ABSTRACT
Polysaccharide from Gynostemma pentaphyllum Makino (GPP) could prevent H22 tumour growth and have immunostimulatory in mice, but the mechanism is largely unknown. In this study, we further investigated whether GPP prevents tumour growth by inducing cytotoxicity to the tumour cells or enhancing immunity in H22 ascites tumour-bearing mice. The results showed that GPP prevented the H22 ascites tumour growth in vivo, but showed little effect on cell viability and cycles to H22 cells in vitro. GPP improved the proportion and mitochondrial level of T cells, and promoted the secretion of IL-2 and IFN-γ in the ascites of the mice. Furthermore, GPP in vitro enhanced the cell viability and promoted activation (in particular T cells), proliferation, and the secretion of IL-2 and IFN-γ of lymphocyte. Taken together, our results demonstrated that GPP prevents H22 ascites tumour growth by enhancing immunity rather than cytotoxicity in mice.
1. Introduction
Gynostemma pentaphyllum (Thunb.) Makino (Jiaogulan) is an edible traditional Chinese medicine and has been traditionally used as a popular herbal tea in China and many other countries for its wide bioactivities (Chen et al., Citation2011; Li et al., Citation2016; Ren et al., Citation2019). Polysaccharides have been demonstrated to be partially responsible for some bioactivity of G. pentaphyllum. Previous studies showed that G. Pentaphyllum Polysaccharides (GPP) has multiple biological activities and function, such as anti-tumour activities (Li et al., Citation2012; Liu et al., Citation2014), immunostimulatory (Ren et al., Citation2019; Shang et al., Citation2016), antioxidant activities (Niu et al., Citation2014; Shang et al., Citation2016) and neuroprotective effect (Deng & Yang, Citation2014; Keilhoff et al., Citation2017). The anti-tumour activity of GPP had been demonstrated in the tumour-bearing mice that it could prevent the growth of melanoma B16 (Li et al., Citation2012) and hepatoma H22 in vivo (Liu et al., Citation2014). Furthermore, GPP could enhance the immunity of the tumour-bearing mice by improving the level of serum TNF-α, IL-2, IL-12 and IFN-γ, increasing the activity of natural killer (NK) cells and cytotoxic T lymphocytes (Li et al., Citation2012; Liu et al., Citation2014). However, the mechanism for GPP preventing tumour growth in mice is largely unknown.
It is well known that anti-tumour immunity plays key roles in preventing the occurrence and development of tumours. This function is called immune surveillance, which is involved by a variety of immune cells and cytokines, including T cells and their secreted cytokines, such as IL-2 and IFN-γ (Ribatti, Citation2017). T cells, particularly cytotoxic T lymphocytes, are an important part of anti-tumour immunity (Garrido et al., Citation2017). However, the tumour-infiltrating T cells are often exhausted (Jiang, Li, & Zhu, Citation2015) due to the decrease in mitochondrial biosynthesis and dysfunctional metabolism (Bengsch et al., Citation2016; Scharping et al., Citation2016). In this case, enhancing T cell anti-tumour immunity by sustaining metabolic fitness and longevity is one of the most promising methods for cancer immunotherapy (O'Sullivan, Sanin, Pearce, & Pearce, Citation2019; Rivadeneira & Delgoffe, Citation2018; Siska & Rathmell, Citation2015).
Though previous studies had reported that GPP could inhibit H22 tumour growth and enhance the immunity in mice, but the mechanism is largely unknown. In the present study, we further investigated whether GPP prevents tumour growth by inducing cytotoxicity to the tumour cells or enhancing immunity in H22 ascites tumour-bearing mice.
2. Materials and methods
2.1. Materials
GPP was prepared from G. Pentaphyllum as described previously (Liu et al., Citation2014) and the total sugar was about 85%. FITC-conjugated anti-mouse CD3 antibody, PE-conjugated anti-mouse CD69 antibody and carboxyfluorescein diacetate succinimidyl ester (CFDA-SE) were purchased from Affinity Bioscience. Propidium Iodide (PI) was purchased from Sigma. Mouse IL-2 and Mouse IFN-γ CBA kit were purchased from BD Biosciences. MitoTracker Deep Red FM kit was purchased from Thermo Fisher Scientific.
