food animals and the environment. Excessive SDZ in food and the environment can have harmful effects, such as anaphylactic reactions (Hiba, Carine, Haifa, Ryszard, & Farouk, Citation2016), poisoning (Khalili, Soudbakhsh, & Talasaz, Citation2011), kidney failure or urinary stones (Dognon, Degand, Douny, Delahaut, Igout, Dahouda, Youssao, & Scippo, Citation2018). A rapid and sensitive analytical approach for the determination of SDZ is urgently needed.
Various researches for detecting of SDZ have been reported, including gas chromatography (GC) (Santos & Ramos, Citation2016), high-performance liquid chromatography (HPLC) (Vosough, Onilghi, & Salemi, Citation2016) and liquid chromatography coupled to mass spectrometry (Danezis, Anagnostopoulos, Liapis, & Koupparis, Citation2016; Jansomboon, Boontanon, Boontanon, Polprasert, & Da, Citation2016; Robert, Brasseur, Dubois, Delahaut, & Gillard, Citation2016). However, these methods are tedious and time-consuming. Therefore, they are not suitable for the quick on-site testing. Immunosorbent assays represent an alternative method widely used to detect the SDZ, with high sensitivity and cost effectiveness (Chu, Xu, He, Wang, & Lu, Citation2011). Enzyme-linked immunosorbent assay (ELISA) is a common approach to detect veterinary drug residues in foods because of its high sensitivity, simple operation and economic benefit (Liu et al., Citation2012; Shen et al., Citation2011; Song, Wang, Zhang, & Wang, Citation2011; Sun & Zhuang, Citation2015). However, this approach still has some shortcomings concerning the required biological antibodies such as low stability, a short lifetime and lack of reusability; therefore, the immunosorbent assay should be developed and optimized in the detection of SDZ (Jiang et al., Citation2013).
Molecular imprinting technology has been widely used in bionic immunoassay because of its ability for synthesizing artificial antibodies (Wang, Jiang, Ju, Qiao, & Xu, Citation2017). Molecularly imprinted polymers (MIPs) have the advantages of simple fabrication, stability and reusability, and have been widely applied in veterinary drug analysis (Lata, Sharma, Naik, Raiput, & Mann, Citation2015; Li et al., Citation2016; Shi et al., Citation2011; Tang et al., Citation2017). In recent years, remarkable progress in developing artificial antibodies for MIP-coated 96-well microplates has been achieved(Bi & Liu, Citation2014), and many biomimetic enzyme-linked immunosorbent assays (BELISA) have been developed, which have enhanced identification capabilities and decreased costs (Tang et al., Citation2017; Zhang, Cui, Han, Yu, & Shi, Citation2017). However, natural enzymes are macromolecules and have structural shielding effects, which limit its application in the immunosorbent assay (Jiang, Wu, Xu, Qiao, & Xu, Citation2017). Therefore, in the biomimetic immunosorbent assay, a new marker to replace the enzyme is necessary.
Artificial enzyme mimics have been attracting increasing attention over the past few years (Wiester, Ulmann, & Mirkin, Citation2011). Recently, gold nanoparticles (AuNPs) were reported to exhibit peroxidase enzyme-like properties, and they have been applied in bionics, biosensings and biomedicines (Deng et al., Citation2014; Lin, Ren, & Qu, Citation2014; Shah, Purohit, Singh, Karakoti, & Singh, Citation2015). The appearance and recent progress of nanotechnology provides new opportunities for the development of nanoparticles, which have stable and high catalytic activity (Yamada et al., Citation2011). Silica has been shown to be a multifunctional biomaterial with its good biocompatibility, which can directly improve the stability of nanoparticles in complex biological systems. SiO2 nanoparticles are ideal carriers which provide as large surface area and ease of surface functionalization (Huang et al., Citation2014; Mao, Bao, Han, Li, & Niu, Citation2012; Shao et al., Citation2014). More importantly, these nanomaterials are much smaller than natural enzyme and therefore have potential as a marker for the BELISA method.
In this research, we reported a sensitive direct competitive BELISA method for the detection of SDZ. The assay used the MIP film as a bionic antibody for recognition and Au@SiO2 nanostructures labelled as a marker takes the place of horseradish peroxidase (HRP). These factors were optimized and the peroxidase activity of the nanostructures was evaluated.
Material and methods
Material and reagents
Pork, beef and chicken samples were obtained from a supermarket (Taian, Shandong) in May 2019.
