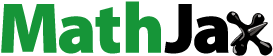
ABSTRACT
Green chicory (Cichorium intybus) leaf is consumed worldwide that has tremendous bio-functional activities. This study was designed to elucidate the bio-functional activities of the green chicory leaf extract (CLE). Trolox equivalent antioxidant activities were carried out against DPPH, ABTS radical scavenging and ferric reducing activities. CLE showed remarkable hepatoprotective activity against oxidative damages and reactive oxygen species reducing effect in RAW 264.7 and Hep G2 cells. The anti-inflammatory effects performed on LPS-induced RAW 264.7 cells and primary spleen cells. In RAW 264.7 cells, Chicory leaf extract significantly restored the iNOS, COX-2, IL-6, TNF-α and other pro-inflammatory cytokines in mRNA expression. Meanwhile, it restored NF-κB (P-65) and MAPK pathways activation in immunoblot assay dose-dependently and repressed the P-65 translocation in the nucleus. Moreover, it showed notable anti-proliferative activities and downregulated NF-κB (P-65) and MAPK activation in LPS-stimulated splenocytes. Our findings suggest that CLE could be used as a natural anti-inflammatory agent.
GRAPHICAL ABSTRACT
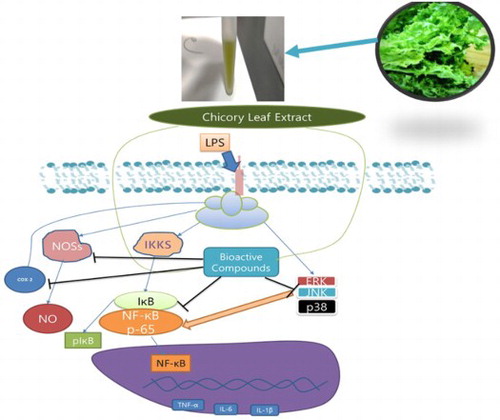
1. Introduction
Cichorium intybus L. (Chicory) is widely cultivated in Europe, Asia, North America and it is also used as a medicinal plant in Italy and Greece (Montefusco et al., Citation2015). Chicory plant is a part of Asteraceae family and its leaves are traditionally consumed as salad and cooked vegetables in Mediterranean cuisines (Jancic et al., Citation2017). Previous reports suggested chicory has anti-toxic, hypoglycaemic activities and many other pharmacological effects that established the chicory as a functional food (Azzini et al., Citation2016; Sahan, Gurbuz, Guldas, Degirmencioglu, & Begenirbas, Citation2017). It has also been commercialized as beverages for its tremendous health benefits. However, green chicory leaves are a vast source of phytochemicals, which are secondary metabolites that have enormous effect as a functional food. Besides, chicory root is widely used as functional food components as well in various ways (Sinkovič et al., Citation2015). Natural functional foods/components previously showed remarkable effects against inflammatory diseases (Ghosh et al., Citation2018; Hasnat, Pervin, Cha, Kim, & Lim, Citation2015; Miao et al., Citation2019; Pervin et al., Citation2016). Chicory leaves are cost-effective with many health benefits and may consume raw in salad and there are many varieties of chicory are available. Green chicory leaf is not only reported as a rich source of polyphenols but also highly bioaccessible which has a great impact on bio functionalities. The single 100 gram of chicory may supply up to 400 mg polyphenol to the human diet (Sinkovič, Hribar, & Vidrih, Citation2014). To the best of our knowledge, this the first-time study to understanding the anti-inflammatory activities of a Chicory leaf.
Living organisms generate reactive oxygen species (ROS) and other free radicals during physiological processes. Though ROSs have many key roles in normal physiological function including metabolism, respiration and so on. Meanwhile, excessive ROS also plays a vital role to trigger inflammation that incurs inflammatory diseases, gastric disorders like gastric adenocarcinoma, osteoporosis, liver diseases and so on (Graziani et al., Citation2005; Jeong et al., Citation2018). Excessive free radicals result in oxidative damage to biomolecules, impair the function of protein, trigger cell death, and cause tissue damage leading to chronic and lethal diseases. The tumorigenic characteristics of ROS have prompted the evaluation of dietary antioxidants as potential preventive and therapeutic effects on animals and humans (Chandel & Tuveson, Citation2014). Hep G2 cells widely studied for evaluating hepatoprotective activity (Jeong et al., Citation2018). Protective activity of liver cells against oxidative damages is relevant to the effect against liver diseases. Excessive free radicals and overwhelmed cellular ROS production imbalance the physiological homeostasis system that may incur chronic diseases including cardiovascular disease (CVD), diabetes, cancer and premature aging. Besides, oxidative stress triggers an adverse inflammatory response which causes chronic inflammatory diseases. Green chicory leaf extract (CLE) is an enormous source of polyphenols that have also been previously (Nwafor, Shale, & Achilonu, Citation2017) reported as the phytochemical constituents of this plant.