2.2. Animals
Specific pathogen-free (SPF) 6–8-week-old male BALB/c mice, weighed 18–22g, were purchased from the Guangdong Medical Laboratory Animal Center (Foshan, China). All animals were kept under SPF condition and fed with standard laboratory diet and water. The experimental procedures were approved by the Animal Care and Use Committee of Guangzhou University of Chinese Medicine, Guangzhou, China.
2.3. Preparation of tumour-bearing mice and treatment protocol
The mice were divided into control, model and GPP treatment group. The tumour-free mice were used as control. H22 ascites tumour-bearing mice were used in the model group and GPP treatment group and prepared by intraperitoneal injection with H22 cells (2 × 106 cells/mouse). After tumour challenge for 5 days, the mice in the GPP treatment group were intragastrically administrated once daily with 200 mg/kg GPP for 10 days according to the reference (Liu et al., Citation2014). The mice in the control and model group were intragastrically administrated with the same volume of saline solution.
2.4. Analysis of ascites volumes, thymus and spleen index
The mice were sacrificed by cervical dislocation on the 2nd day after the last administration. The ascites fluid was collected and measured via syringe according to the reference (Zhang et al., Citation2018). After centrifugation (300 × g for 5 min), the supernate of ascites was collected for the cytokine dectection. The thymus and spleen were excised and weighed. The immune organ index was calculated using the formula: thymus or spleen index (mg/g) = thymus or spleen weight (mg)/body weight (g).
2.5. Analysis of the proportion and mitochondrial biosynthesis of T cells in the ascites
The ascites were centrifuged at 300 × g for 5 min at 4°C after the volume was recorded. The supernatant was collected for further analysis of the levels of IL-2 and IFN-γ, while the cells were washed twice and resuspended in PBS. After being stained with FITC-conjugated anti-mouse CD3 antibody and MitoTracker Deep Red FM according to the manufacturer’s instructions, the cells were detected using a FACS Canto™ II flow cytometer (BD Biosciences).
2.6. Effect of GPP on the viability of h22 cells and lymphocytes in vitro
The cell viability was measured by the MTT assay as described previously (Deng et al., Citation2017). Briefly, to determine the effect of GPP on the viability of H22 cells, the cells were seeded in 96-well plates (1 × 104 cells/well) and treated with GPP (0, 125, 250, 500, and 100 μg/mL) for 24, 48 and 72 h. To determine the effect of GPP on the lymphocyte viability, splenocytes were prepared as described previously (Deng et al., Citation2017) and seeded in 96-well plates (4 × 105 cells/well), and then treated with GPP (0, 200, 400, and 800 μg/mL) for 48 h. After the cells were treated with DMSO, the absorbance at 570 and 630 nm was measured using a microplate reader.
2.7. Effect of GPP on lymphocyte activation in vitro
Splenocytes were treated with GPP (0, 200, 400, and 800 μg/mL) for 24 h and the cells stimulated with ConA (5 μg/mL) were used as a positive control. After centrifugation at 300 × g for 5 min at 4°C, the supernatants were collected for further cytokine analysis, while the cells were washed twice and resuspended in PBS. Then the cells were stained with FITC-conjugated anti-mouse CD3 antibody and PE-conjugated anti-mouse CD69 antibody according to the manufacturer’s instructions. Finally, the cells were measured using a FACS Canto™ II flow cytometer (BD Biosciences).
2.8. Effect of GPP on the lymphocyte proliferation in vitro
The effect of GPP on lymphocyte proliferation was determined by carboxyfluorescein succinimidyl ester (CFSE) labelling. The CFSE labelling was performed as described previously (Quah & Parish, Citation2010). Briefly, splenocytes were co-incubation with CFDA-SE dye (5 μM) for 5 min at room temperature; in this period, CFDA-SE converted to CFSE. The cells were washed twice with PBS containing 5% FCS and treated with GPP (0, 200, 400, and 800 μg/mL) for 72 h before being determined by flow cytometry.
2.9. Effects of GPP on the cell cycles of lymphocytes and h22 cells
To investigate the effect of GPP on the cell cycles of lymphocytes and H22 cells, splenocytes were treated with GPP (0, 200, 400, and 800 μg/mL) for 48 h, while H22 cells were treated with GPP (0, 250, 500, and 1000 μg/mL) for 72 h. And then the cells were analysed by flow cytometry with PI staining as described previously (Deng et al., Citation2017).