The SDZ ( > 98%) was purchased from the Shandong Xiya chemical industry Co., Ltd. (Linyi, China). Sodium borohydride (NaBH4) was purchased from Tianjin Kemiou Chemical Reagent Co., Ltd. (Tianjin, China). Cetyltrimethylammonium bromide (CTAB), 3,3’,5,5’-tetramethylbenzidine (TMB) and chlorauric acid (HAuCl4·3H2O) were obtained from Beijing Biotopped Science & Technology Co., Ltd. (Beijing, China). Ethylsilicate (TEOS) was from Shanghai Macklin Biochemical Co., Ltd. (Shanghai, China). 3-aminopropyl triethoxysilane (APTES) was obtained from Shanghai Yuanye Bio-Technology Co., Ltd. (Shanghai, China). Methacrylic acid (MAA), 2,2-azobisisobutyronitrile (AIBN), methanol, acetic acid and acetonitrile were from Tianjin Chemical Reagent Factory (Tianjin, China). MAA was vacuum distilled and AIBN was recrystallized before use. Other materials and solutions are included in the supplementary material.
Apparatus
Microlon 96-well plates (Costar®, Corning) were purchased from Beijing Biolead Biology Sci & Tech Co., Ltd. (Beijing, China). The 96-well plates were washed in a ST-36wet microplate washer (Kehua Bio-engineering Co., Ltd., Shanghai, China). Immunoassay absorbance was read with a Multimode Plate Reader (Molecular Devices, Sunnyvale, CA). The wavelength was set in a dual-wavelength mode (450–650 nm).
Synthesis of Au nanorods (NRs)
Au NRs were synthesized by a seed-mediated method (He et al., Citation2011). First, 7.5 mL of CTAB (0.1 M) aqueous solution was mixed with 100 μL of HAuCl4 (24 mM), and then 1.8 mL of deionized water was added to dilute the mixture. After that, 0.6 mL of ice-cold NaBH4 (0.01M) was added quickly. The solution was stirred by stirred magnetically for 3 min, the colour of the solution changed from bright yellow to brown, which indicated that the Au seeds were formed. A 240 μL of the Au seed was added to the preparation growth solution to incubate at 28°C for 12 h, and then the Au NRs were purified by centrifugation (12,000 rpm, 10 min) twice. The sediment was redispersed in double distilled water.
The details of preparing the growth solution are mentioned in supplementary material.
Synthesis of Au@SiO2 NRs
All reactions were conducted in a temperature-controlled water bath at 30°C. Firstly, 10 mL of the Au NRs was centrifugated (6500 rpm/min, 10 min) twice and was then dissolved in double distilled water. The precipitate was collected and dissolved in 10 mL of deionized water. Then, the pH of the above solution was adjusted to around 10.6 using a small quantity of NaOH (0.1 M) solution. The mixture was stirred magnetically for 20 min, and then three 10 μL of TEOS ethanol solution (20% v/v) were added at 30-min intervals by stirred magnetically. Finally, this mixed solution was reacted at 30°C for 24 h.
Surface modification of Au@SiO2 NRs
First, 20 mL of Au@SiO2 was centrifuged (6500 rpm/min, 10 min), and then successively dispersed in ethanol, ethyl acetate and toluene solution. Thus, 5 mL of Au@SiO2 toluene solution was obtained. Finally, 20 μL of APTES was added, and then the mixture solution was treated with ultrasound for 2 h, before two washes in ethyl acetate and ethanol.
Preparation of the nanozyme conjugate
Conjugation of the SDZ antigen and the Au@SiO2 NRs was performed as follows: 5 mL of Au@SiO2 NRs and 10 μL of glutaraldehyde (25% aqueous solution) were added to a reaction vessel. After stirring by magnetically stirred for 1 h, an equimolar mass of SDZ solution was added, then the solution was stirred for another 1 h. Finally, these products were washed to remove unreacted materials and preserved at 4°C.
Synthesis of MIP film
The MIP and non-molecularly imprinted polymer (NIP) films were prepared on the surface of 96-well plates. The preparation process is described in supplementary material.
Direct competitive BELISA procedure
The details of the procedure are introduced in supplementary material and .