Inflammation is an inevitable and essential cellular event in response to infection, antigen in the living organisms. The inflammation is also recognized as a hallmark of many chronic diseases, such as cancer, obesity, diabetes, arthritis, cardiac diseases, IBD, Parkinson disease (PD) and so on (Al Mijan & Lim, Citation2018; Chen et al., Citation2018; Ghosh et al., Citation2018; Miao et al., Citation2019; Tilg, Zmora, Adolph, & Elinav, Citation2020) when it goes aversively. Molecular mechanisms of inflammation are still complex and not fully understood. CLE is rich in polyphenols that have high potentialities to restore pro-inflammatory cytokines and mediators and act as an inflammatory regulator. Inflammation is the response of immune cells (e.g. macrophages, neutrophils, B cells and so on). RAW 264.7 cell line (murine macrophage) is well established and extensively used for anti-inflammatory studies (Ghosh et al., Citation2018; Hasnat et al., Citation2015; Miao et al., Citation2019; Ng, Zainal Abidin, Shuib, & Israf Ali, Citation2015). Splenocytes contain B cells, T cells, Monocytes and other immune cells that protect the host from foreign invaders and pivotal inflammatory mediators as well. Nuclear factor-kappa B (NF-κB) is the most widely studied as pivotal inflammatory mediators and activated NF-κB plays a central role as inflammatory mediators that produce pro-inflammatory cytokines/chemokines, apoptosis and transcription factor regulators gene and so on (Gupta, Sundaram, Reuter, & Aggarwal, Citation2010). NF-κB signalling pathway is activated by microbial oppression to modulate host cell. NF-κB complex then translocated into nuclease that generates hundreds of regulatory genes (Rahman & McFadden, Citation2011) that may be perpetuated to tissue damages and leads to inflammatory disease, such as osteoporosis, IBD, atherosclerosis, neurodegeneration, cancer (Ghosh et al., Citation2018; Pervin et al., Citation2016; Wong & Tergaonkar, Citation2009). In normal cells, NF-κB remains in the cytoplasm with inhibitor of NF-κB (IκB) complex and mostly the NF-κB activates by Mitogen-activated protein kinases (MAPKs) such as c-Jun amino-terminal kinase (JNK), extracellular signal-regulated kinases (ERK). The phosphorylation of ERK, JNK, P38 translocate NF-κB into the nucleus. Whereas, degradation of IκB disassociation with NF-κB that kept NF-κB inactive in cytoplasm. NF-κB Nuclear translocation (activated) is considered the hallmark of the inflammatory response that produced pro-inflammatory cytokines and results in chronic diseases. Splenocytes are consist of neutrophils, monocytes, macrophages, and T-lymphocytes which is a vital part of our adaptive and innate immune cells that protect the host of infection and also are important inflammatory mediators and phagocytic role in the physiological process (Bayazid, Kim, Park, & Lim, Citation2020; Ingram, Perry, Scott, Coletta, & Hull, Citation2010; Yuandani, Ilangkovan, Husain, & Chan, Citation2016; Zhang et al., Citation2013). RAW264.7 cells and splenocytes are playing a major role in the elucidation of inflammation.
CLE is consisting of natural secondary metabolites that may have a great impact on inflammation. Recent studies focus on the investigation of functional foods for using as natural anti-inflammatory agents. Our study is designed to evaluate the chicory leaf extract on anti-inflammatory potentialities through NF-κB and MAPK pathway and hepatoprotective activity.
2. Materials and methods
2.1. Chemicals and reagents
Sodium nitrite, trichloroacetic acid (TCA), 2,2-diphenyl-1-picrylhydrazyl (DPPH), 2′7′-dichlorodihydrofluorescein diacetate (H2DCF-DA), 2,2′-azinobis-(3-ethylbenzothiazoline-6-sulfonic acid) (ABTS), potassium persulphate, anhydrous sodium phosphate (dibasic), ammonium thiocyanate, Trolox (6-hydroxy-2,5,7,8-tetramethylchroman-2-carboxylic acid), EDTA, anhydrous sodium phosphate (monobasic) and ferrous sulphate (FeSO4) were purchased from Sigma Aldrich, Sigma Chemical (St. Louis, MO, USA). Stripping Buffer, Plasmid DNA, 2× PCR Master Mix were purchased from Thermo Scientific, USA. Ferric chloride and sodium hydroxide were obtained from Wako Pure Chemical Industries Ltd. (Osaka, Japan). Dulbecco's Modified Eagle's Medium (DMEM), (Foetal Bovine Serum) FBS, DPBS and Trypsin EDTA were purchased from WELGENE Inc. (Seoul, Korea).