2.10. Analysis of the cytokines levels
The levels of IL-2 and IFN-γ in the previously collected supernatants of ascites and cell culture medium were determined by CBA kits and using a FACS Canto™ II flow cytometer (BD Biosciences) according to the manufacturer’s instructions.
2.11. Statistical analysis
The data were shown as Mean ± SEM. Unpaired Student’s t-test or ANOVA was used to assess the statistical significance of differences between experimental groups. P value less than 0.05 was considered statistically significant differences.
3. Results and discussion
3.1. GPP prevents h22 ascites tumour growth in mice independent of cytotoxicity
Previous studies had reported that GPP could prevent H22 solid tumour growth and greatly prolonged the survival period in H22 ascites tumour in mice (Liu et al., Citation2014). In the present study, we further investigated the mechanism of GPP in preventing the tumour growth. The ascites tumour mice were prepared by injecting with H22 cells into the abdominal cavity as previously mentioned. The tumour-free mice were used as a control. As shown in (A), the ascites volumes of the mice in the GPP group were significantly lower when compared to those of the model group (P < 0.05). The result demonstrated that GPP inhibited H22 ascites tumour growth in the present study, which was consistent with the previous study (Liu et al., Citation2014).
Figure 1. Effects of GPP on the growth of H22 ascites tumour in mice and H22 cells in vitro. (A) Ascites tumour volumes from BALB/c mice in the model group and GPP treatment group (200 mg/kg). (B) Cell viability of H22 cells. The cells were treated with 0, 125, 250, 500 and 1000 μg/mL of GPP for 24, 48 and 72 h. The cell viability was detected by MTT assay. (C) Statistical analysis of the cell-cycle progress in H22 cells after the cells were treated with GPP for 72 h. (D) Representative flow cytometry histogram of cell-cycle progression in H22 cells. Data are represented as Mean ± SEM (n = 8 in vivo, n = 5 in vitro), *P < 0.05 versus model group.
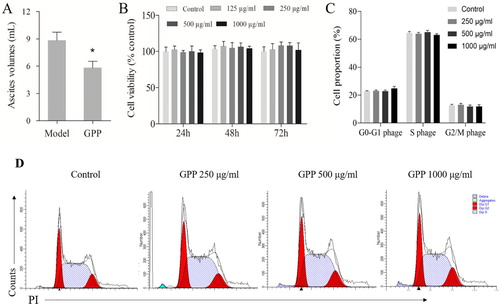
As known, chemotherapy drugs prevent tumour growth by inducing cytotoxicity to tumour cells. To investigate whether GPP prevented H22 ascites tumour growth also by cytotoxicity, H22 cells were treated with GPP in vitro. Cell viability and cycles were determined by MTT assay and flow cytometry, respectively. As shown in (B), the cell viability of H22 cells in GPP treatment groups (125, 250, 500 and 1000 μg/mL) had no significant difference in comparison to those of the control group after GPP treatment for 24, 48 and 72 h. Also, the cell cycles of H22 cells in GPP treatment groups (250, 500 and 1000 μg/mL) had no significant difference in comparison to those of the control group as shown in (C,D). The results indicated that GPP prevented H22 ascites tumour growth in mice independent of cytotoxicity.
3.2. GPP improves the proportion and mitochondrial biosynthesis of T cells in h22 ascites tumour
Since GPP showed little cytotoxicity effect on the growth of H22 cells in vitro in the present study, we speculated that the prevention effect of GPP on H22 ascites tumour growth depended on boosting immunity. Evidence supported our conclusion is that GPP could enhance the immunity in the tumour-bearing mice and immunosuppressed mice, such as improved the immune organ index and increased the activity of cytotoxic T lymphocytes as well as elevated CD4+ T lymphocyte counts (Liu et al., Citation2014; Shang et al., Citation2016). To test our hypothesis in the present study, the indexes for thymus and spleen, the proportion and mitochondrial biosynthesis of T cells in H22 ascites tumour were investigated.
The thymus and spleen are two important immune organs and play critical roles in anti-tumour defense (Zhang et al., Citation2018). In the present study, as shown in (A,B), the thymus and spleen indexes in the model group were significantly lower than those in the control group (both P < 0.01). This indicated that the tumour-bearing mice were under the immunosuppressive condition. The results also showed that those indexes in GPP group were significantly higher than those in the model group (P < 0.05), which indicated that GPP relieved immunosuppression in the tumour-bearing mice.