Sample preparation
To determine the feasibility of the experiment, a beef sample was assessed, which was determined that the beef samples did not contain SDZ through HPLC. Briefly, the beef sample was minced, and 5.0 g was placed in a 50 mL container. After 1.0 mL of a SDZ standard solution (0.50, 2.50, 12.50 mg/L) were spiked, the mixtures were reacted at 4°C for 24 h. After that, the mixtures were extracted using 20 mL of ethyl acetate twice. The resulting extracts were collected and the fluid was evaporated. Then 1 mL of methanol was added to redissolve the solids. The liquid product was analysed by the BELISA procedure after filtering with a 0.22-μm membrane.
To detect SDZ residues, pork and chicken samples were prepared described above, without the addition of a SDZ standard. The extract was studied by the BELISA procedure, and the content of SDZ was determined.
Results and discussions
Characterization of Au@SiO2 nanostructures
The absorption spectra of the Au NRs and Au@SiO2 nanoparticles were assessed through UV-vis-NIR (Figure S2). The Au NRs had an absorption peak at 810 nm, which indicated that the Au NRs were synthesized successfully. Au NRs were coated with mesoporous silica to form core–shell Au@SiO2 nanostructures. After coating with SiO2 nanoparticles, the band was red-shifted to 906 nm, which indicated that Au NRs@SiO2 nanostructures were formed.
Characterization of the imprinted film
The FT-IR spectra of the imprinted film after extraction (a), the imprinted film before extraction (b), the non-imprinted film (c) and SDZ (d) are compared in . The SDZ stretching peak near 3381 and 3453 cm−1, indicating an N-H stretching vibration, and the peak near 1108 cm−1 were assigned to S = O group. For the MIP film before extraction, the features around 1105 cm−1 was identified as an S = O stretching vibrations. The stretch shift (S = O) might be caused by the oxygen atoms of S = O in SDZ combining with the hydrogen atoms of –OH in MAA to form hydrogen bonds. However, the S = O stretching vibration peak was not found in the imprinted film after extraction indicating that the SDZ was extracted completely. These results demonstrated that the MIP film was prepared successfully.
Thermo-gravimetric analysis (TGA)
A TGA curve of the imprinted film is depicted in Figure S3. When the temperature was between 0°C and 370°C, there was no significant reduction in the mass of MIP film. When the temperature exceeded 370°C, the mass decrease sharply. This result showed that the imprinted film has good thermal stability.
Static adsorption experiment
The adsorption capacity is an important property for the imprinted film. A static adsorption experiment was performed in a concentration range of 100 mg/L to 500 mg/L. As shown in Figure S4, the adsorbability of the MIP film and the NIP film increased with increasing SDZ concentrations. The MIP film exhibited a better adsorption ability for SDZ than the NIP film. The adsorption capacity of the MIP film (18.38 μg/well) was more than 1.6 times that of the NIP film (11.53 μg/well) at a 300 mg/L concentration.
Optimization of the BELISA conditions
The sensitive can be improved by optimizing the conditions of BELISA procedure, including the reaction solution. The recognition capability and selectivity of the MIP film towards the SDZ were influenced deeply by the solvent used for the preparation of the standard solution and samples, hence it influenced the accuracy of the BELISA procedure. Methanol, ethyl alcohol and acetonitrile were studied for use as preparation solutions. As shown in , the results indicated that when methanol was used the inhibition ratio and the sensitivity of the method were better than those with other solutions. Therefore, methanol used throughout the experiment as the preparation solution.
Selectivity of the BELISA assay
The selectivity of MIP and NIP films were investigated () using SDZ standard solution with 0.0512–160 mg/L concentrations in methanol. In contrast, the inhibition rate of the MIP film was higher at the same concentration of SDZ. From the standard curves, when the inhibition ratio was 50% and the SDZ concentration of the imprinted film was 32 mg/L, while that of the NIP film was 163 mg/L. Above results demonstrated that the MIP film had better recognition capability and higher sensitive for SDZ than the NIP film.
Figure 3. Standard BELISA competition curves for sulfadiazine with concentrations of 0.0512–160 mg/L in methanol.
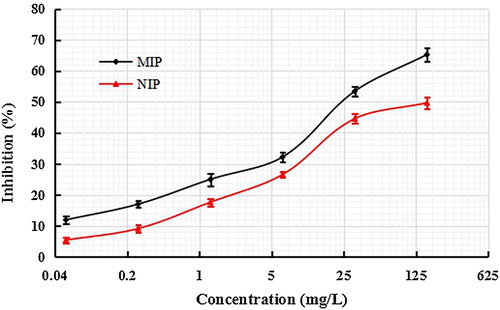
To evaluate the specificity of the BELISA method, two structural analogues of SDZ (sulfathiazole and sulfamethazine) were applied to the competitive assay. Standard curves are established in . The cross-reactivity (CR) was calculated as the percentage between the IC50 values for SDZ and that for other interfering compound using the following the equation:
The analysis is presented in . The cross-reactivity values for sulfamethazine and sulfathiazole were 19.7% and 30.6%, respectively, which demonstrated that the MIP film had a lower absorption towards structural analogues than the SDZ. The results determined that the MIP film has advantages to be used as artificial antibodies in the BELISA method.