2.2. Sample preparation
The Chicory leaves were extracted by 70% (v/v) ethanol in distilled water (DW). Fresh leaves of chicory were lyophilized and 100 g of chicory was soaked in ethanol solution for 24 h at room temperature (RT) (Chou, Kuo, & Lin, Citation2009). This process has been repeated three times. Afterward, the extracted solution was filtered through Whatman No. 1 filter paper (GE Healthcare, Buckinghamshire, UK) and the sample was evaporated in a vacuum rotary evaporator. Then, the concentrated extract had been lyophilized in a freeze dryer and the yield (w/w) was calculated as []. The lyophilized extract was maintained at −20°C before use.
2.3. Total phenol and flavonoid content
Total polyphenol and flavonoid contents were determined by Folin–Ciocalteu and aluminium colorimetric method respectively as reported previously (Debnath et al., Citation2011; Kim et al., Citation2019). The phenolic and flavonoid content of CLE was determined from the regression equation of Gallic acid and catechin standard curve respectively.
2.4. Antioxidant activities
The antioxidant activities were assessed as described earlier (Jeong et al., Citation2018; Kim et al., Citation2019) with some modifications. Trolox equivalent antioxidant capacity (TEAC) was determined from Trolox calibration curve erected by a range of concentration of Trolox (0-80) μmol and its absorbance of DPPH, ABTS and ferric reducing activities were taken at 517, 734 and 700 nm, respectively (Versa max, Molecular Devices, CA, USA) and analysed by SoftMax pro 7 edition. The results were expressed as μM of Trolox equivalent (TE) antioxidant activity per milligram of dry mass.
2.5. Cell Culture
Murine macrophage RAW 264.7 cell had been purchased from Korean Cell Line Bank (Seoul, Korea) and maintained by DMEM with 10%FBS and 1% penicillin streptomycin. Splenocytes were isolated from ICR 6 weeks old male mice (Orient Bio Inc. Seongnam, Korea) and human liver carcinoma (Hep G2) cells were maintained in RPMI 1640(Gibco, NY, USA) supplemented with 10% FBS and 1% P.S. The cells were maintained in a humid incubator at 37°C with 5% CO2.
2.6. Cellular antioxidant activity
Intracellular antioxidant activity was examined by the oxidation of 2′,7′-dichlorodihydro-fluorescein diacetate (H2DCF-DA) to fluorescent 2′,7′-dichlorofluorescein (DCF) method as described (Ghosh et al., Citation2018; Jeong et al., Citation2018) with some changes. Briefly, 5 × 104 RAW 264.7 cells in DMEM and 1 × 105 Hep G2 (Human Liver Carcinoma) in RPMI 1640 were seeded in each well in a 96 well plate. After 24 h of incubation, the RAW 264.7 cells were treated with a range of concentrations of sample (10–1000) μg/mL and Hep G2 treated with (10–500) μg/mL of sample for another 24 h. In both cases, 25 μg/mL of Gallic acid (HPLC grade, Sigma Aldrich) was added into the cells and were considered as a positive control. Afterward, 10 μM H2DCF-DA in media was added into the cells for 40 min then 60 μM H2O2 was added and incubated for 1hour. The fluorescence was measured using SoftMax pro 5 at the excitation at 485 nm and the emission at 530.
2.7. Cell viability
Cell viability was investigated by MTT (3-(4,5-dimethylthiazol-2-yl)-2,5 diphenyltetrazolium bromide) assay as previously mentioned (Hasnat et al., Citation2015) with some modifications. In short, 5 × 104 RAW 264.7 cells in DMEM and 1 × 105 Hep G2 in RPMI were seeded and incubated for 24 h in the incubator. After that, Raw 264.7 cells were treated with different concentrations of CLE (10-1000) μg/mL and LPS 1 μg/mL for more 24 h. In the case of Hep G2 cells were treated by CLE (10–500) μg/mL with or without H2O2 (400 μM). 25 μg/mL Gallic acid (GA) was added into the cells as a positive control. After incubation 0.5 mg/mL MTT (final concentration) was added to every well and kept in the incubator for 2 h. The supernatant was then removed and DMSO was added to solubilize the formazan and kept at room temperature in a dark condition for 10 min and absorbance was taken at 570 nm (Versa max, Molecular Devices, CA, USA) and analysed by SoftMax pro 7 edition.
2.8. NO assay
Nitric oxide (NO*) production by LPS-induced RAW 264.7 cells was evaluated by Griess assay (Hasnat et al., Citation2015; Miao et al., Citation2019). The cells were seeded as 5 × 104 cells/well in a 96-well plate and incubated for 24 h. Afterward, cells were treated with LPS (1 μg/mL) and CLE (10–1000) μg/mL and GA for another 18 h, where the control group contained only DMEM. Then, 80 μL supernatant from each well was collected and added into a new 96-well plate and mixed with the same amount of Griess reagent. The mixture was kept in a shaker at room temperature under the dark condition for 10 min before taking absorbance at 540 nm. Nitric Oxide (NO) was determined from the standard curve of NaNO2.