Figure 2. Effects of GPP on immune organ index, proportion and mitochondrial biosynthesis of T cells in H22 ascites tumour-bearing mice. (A) Thymus index. (B) Spleen index. (C) Representative dot plots of T cells percentage gated on abdominal cavity cells. The percentage of T cells in abdominal cavity from tumour-free mice or H22 ascites tumour-bearing mice was analysed by flow cytometry. (D) Statistical analysis of the percentage of T cells in abdominal cavity cells. (E) Representative histograms of mitochondrial biosynthesis level gated T cells. The mitochondrial biosynthesis level was represented as mean fluorescence intensity (MFI) of Mito FM and was analysed by flow cytometry. Data are represented as Mean ± SEM, n = 8 in each group. *P < 0.05 and **P < 0.01 versus model group.
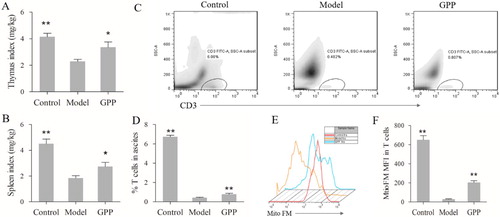
T cells are an important part of anti-tumour immunity, studies have reported that the infiltration of T cells in cancer patients is a favourable prognostic factor (Hiraoka et al., Citation2006; Zengin, Citation2019). Though the effect of GPP on the activity of cytotoxic T lymphocytes had been reported in H22 tumour-bearing mice, the mechanism is largely unknown. To uncover it, we firstly investigated the effect of GPP on the infiltration of T cells in the ascites in the present study. As shown in (C,D), the proportion of T cells in the ascites of the model group was significantly lower than those in the control group (P < 0.01); while GPP group had a higher proportion of T cells than the model group (P < 0.01). The results indicated that GPP treatment promoted the infiltration of T cells in the ascites. However, accumulated evidence has shown that T cells in the tumour microenvironment are exhausted, which dues to the metabolic alterations and bioenergetic insufficiencies (Scharping et al., Citation2016; Zhang & Romero, Citation2018). Also, our previous study demonstrated that the expression of PD-1, an exhaustion phenotype of T cells, in tumour tissues of H22 tumour-bearing mice was remarkably increased (Deng et al., Citation2018). Thus, we further investigated whether GPP could improve the mitochondrial biosynthesis of T cells in H22 ascites tumour. The mitochondrial biosynthesis in T cells was measured with Mito FM reagent according to the reference (Scharping et al., Citation2016). As shown in (E,F), the Mito FM fluorescence of T cells in the model group was dramatically decreased when compared to those in the control group (P < 0.01); while the Mito FM fluorescence of T cells in GPP group was remarkably increase than those in the model group (P < 0.01). The results indicated that GPP could improve the mitochondrial biosynthesis of T cells in H22 ascites tumour. This provides evidence for GPP enhancing the killing activity of lymphocytes (mainly T cells) in H22 tumour-bearing mice partly by improving the mitochondrial biosynthesis as described in the present study (Figure S1 in supplementary material) and in the previous studies (Liu et al., Citation2014).
3.3. GPP increases the secretion of IL-2 and IFN-γ in tumour-bearing mice
Some cytokines play important roles in anti-tumour immune response and several have been used for cancer therapy (Floros & Tarhini, Citation2015; Shourian, Beltra, Bourdin, & Decaluwe, Citation2019). For example, IL-2 is one of the T cell growth factor (Choudhry et al., Citation2018), while IFN-γ can enhance tumour cell recognition by cytotoxic effector T cells (Shourian et al., Citation2019). In the present study, we investigated the effect of GPP on the production of IL-2 and IFN-γ in ascites of the tumour-bearing mice. As shown in , mice in the GPP group had higher levels of IL-2 and IFN-γ in the ascites than those in the model group (P < 0.05 or P < 0.01). The results were similar to the previous report in which polysaccharides from G. pentaphyllum Makino increased the levels of serum IL-2 and IFN-γ in H22 solid tumour-bearing mice and melanoma B16 tumour-bearing mice (Li et al., Citation2012; Liu et al., Citation2014).