Table 1. The cross-reactivity (CR) ratio of three kinds of antibiotics.
Parameters of the BELISA method
The standard curve was assessed by SDZ in methanol under optimal conditions. The limit of detection (LOD, IC15) of the method was 0.2 mg/L (40 μg/kg). According to the Ministry of Agriculture of the People’s Republic of China (NY 5070–2002), the maximum allowable residue limit of SDZ is 100 μg/kg. Therefore, the developed BELISA method can effectively applied to the determination of SDZ in food.
Accuracy of the BELISA assay
The accuracy and sensitivity of the proposed method were evaluated. The beef sample spiked with sulafdiazine at different levels of 0.5, 2.5 and 12.5 mg/L were assessed using the developed BELISA method. The results are analysed in . Good recoveries of 78.00%–90.96% were achieved.
Table 2. The recoveries of spiked sulfadiazine in beef samples by determination using the BELISA method.
To validate the applicability of the BELISA method, pork and chicken samples were analysed by HPLC. The SDZ in the pork and chicken samples at levels of 3.56 ± 0.03 μg/mL and 5.88 ± 0.02 μg/mL, respectively, as assessed through HPLC. The resulting concentrations obtained by BELISA method were 3.11 ± 0.02 μg/mL and 5.66 ± 0.05 μg/mL. There was no significant difference between the BELISA method and HPLC method (P > 0.05). Above data verified that the BELISA techniques have good accuracy for the detection of SDZ in foods.
Strengths and advantages of the BELISA method
In this research, a BELISA method has been established for the determination of SDZ using Au@Pt@SiO2 nanozyme as a marker. The BELISA method is simple and no large equipments are required compared with conventional methods. Furthermore, the imprinted film is more stable than the biological antibody and can be reused. Therefore, the BELISA method has a low cost in detecting SDZ residues.
Conclusions
In this study, using a synthetic enzyme in place of a natural enzyme as a label, a rapid BELISA method to detect SDZ residues was invented. The assay investigated the stability and ease of preparation of these novel nanoparticles. The proposed method has potential applications in antibiotic residue analysis in agricultural and food products.
Ethical approval
This article does not contain any studies with animals performed by any of the authors.
Informed consent
Informed consent was obtained from all individual participants included in the study.
Supplemental Material
Download MS Word (237.5 KB)Disclosure statement
No potential conflict of interest was reported by the author(s).
Additional information
Funding
References
- Bi, X., & Liu, Z. (2014). Facile preparation of glycoprotein-imprinted 96-well microplates for enzyme-linked immunosorbent assay by boronate affinity-based oriented surface imprinting. Analytical Chemistry, 86(1), 959–966. doi: 10.1021/ac403736y
- Chu, J. S., Xu, Y., He, Q. F., Wang, L. H., & Lu, J. (2011). Determination of sulfadianzine residues in pork by direct competitive chemiluminescence enzyme immunoassay. Food Science, 32(10), 124–129.