2.9. Splenocytes proliferation
Primary Spleen cells were isolated from ICR 5weeks old male mice (Orient Bio Inc. Seongnam, Korea) as previous described (Bayazid et al., Citation2020; Yang, Kyoung Seo, Lee, & Young Lee, Citation2015) and maintained in RPMI 1640 (WELGENE Inc. Seoul, Korea) media supplemented with 10% FBS and 1% P.S. The cells were seeded in a 24-well plate as 2 × 106 cells/well. 24 h later, 100 ng/mL of LPS-induced in cells with or without CLE (100, 200 and 400 μg/mL). 24 h later, 100 ng/mL of LPS-induced in cells with or without CLE (100, 200 and 400 μg/mL). Control group was treated only with media. After 24 h of incubation, cell counting was performed by cytometer using trypan blue and MTT assay performed as previously reported (Ghosh et al., Citation2018).
2.10. Western blotting analysis
RAW 264.7 cells in DMEM were seeded as 1 × 106 cells/well and primary spleen cells in RPMI 1640 were seeded as 2 × 106 cells/well in a 6-well plate and incubated 24 h. Then, the cells were treated with CLE (100 and 250) μg/mL and LPS (1 μg/mL) followed by 12 h incubation. Afterward, total protein was extracted using PRO-PREP buffer (iNtRON Biotechnology) and the protein was separated from cell lysate by centrifugation at 14,000 × g (4°C). An equal amount of protein (30 μg/lane) was subjected into 10% SDS polyacrylamide gel. After electrophoresis, protein samples were transferred into Polyvinylidene fluoride (PVDF) membrane and blocked by 5% BSA. The membranes were incubated with corresponding primary antibodies at 4°C overnight then the membranes were incubating with secondary antibody (anti-rabbit) at room temperature for 1hour. Then, the immunosignals in membranes were visualized by enhanced chemiluminescence (ECL) in iBright (Invitrogen). The band intensity was quantified by ImageJ software.
2.11. Confocal microscopy
The RAW 264.7 cells were seeded in a 6-well plate as 5 × 104 cells/well and incubated overnight. Afterward, cells were pretreated with CLE (400 μg/mL) after 1 h LPS (1 μg/mL) induced and incubated for more 12 h. The media was aspirated and cells were washed by ice-cold PBS and fixed by 4% paraformaldehyde solution for 15 min (Miao et al., Citation2019). After blocking by blocking buffer, the cells were incubated with primary antibody rabbit anti-P65 (1:500) at 4°C overnight after rinsed cells were treated with secondary antibody (1:1000) (Alexa Fluor 568 goat anti-rabbit IgG, H + L) for incubation at room temperature for 1 h in the dark. The cells specimen were stained by Hoechst 33342 dye (Thermo Fisher Scientific) and take an image by the confocal microscope.
2.12. mRNA expression by reverse transcriptase polymerase chain reaction (RT-PCR)
RAW 264.7 cells were seeded in a 6-well plate as 1 × 106 cells/well and incubated for 24 h. The cells were treated with or without LPS (1 μg/mL) and CLE (100 and 250) μg/mL for 12 h. After washing with ice-cold Phosphate buffer saline (PBS) the total RNA was extracted by using TRIzol RNA isolation reagent (Life Technologies, CA, USA) as per manufacturer instructions. Isolated RNA was quantified by nanodrop and an equal amount of RNA (2 μg) from each group was reverse transcription using SuperScript II kit (Invitrogen, San Diego, CA, USA) as mentioned before (Hasnat et al., Citation2015; Jimenez et al., Citation2008). PCR amplification was performed using Emerald Amp 2× PCR Master Mix (Takara) according to manufacturer instructions. mRNA bands were electrophoresed and stained with ethidium bromide (Pervin et al., Citation2016). The primer sequences were described in . The bands were captured by iBright (Invitrogen) and band intensity was measured by ImageJ software.
Table 1. Sequences of primers used for RT-PCR in this study.
2.13. Determination of gallic acid
Bioactive compounds of CLE were determined in Thermo Scientific Dionex Ultimate 3000 series consist of C18 analytical column (Thermo Fisher Scientific, Waltham, MA, USA) that 4.6 × 250 mm, 5 μm particle size, Supelco C-18 column. For identifying bioactive compounds in CLE, water was used as mobile phase A and acetonitrile was used as mobile phase B (elution conditions: 0–5 min, 0% B; 5–35 min, 90% B; 35–40 min, 100%; flow rate, 0.8 mL/min; injection volume, 20 μL; wavelength, 280 nm and column temperature 25°C). On-line HPLC against ABTS radical did as same gradient setting and column at 734 nm using PDA (Photodiode Array). Before injecting, all standards (11 standards were analysed that obtained from Sigma Aldrich HPLC grade) and sample were dissolved in 70%ethanol and filtered through 0.45 μm membrane filter. All solvents and mobile phases were HPLC grade and polyphenols were confirmed by matching the retention time with the standards.