Figure 3. GPP treatment promoted the secretion of IL-2 and IFN-γ in the ascites of H22 tumour-bearing mice. The cytokines were investigated by CBA kits and measured by flow cytometry. (A) The level of IL-2 in ascites. (B) The level of IFN-γ in the ascites. Data are represented as Mean ± SEM, n = 8 in each group. *P < 0.05 and **P < 0.01 versus model group in the mice.
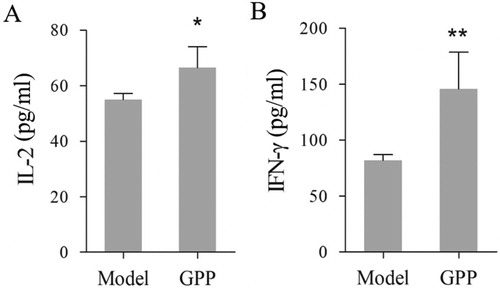
3.4. GPP stimulates the activation and proliferation of lymphocytes in vitro
Lymphocytes, especially the T lymphocytes, play important roles in anti-tumour immunity. After encountering antigens as well as other activating stimuli, T lymphocytes would produce several biological responses, such as activation, proliferation, differentiation into effector and memory subsets (Smith-Garvin, Koretzky, & Jordan, Citation2009; Van Parijs & Abbas, Citation1998; Williams & Bevan, Citation2007). Based on the results as shown above in the present study, we speculated that GPP prevented H22 ascites tumour growth in mice by enhancing anti-tumour immunity. To make the speculation more convincing and uncover the mechanism of how GPP influenced the T lymphocyte function, we further investigated the effect of GPP on the activation and proliferation of lymphocytes in vitro.
We firstly investigated the effect of GPP on cell viability and activation of lymphocytes by MTT assay and flow cytometry, respectively. As shown in (A), the cell viability of lymphocytes in GPP groups (200, 400 and 800 μg/mL) was higher significantly than those in the control group (all P < 0.01). Furthermore, the flow cytometry results showed that CD69 expression levels of lymphocytes and T cells in GPP groups were higher significantly than those in the control group (all P < 0.01, (B–E)). Since CD69 is one of the early activation markers for lymphocytes (Cibrian & Sanchez-Madrid, Citation2017), the results demonstrated that GPP could stimulate the activation of lymphocytes, including T lymphocytes.
Figure 4. Effect of GPP on cell viability and activation of lymphocytes in vitro. (A) Cell viability of lymphocytes was measured by MTT assay. (B) Representative histograms of CD69+ cells gated on lymphocytes. (C) Statistical analysis of the percentage of CD69 + lymphocytes. (D) Representative histograms of CD69+ T cells gated on CD3+ lymphocytes. (E) Statistical analysis of the percentage of CD69 + T cells. Data are represented as Mean ± SEM, n = 5 in each group. **P < 0.01 versus control group.
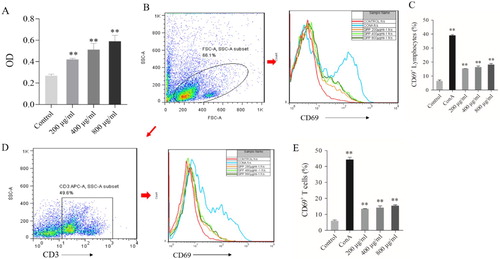
We next investigated whether GPP could also stimulate the proliferation of lymphocytes in vitro. As shown in (A), the numbers of lymphocyte colonies in GPP groups were more than those in the control group, which indicated the promotion effect of GPP on lymphocyte proliferation. To confirm it, we used the CFSE labelling to detect the percentages of dividing lymphocytes after stimulation for 72 h. As shown in (B,C), the percentages of dividing lymphocytes in GPP groups were significantly higher than those in the control group (all P < 0.01). In consistent with these results, the cell-cycle analysis results ((D,E)) showed that the percentages of cells stepped into S phase and G2/M phase in GPP group were higher than those in the control group. These results demonstrated that GPP could stimulate the proliferation of lymphocytes in vitro.