- Danezis, G. P., Anagnostopoulos, C. J., Liapis, K., & Koupparis, M. A. (2016). Multi-residue analysis of pesticides, plant hormones, veterinary drugs and mycotoxins using hilic chromatography-ms/ms in various food matrices. Analytica Chimica Acta, 942, 121–138. doi: 10.1016/j.aca.2016.09.011
- Deng, H. H., Li, G. W., Hong, L., Liu, A. L., Chen, W., Lin, X. H., & Xia, X. H. (2014). Colorimetric sensor based on dual-functional gold nanoparticles: Analyte-recognition and peroxidase-like activity. Food Chemistry, 147, 257–261. doi: 10.1016/j.foodchem.2013.09.151
- Dognon, S. R., Degand, G., Douny, C., Delahaut, P., Igout, A., Dahouda, M., Youssao A. K. I., Scippo, M. L. (2018). The modified new two plates test for detecting tetracycline, beta-lactam, and sulfonamide antibiotic residues in kidney and muscle of cattle slaughtered in north-east Benin. Food Analytical Methods, 11, 3406–3416. doi: 10.1007/s12161-018-1322-y
- He, W., Liu, Y., Yuan, J., Yin, J. J., Wu, X., Hu, X., Zhang Ke, Liu Jianbo, Chen Chunying, Ji Yinglu, Guo Yuting, (2011). Au@pt nanostructures as oxidase and peroxidase mimetics for use in immunoassays. Biomaterials, 32(4), 1139–1147. doi: 10.1016/j.biomaterials.2010.09.040
- Hiba, A., Carine, A., Haifa, A. R., Ryszard, L., & Farouk, J. (2016). Monitoring of twenty-two sulfonamides in edible tissues: Investigation of new metabolites and their potential toxicity. Food Chemistry, 192, 212–227. doi: 10.1016/j.foodchem.2015.06.093
- Huang, L., Wu, Q., Wang, J., Foda, M., Liu, J., Cai, K., & Han, H. (2014). A brilliant sandwich type fluorescent nanostructure incorporating a compact quantum dot layer and versatile silica substrates. Chemical Communications, 50(22), 2896. doi: 10.1039/c3cc48405j
- Jansomboon, W., Boontanon, S. K., Boontanon, N., Polprasert, C., & Da, C. T. (2016). Monitoring and determination of sulfonamide antibiotics (sulfamethoxydiazine, sulfamethazine, sulfamethoxazole and sulfadiazine) in imported pangasius catfish products in Thailand using liquid chromatography coupled with tandem mass spectrometry. Food Chemistry, 212, 635–640. doi: 10.1016/j.foodchem.2016.06.026
- Jiang, W., Wang, Z., Beier, R. C., Jiang, H., Wu, Y., & Shen, J. (2013). Simultaneous determination of 13 fluoroquinolone and 22 sulfonamide residues in milk by a dual-colorimetric enzyme-linked immunosorbent assay. Analytical Chemistry, 85(4), 1995–1999. doi: 10.1021/ac303606h
- Jiang, M. D., Wu, S., Xu, L. H., Qiao, X. G., & Xu, Z. X. (2017). Determination of trichlorfon residues in vegetables using a quantum dot-labeled biomimetic immunoassay method followed by capillary electrophoresis. Food and Agricultural Immunology, 28(6), 1242–1255. doi: 10.1080/09540105.2017.1333579
- Khalili, H., Soudbakhsh, A., & Talasaz, A. H. (2011). Severe hepatotoxicity and probable hepatorenal syndrome associated with sulfadiazine. American Journal of Health-System Pharmacy, 68(10), 888–892. doi: 10.2146/ajhp100516
- Lata, K., Sharma, R., Naik, L., Raiput, Y. S., & Mann, B. (2015). Synthesis and application of cephalexin imprinted polymer for solid phase extraction in milk. Food Chemistry, 184, 176–182. doi: 10.1016/j.foodchem.2015.03.101
- Li, L., Lin, Z. Z., Peng, A. H., Zhong, H. P., Chen, X. M., & Huang, Z. Y. (2016). Biomimetic elisa detection of malachite green based on magnetic molecularly imprinted polymers. Food Chemistry, 1035, 25–30.
- Lin, Y., Ren, J., & Qu, X. (2014). Nano-gold as artificial enzymes: Hidden talents. Advanced Materials, 26(25), 4200–4217. doi: 10.1002/adma.201400238
- Liu, B., Ge, Y., Zhang, Y., Song, Y., Lv, Y., & Wang, X. X. (2012). Production of the class-specific antibody and development of direct competitive elisa for multi-residue detection of organophosphorus pesticides. Food and Agricultural Immunology, 23(2), 157–168. doi: 10.1080/09540105.2011.608120
- Mao, Y., Bao, Y., Han, D., Li, F., & Niu, L. (2012). Efficient one-pot synthesis of molecularly imprinted silica nanospheres embedded carbon dots for fluorescent dopamine optosensing. Biosensors & Bioelectronics, 38(1), 55–60. doi: 10.1016/j.bios.2012.04.043
- Robert, C., Brasseur, P. Y., Dubois, M., Delahaut, P., & Gillard, N. (2016). Development and validation of rapid multiresidue and multi-class analysis for antibiotics and anthelmintics in feed by ultra high performance liquid chromatography coupled to tandem mass spectrometry. Food Additives & Contaminants: Part A, 33(8), 1312–1323.