2.14. Statistical analysis
The results were demonstrated as mean ± SD for all experimental data. Data analyses were performed with Microsoft Excel 2016 edition and GraphPad Prism 5.0 software (GraphPad Software, Inc., San Diego, CA, USA) using a one-way analysis of variance according to Tukey's Multiple Comparison Test. p-Value less than 0.05 was considered statistically significant. All experimental data were performed at least triplicate.
3. Results
3.1. Total polyphenol and flavonoid contents of chicory leaf extract
The yield of green chicory leaf extract (CLE) was 28.6%. Polyphenols and flavonoids are secondary metabolites these are tremendous bio-effective and exert enormous antioxidant activities (Hendrich, Citation2006). Total phenolic content (TPC) and total flavonoid content (TFC) were illustrated in as gram and milligram (mg) of dry mass respectively. TPC and TFC of Chicory leaf were 2.11 ± 0.24 g and 500.5 ± 24.32 mg per 100 g of dry mass.
Table 2. Total phenolic, flavonoids content and yield of the green chicory leaves.
3.2. Antioxidant activities of chicory leaf extract
Antioxidant activities of CLE expressed as Trolox equivalent against DPPH, Ferric Reducing and ABTS radical scavenging activities are shown in . Trolox is a vitamin E derivative that is well-known for its robust and tremendous free radicals scavenging activities (Alberto, Russo, Grand, & Galano, Citation2013). Chicory leaf is an enormous source of polyphenols (Sahan et al., Citation2017) and antioxidant activities are a major characteristic of polyphenols. The ferric reducing, DPPH and ABTS radical scavenging activities were 126.26 ± 0.89, 77.87 ± 2.38 and 110.64 ± 022 μM Trolox equivalent per milligram (mg) of the dry mass of chicory leaves respectively.
Table 3. Antioxidant of chicory leaf extract.
3.3. Cellular antioxidant activities
Previous ROS suppressive activities by measuring the conversion of H2DCF-DA to DCF in cells were investigated in many studies as a cellular antioxidant activity and ROS behaves like a secondary messenger in many inflammatory pathways (Chen, Zhang, et al., Citation2019; Ghosh et al., Citation2018; Lander, Citation1997). CLE suppressed significantly the ROS generation from the concentration of 10 μg/mL and reduced in a dose-dependent manner in H2O2 accumulated RAW 264.7 cells and Hep G2 Cells. Eventually, CLE extract restored the ROS generation which is demonstrated in . Positive control (GA) reduced ROS generation more in Hep G2 than RAW 264.7 cells.
3.4. Effects of CLE on cell viability and NO production in LPS-induced RAW 264.7 cells
MTT assay widely used for evaluating cell viability assay (Alim et al., Citation2019; Cásedas, González-Burgos, Smith, López, & Gómez-Serranillos, Citation2018; Miao et al., Citation2019). RAW 264.7 cells were damaged by LPS while CLE significantly attenuated cell death at the concentration of 10 μg/mL and completely restored cell viability from the concentration of 100 μg/mL. CLE showed no toxic effect up to 1000 μg/mL (not showed) when treating alone and protective effect against LPS-induced cells that demonstrated in (a). The production of NO by macrophages is an important inflammatory response (Taciak et al., Citation2018). LPS-treated cells increased NO production drastically and that is inhibited by CLE dose-dependently. CLE significantly suppressed the NO production showed in (b). CLE reduced NO production to 56.63, 50.25, 38.625 and 26.4 μM with the concentration of 100, 250, 500 and 1000 μg/mL respectively. CLE results have shown more effective than the GA from the concentration of 250 μg/mL in RAW 264.7 cells.
Figure 2. The effect of CLE on LPS-induced cell viability, proliferation and NO production. (a) Cell viability of RAW 264.7, cells treated with different concentrations of CLE with 1 μg/mL LPS. (b) The inhibitory rate of NO production in LPS-induced RAW 264.7 of CLE. Human liver cells (Hep G2) were treated with CLE for 24 h in the presence or absence of 1 μg/mL LPS. (c) Effect of CLE on Hep G2 (human liver carcinoma) cells. (d) Protective effect of CLE on Hep G2 cells against oxidative damages. Data were expressed as the mean ± SD of three separate experiments; where n = 3. *p < 0.05, **p < 0.01, ***p < 0.001 compared to the LPS or H2O2-treated group.