Figure 5. Effects of GPP on the proliferation and cell-cycle of lymphocytes in vitro. The lymphocytes were stimulated with 0, 200, 400 and 800 μg/mL of GPP for 72 h. (A) Lymphocyte colony was observed in optical microscopy with 100× magnification. (B) Representative flow cytometry histogram overlay plots of CFSE in lymphocytes. (C) Statistical analysis of the percentage of dividing lymphocytes. (D) Representative flow cytometry histogram of cell-cycle progression in lymphocytes. (E) Statistical analysis of the cell-cycle progression in lymphocytes. Data are represented as Mean ± SEM, n = 5 in each group. **P < 0.05 and **P < 0.01 versus control group.
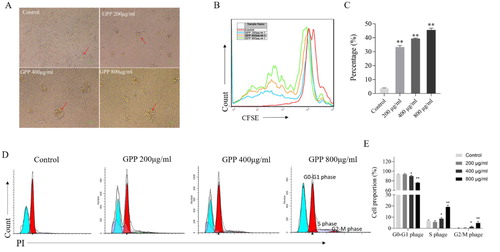
3.5. GPP stimulates lymphocytes to secrete IL-2 and IFN-γ in vitro
Cytokine secretion is also one of the biological responses for lymphocytes after activation. To further uncover the mechanism of GPP increased the secretion of IL-2 and IFN-γ in tumour-bearing mice, we investigated the effect of GPP on the secretion of IL-2 and IFN-γ in lymphocytes in vitro. As shown in , GPP at the dose of 400 and 800 μg/mL could stimulate lymphocytes to secrete IL-2 significantly (P < 0.05 and P < 0.01, respectively); while GPP at the dose of 200, 400 and 800 μg/mL could stimulate the secretion of IFN-γ significantly (all P < 0.01). The results provided strong evidence to illustrate one possible mechanism for GPP increased the secretion of IL-2 and IFN-γ in tumour-bearing mice.
Figure 6. Effects of GPP on the secretion of IL-2 and IFN-γ in lymphocytes in vitro. The lymphocytes were stimulated with 0, 200, 400 and 800 μg/mL of GPP and 5 μg/mL of ConA for 24 h. The cytokines were investigated by CBA kits and measured by flow cytometry. (A) The level of IL-2 in cell culture supernatant. (B) The level of IFN-γ in cell culture supernatant. Data are represented as Mean ± SEM, n = 5 in each group. *P < 0.05 and **P < 0.01 versus control group.
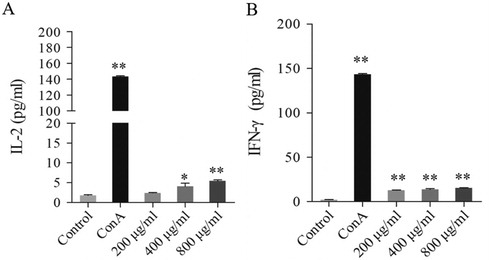
4. Conclusion
In the present study, our results uncovered the mechanism of GPP prevented the H22 tumour growth in the mice (). The results showed that GPP prevented H22 ascites tumour growth in mice independent of cytotoxicity. Furthermore, the results showed that GPP not only improved the proportion and mitochondrial level of T cells, but also promoted the secretion of IL-2 and IFN-γ in the ascites of the mice. These could be supported by the in vitro results that GPP showed promotion effects in vitro on lymphocytes viability, activation (in particular T cells), proliferation, and the secretion of IL-2 and IFN-γ. Taken together, these results demonstrated that GPP prevents H22 ascites tumour growth by enhancing immunity rather than cytotoxicity in mice.
Figure 7. The mechanism that GPP prevents H22 tumour growth by enhancing immunity. In the tumour microenvironment, effector T cells became exhaustion, which dues to the metabolic alterations and bioenergetic insufficiencies. GPP improved the mitochondrial biosynthesis of T cells, promoted the secretion of IL-2 and IFN-γ, which contributed to enhance the tumour cell killing activity.
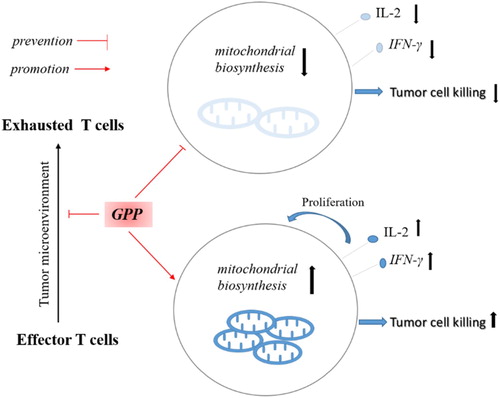
Supplemental Material
Download MS Word (630.9 KB)Disclosure statement
No potential conflict of interest was reported by the author(s).