- Santos, L., & Ramos, F. (2016). Analytical strategies for the detection and quantification of antibiotic residues in aquaculture fishes: A review. Trends in Food Science & Technology, 52, 16–30. doi: 10.1016/j.tifs.2016.03.015
- Shah, J., Purohit, R., Singh, R., Karakoti, A. S., & Singh, S. (2015). Atp-enhanced peroxidase-like activity of gold nanoparticles. Journal of Colloid and Interface Science, 456, 100–107. doi: 10.1016/j.jcis.2015.06.015
- Shao, K., Wang, J., Jiang, X., Shao, F., Li, T., Ye, S., Chen Lu, Han, H. (2014). Stretch-stowage-growth strategy to fabricate tunable triply-amplified electrochemiluminescence immunosensor for ultrasensitive detection of pseudorabies virus antibody. Analytical Chemistry, 86(12), 5749–5757. doi: 10.1021/ac500175y
- Shen, Y. D., Deng, X. F., Xu, Z. L., Wang, Y., Lei, H. T., Wang, H., Yang J. Y., Xiao Z. L., Sun, Y. M. (2011). Simultaneous determination of malachite green, brilliant green and crystal violet in grass carp tissues by a broad-specificity indirect competitive enzyme-linked immunosorbent assay. Analytica Chimica Acta, 707(1-2), 0–154. doi: 10.1016/j.aca.2011.09.006
- Shi, Y., Peng, D. D., Shi, C. H., Zhang, X., Xie, Y. T., & Lu, B. (2011). Selective determination of trace 17β-estradiol in dairy and meat samples by molecularly imprinted solid-phase extraction and hplc. Food Chemistry, 126(4), 1916–1925. doi: 10.1016/j.foodchem.2010.12.020
- Song, Y., Wang, Y. Y., Zhang, Y., & Wang, S. (2011). Development of enzyme-linked immunosorbent assay for rapid determination of sildenafil in adulterated functional foods. Food and Agricultural Immunology, 23(4), 1–14.
- Sun, R., & Zhuang, H. (2015). Development of a highly sensitive biotin–streptavidin enzyme-linked immunosorbent assay for detecting diethyl phthalate based on a specific polyclonal antibody. Food and Agricultural Immunology, 26(5), 746–760. doi: 10.1080/09540105.2015.1027666
- Tang, Y., Gao, J., Liu, X., Gao, X., Ma, T., Lu, X., & Li, J. (2017a). Ultrasensitive detection of clenbuterol by a covalent imprinted polymer as a biomimetic antibody. Food Chemistry, 228, 62–69. doi: 10.1016/j.foodchem.2017.01.102
- Tang, Y., Zhang, Y., Zhang, H., Liu, X., Gao, X., & Lv, C. (2017b). Visual flow-through column biomimetic immunoassay using molecularly imprinted polymer as artificial antibody for rapid detection of clenbuterol in water sample. Food and Agricultural Immunology, 28(52), 949–957. doi: 10.1080/09540105.2017.1320359
- Vosough, M., Onilghi, M. N., & Salemi, A. (2016). Optimization of matrix solid-phase dispersion coupled with high performance liquid chromatography for determination of selected antibiotics in municipal sewage sludge. Analytical Methods, 8(24), 4853–4860. doi: 10.1039/C6AY00112B
- Wang, S. M., Jiang, M. D., Ju, Z. L., Qiao, X. G., & Xu, Z. X. (2017). A flow-injection chemiluminescent biomimetic immunoassay method using a molecularly imprinted polymer as a biomimetic antibody for the determination of trichlorfon. Food and Agricultural Immunology, 29(2), 159–170.
- Wiester, M. J., Ulmann, P. A., & Mirkin, C. A. (2011). Enzyme mimics based upon supramolecular coordination chemistry. Angewandte Chemie International Edition, 50(1), 114–137. doi: 10.1002/anie.201000380
- Yamada, Y., Tsung, C. K., Huang, W., Huo, Z., Habas, S. E., Soejima, T., Aliaga C. E, Somorjai G. A., Yang, P. (2011). Nature Chemistry, 3(5), 372–376. doi: 10.1038/nchem.1018
- Zhang, C., Cui, H., Han, Y., Yu, F., & Shi, X. (2017). Development of a biomimetic enzyme-linked immunosorbent assay based on molecularly imprinted polymers on paper for the detection of carbaryl. Food Chemistry, 240, 893–897. doi: 10.1016/j.foodchem.2017.07.109