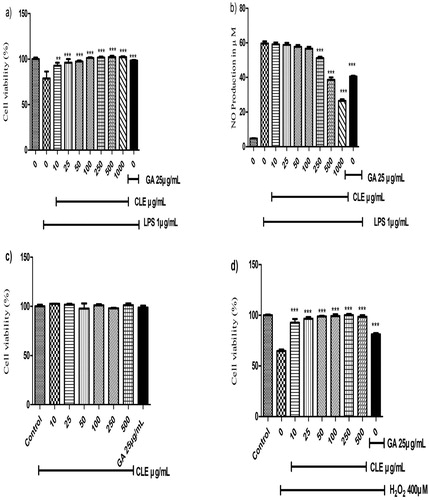
3.5. Hepatoprotective effect of CLE on Hep G2 cells against oxidative damage
The hepatoprotective effect of CLE against oxidative damages was investigated by MTT assay. CLE has no cytotoxic effect on Human Liver Carcinoma (Hep G2) cells up to 500 μg/mL. Furthermore, CLE attenuated cell viability against oxidative damage by 400 μM H2O2 induced in Hep G2 cells. H2O2 reduced cell viability to about 65% of control. Where, CLE showed remarkable hepatoprotective activity and almost repaired cell death from the concentration of 10 μg/mL and restored the cell viability in a dose-dependent manner and reached 100% cell viability from the concentration of 100 μg/mL that illustrated in (c,d).
3.6. Effect of CLE on immunoblotting of NF-κB/MAPKs activation and cytokines production
Immunoblotting was conducted to elucidate the underlying molecular mechanism of inflammatory response. LPS increases NF-κB and Mitogen-activated protein kinases (MAPKs) activation as in inflammatory responses. MAPKs play essential roles in the activation of transcription factor NF-κB that results chronic inflammation (Yuan, Chen, Sun, Guan, & Xu, Citation2013). On the other hand, NF-κB-inhibitor of kappa Bα (IκBα) downregulates the NF-κB translocate in the nucleus to bind with pro-inflammatory cytokines gene promoters and increased their production (Hasnat et al., Citation2015; Tietze et al., Citation2000). We found CLE inhibited the degradation of IκBα by LPS and the phosphorylation of NF-κB (P-65) as well as. CLE restored the P-38, ERK1/2 and JNK activation in the stimulated macrophage attenuated when it treated with CLE. The interaction among TNF-α, IL-1β, IL-6 perpetuates a vicious cycle in the cytokine network. CLE reduced the expression of TNF-α, IL-1β, IL-6 as described in (a).
Figure 3. (a) The effect of chicory extract on the phosphorylation of MAPKs, NF-kB signalling pathways and pro-inflammatory cytokines production in LPS-stimulated RAW 264.7 cells. Histograms were presented as Mean ± SD, where n = 3. *p < 0.05, **p < 0.01, ***p < 0.001 compared to the LPS-treated group. (b) The effect of chicory extract on NF-kB nucleus translocation in LPS-stimulated RAW 264.7 cells.
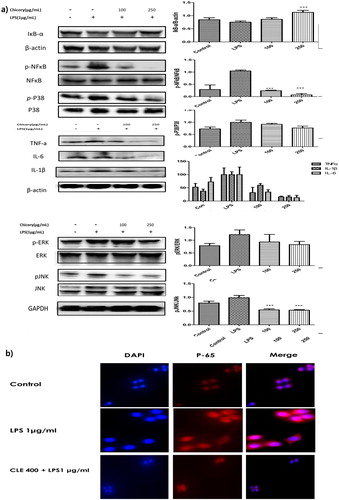
3.7. Effect of CLE on P-65 nuclear translocation in the LPS-stimulated RAW 264.7 cells
Confocal Microscopy was performed to investigate the P-65(NF-κB) translocation in the nucleus by LPS (1 μg/mL) induced RAW 264.7 cells. Where LPS-treated cell was larger than the control group and that remarkably restored by in 400 μg/mL CLE pre-treatment. Besides, cells shape transformation by LPS that fixed by the CLE (b). Red fluorescence indicated the presence of P-65 level that enhanced by LPS stimulation and notably suppressed by the CLE.
3.8. Effect of CLE on inflammatory cytokines mRNA expression in RT-PCR
The expressions of iNOS and COX-2 were markedly increased by LPS (1 μg/mL) and CLE treatment ameliorated the upregulation of both iNOS and COX-2 significantly at the concentration of 250 μg/mL. TNF-α, IL-1β, IL-6 and IFN-γ upregulation is correlated with NF-κB activation (Hasnat et al., Citation2015). As described in CLE significantly suppressed the increase of TNF-α, IL-1β, IFN-γ and IL-6 induced by LPS. The anti-inflammatory effects of CLE were re-confirmed at mRNA expressions.