Additional information
Funding
References
- Bengsch, B., Johnson, A. L., Kurachi, M., et al. (2016). Bioenergetic insufficiencies due to metabolic alterations regulated by the inhibitory receptor PD-1 are an early driver of CD8+ T cell exhaustion. Immunity, 45(2), 358–373. doi: 10.1016/j.immuni.2016.07.008
- Chen, T., Li, B., Li, Y., et al. (2011). Catalytic synthesis and antitumor activities of sulfated polysaccharide from Gynostemma pentaphyllum Makino. Carbohydrate Polymers, 83(2), 554–560. doi: 10.1016/j.carbpol.2010.08.024
- Choudhry, H., Helmi, N., Abdulaal, W. H., et al. (2018). Prospects of IL-2 in cancer immunotherapy. Biomed Research International, 2018, 9056173. doi: 10.1155/2018/9056173
- Cibrian, D., & Sanchez-Madrid, F. (2017). CD69: from activation marker to metabolic gatekeeper. European Journal of Immunology, 47(6), 946–953. doi: 10.1002/eji.201646837
- Deng, X., Li, X., Luo, S., et al. (2017). Antitumor activity of Lycium barbarum polysaccharides with different molecular weights: An in vitro and in vivo study. Food & Nutrition Research, 61(1), 1399770. doi: 10.1080/16546628.2017.1399770
- Deng, X., Luo, S., Luo, X., et al. (2018). Polysaccharides from Chinese herbal Lycium barbarum induced systemic and local immune responses in H22 tumor-bearing mice. Journal of Immunology Research, 3431782. doi: 10.1155/2018/3431782
- Deng, Q., & Yang, X. (2014). Protective effects of Gynostemma pentaphyllum polysaccharides on PC12 cells impaired by MPP+. International Journal of Biological Macromolecules, 69, 171–175. doi: 10.1016/j.ijbiomac.2014.05.049
- Floros, T., & Tarhini, A. A. (2015). Anticancer cytokines: Biology and clinical effects of interferon-alpha2, interleukin (IL)-2, IL-15, IL-21, and IL-12. Seminars in Oncology, 42(4), 539–548. doi: 10.1053/j.seminoncol.2015.05.015
- Garrido, F., Perea, F., Bernal, M., et al. (2017). The escape of cancer from T cell-mediated immune surveillance: HLA class I loss and tumor tissue architecture. Vaccines, 5, 71. doi: 10.3390/vaccines5010007
- Hiraoka, K., Miyamoto, M., Cho, Y., et al. (2006). Concurrent infiltration by CD8+ T cells and CD4+ T cells is a favourable prognostic factor in non-small-cell lung carcinoma. British Journal of Cancer, 94(2), 275–280. doi: 10.1038/sj.bjc.6602934
- Jiang, Y., Li, Y., & Zhu, B. (2015). T-cell exhaustion in the tumor microenvironment. Cell Death & Disease, 6, e1792. doi: 10.1038/cddis.2015.162
- Keilhoff, G., Esser, T., Titze, M., et al. (2017). Gynostemma pentaphyllum is neuroprotective in a rat model of cardiopulmonary resuscitation. Experimental and Therapeutic Medicine, 14(6), 6034–6046. doi: 10.3892/etm.2017.5315
- Li, Y., Lin, W., Huang, J., et al. (2016). Anti-cancer effects of Gynostemma pentaphyllum (Thunb.) Makino (Jiaogulan). China Medicine, 11, 43. doi: 10.1186/s13020-016-0114-9
- Li, X. L., Wang, Z. H., Zhao, Y. X., et al. (2012). Isolation and antitumor activities of acidic polysaccharide from Gynostemma pentaphyllum Makino. Carbohydrate Polymers, 89(3), 942–947. doi: 10.1016/j.carbpol.2012.04.040
- Liu, J., Zhang, L., Ren, Y., et al. (2014). Anticancer and immunoregulatory activity of Gynostemma pentaphyllum polysaccharides in H22 tumor-bearing mice. International Journal of Biological Macromolecules, 69, 1–4. doi: 10.1016/j.ijbiomac.2014.05.014