3.9. Effect of CLE on proliferation and NF-κB/MAPK activation of splenocytes
LPS could stimulate splenocyte proliferation which may cause arthritis, autoimmune diseases and trigger pro-inflammatory cytokines, phagocytosis, tissue damaged (Zhang et al., Citation2013). LPS stimulation activates the splenocytes that are an indicator of inflammatory response. LPS stimulation enhanced the splenocytes proliferation notably and that significantly reversed by CLE as described in (a,b). MTT assay was expressed as % of control and cell proliferation was increased by about 170% of control when treated with LPS only that very significantly reduced by CLE. Cell counting is investigated by trypan blue and similar results have been shown in cell counting. The number of cells reversed to about 1.407 × 106 from 2.5 × 106 (LPS-treated) at 400 μg/mL of CLE. MTT assay and cell counting CLE significantly decreased the splenocytes dose-dependently. In (c) CLE restored the activated NF-κB and MAPK protein expression in LPS-stimulated primary spleen cells. NF-κB signalling associated the escalation of the proliferation of splenocytes.
Figure 5. Splenocytes were treated with 100 ng/mL LPS in the presence or absence of CLE for 24 h. (a) Splenocytes proliferation (expressed as percentage of control) was measured by the MTT assay. (b) Mean number of splenocytes × 10³/well. Data were expressed as the mean ± SD of three separate experiments; where n = 3. *p < 0.05, **p < 0.01, ***p < 0.001 compared to the LPS-treated group. (c) The effect of CLE on the activation of MAPKs, NF-kB signalling pathways against LPS (1 μg/mL) induced in primary spleen cells.
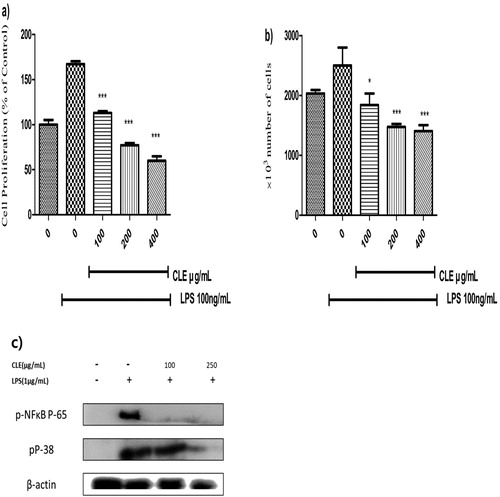
3.10. Determination of major bioactive compounds in CLE
Among 11 standards (Naringin, 4-Hydroxybenzoic Acid. Ascorbic Acid, Trans-cinnamic Acid, Chlorogenic Acid, Vanilic Acid, Quercetin, Ellagic Acid, Rutin hydrate, Syringic Acid, Gallic acid) were analysed. we determined gallic acid in green chicory leaf extract (CLE) described in . Which inferred the prominent bioactive compound in the CLE and determined 18.86 ± 1.8 mg per gram of dry mass that indicates over 80% of the total phenolic content of CLE (according to FC method). Moreover, On-line HPLC against ABTS radical illustrated in (Supplementary Figure) showed almost similar activity as gallic acid that emphasized our data as evidential. Although the previous study reported (Sahan et al., Citation2017) that the several polyphenols found in the green chicory where extract contained other parts of chicory (e.g. root, flower, etc.). Hence, gallic acid in green CLE was found as previously reported (Nwafor et al., Citation2017). We investigated the effect of Gallic acid and CLE on NO* production on LPS-induced RAW 264.7 cells. The results associated with our HPLC analysis is illustrated in .
Figure 6. HPLC chromatograms of the CLE. (a) The chromatogram of the CLE (10 mg/mL), (b) The chromatogram of the standard sample gallic acid (0.25 mg/mL). According to HPLC analysis, 50, 100, 250, 500 and 1000 µg/mL of CLE contains about 3.2, 6.3, 15.8, 31.6 and 63.23 µg/mL of gallic acid, respectively. NO assay and cell viability assay were performed as previously described in this study. (c) gallic acid (Sigma Aldrich HPLC grade) showed almost similar effect as CLE on NO assay in LPS-induced RAW 264.7cells. (d) CLE attenuated cell viability against LPS and no cytotoxic effect in RAW 264.7cells, while the highest concentration (63.23 µg/mL) of gallic acid the cell viability was less than LPS group that indicated cytotoxicity of gallic acid. Data were expressed as the mean ± SD of three separate experiments; where n = 3.