- Niu, Y., Shang, P., Chen, L., et al. (2014). Characterization of a novel alkali-soluble heteropolysaccharide from tetraploid Gynostemma pentaphyllum Makino and its potential anti-inflammatory and antioxidant properties. Journal of Agricultural and Food Chemistry, 62(17), 3783–3790. doi: 10.1021/jf500438s
- O'Sullivan, D., Sanin, D. E., Pearce, E. J., & Pearce, E. L. (2019). Metabolic interventions in the immune response to cancer. Nature Reviews Immunology, 19(5), 324–335. doi: 10.1038/s41577-019-0140-9
- Quah, B. J., & Parish, C. R. (2010). The use of carboxyfluorescein diacetate succinimidyl ester (CFSE) to monitor lymphocyte proliferation. Journal of Visualized Experiments, 44, e2259. doi: 10.3791/2259
- Ren, D., Zhao, Y., Zheng, Q., et al. (2019). Immunomodulatory effects of an acidic polysaccharide fraction from herbal Gynostemma pentaphyllum tea in RAW264.7 cells. Food & Function, 10(4), 2186–2197. doi: 10.1039/c9fo00219g
- Ribatti, D. (2017). The concept of immune surveillance against tumors. The first theories. Oncotarget, 8(4), 7175–7180. doi: 10.18632/oncotarget.12739
- Rivadeneira, D. B., & Delgoffe, G. M. (2018). Antitumor T-cell reconditioning: Improving metabolic fitness for optimal cancer immunotherapy. Clinical Cancer Research, 24(11), 2473–2481. doi: 10.1158/1078-0432.CCR-17-0894
- Scharping, N. E., Menk, A. V., Moreci, R. S., et al. (2016). The tumor microenvironment represses T cell mitochondrial biogenesis to drive intratumoral T cell metabolic Insufficiency and dysfunction. Immunity, 45(3), 701–703. doi: 10.1016/j.immuni.2016.08.009
- Shang, X., Chao, Y., Zhang, Y., et al. (2016). Immunomodulatory and antioxidant effects of polysaccharides from Gynostemma pentaphyllum Makino in immunosuppressed mice. Molecules, 21, 8. doi: 10.3390/molecules21081085
- Shourian, M., Beltra, J. C., Bourdin, B., & Decaluwe, H. (2019). Common gamma chain cytokines and CD8 T cells in cancer. Seminars in Immunology, 42, 101307. doi: 10.1016/j.smim.2019.101307
- Siska, P. J., & Rathmell, J. C. (2015). T cell metabolic fitness in antitumor immunity. Trends in Immunology, 36(4), 257–264. doi: 10.1016/j.it.2015.02.007
- Smith-Garvin, J. E., Koretzky, G. A., & Jordan, M. S. (2009). T cell activation. Annual Review of Immunology, 27, 591–619. doi: 10.1146/annurev.immunol.021908.132706
- Van Parijs, L., & Abbas, A. K. (1998). Homeostasis and self-tolerance in the immune system: Turning lymphocytes off. Science, 280(5361), 243–248. doi: 10.1126/science.280.5361.243
- Williams, M. A., & Bevan, M. J. (2007). Effector and memory CTL differentiation. Annual Review of Immunology, 25, 171–192. doi: 10.1146/annurev.immunol.25.022106.141548
- Zengin, M. (2019). Prognostic role of tumour-infiltrating T lymphocytes in stage IIA (T3N0) colon cancer: A broad methodological study in a fairly homogeneous population. Annals of Diagnostic Pathology, 41, 69–78. doi: 10.1016/j.anndiagpath.2019.05.007
- Zhang, Z., Luo, D., Xie, J., et al. (2018). Octahydrocurcumin, a final hydrogenated metabolite of curcumin, possesses superior anti-tumor activity through induction of cellular apoptosis. Food & Function, 9(4), 2005–2014. doi: 10.1039/c7fo02048a
- Zhang, L., & Romero, P. (2018). Metabolic control of CD8 + T cell fate decisions and antitumor immunity. Trends in Molecular Medicine, 24(1), 30–48. doi: 10.1016/j.molmed.2017.11.005