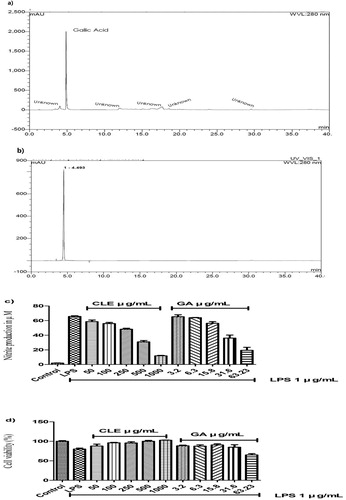
Table 4. Major bioactive components in CLE.
4. Discussions
This study extensively found the antioxidant and anti-inflammatory effects of the green chicory leaf extract (CLE) with the elucidation of molecular mechanisms in murine macrophages. Previously, CLE was examined as a tremendous source of polyphenol (Sahan et al., Citation2017; Sinkovič et al., Citation2015) and though we found a high content of gallic acid among 11 standards and Our study highly recommended to characterize the bioactive compounds in the green chicory leaf. Phenolic compounds that mostly found in plants are play roles in the prevention of oxidative damage in living organisms that perpetuated an enormous amount of chronic diseases (Graziani et al., Citation2005; Mijan et al., Citation2019; Tang et al., Citation2019). Furthermore, reactive oxygen species (ROS) are inevitable by-products in physiological processes that required neutralized by antioxidants and enzymes. Though, ROS play a crucial role like regulations of signalling pathway. Hence, excessive ROS cause various toxic effects, aging, diabetes, intracellular damages and many other metabolic disorders (Di Meo, Reed, Venditti, & Victor, Citation2016; Jeong et al., Citation2018). CLE showed tremendous free radical scavenging and cellular antioxidant activities by suppressing the ROS production in both Raw 264.7 and Hep G2 (Human Liver Carcinoma) cells. CLE restored Hep G2 cells damaged by oxidative stress significantly.
Nevertheless, in few studies, phenolics had been found to cause oxidative damage in cells (Ohshima, Yoshie, Auriol, & Gilibert, Citation1998; Yang, Liao, Kim, Yurkow, & Yang, Citation1998). We performed MTT assay where CLE showed no toxic effect in RAW 264.7 cells (data not shown). Moreover, CLE restored cell viability against LPS-induced cell death. Meanwhile, CLE significantly suppressed LPS (100 ng/mL) stimulated spherocytes proliferation. Splenocytes contain B cells, T cells, Monocytes and other immune cells that protect the host from foreign invaders and as well as inflammatory We performed MTT assay where CLE showed mediators. Splenocytes activated by mitogen/pyrogen to recruits immune cells (e.g. neutrophils, monocytes, macrophages, and T-lymphocytes) that upregulate proliferation and may attack other host cells that result in tissue damages and inflammatory diseases. CLE showed remarkable anti-proliferative activity on LPS-induced splenocytes (Yuandani et al., Citation2016; Zhang et al., Citation2013). Furthermore, NO production is an inflammatory biomarker and excessive NO releases associated with the inducible nitric oxide synthase (iNOS) that involved highly in the inflammatory response (Miao et al., Citation2019). CLE showed significant inhibitory activities of NO production in LPS-induced RAW 264.7 cells.
Inflammation is the action of innate and adaptive immune cells that essential for host defense mechanisms and tissue homeostasis. On the other hand, adverse inflammation perpetuated Inflammatory diseases like cancer, IBD, diabetes, osteoporosis, atherosclerosis and so on (Chen, Teng, et al., Citation2019; Ghosh et al., Citation2018; Greten & Grivennikov, Citation2019). However, inflammatory responses remain complex and its mechanisms are not fully understood. Macrophages play a major role in inflammation (Miao et al., Citation2019; Schappe et al., Citation2018) and RAW 264.7 cell line is vastly used for studying pro-inflammatory mediators and cytokines expressions because of its reproducible response against LPS and the cell line successfully established for anti-inflammatory investigations (Peng, Ai-lati, Ji, Chen, & Mao, Citation2019). NF-κB activates by LPS and other antigen receptor signalling pathways while there is more than one pathway for regulating the pro-inflammatory cytokines but the NF-κB (P65) is a pivotal transcription factor. The nuclear factor-kappa B (NF-κB) regulates multiples aspect of inflammation by both innate and adaptive immune cells thus NF-κB is considered in the central role of inflammation that produces varieties of pro-inflammatory cytokine and chemokines by translocating in nucleus and transcription (Liu, Zhang, Joo, & Sun, Citation2017). Those result in chronic disorders. Previous studies illustrated in mice with deficient NF-κB subunits reported that the transcription factor is necessary for the lymphocyte response to antigens and cytokine-inducible gene expression precisely P65 subunit is essential for the induction of TNF-α dependent genes (Miao et al., Citation2019). CLE restored NF-κB P65 and MAPK phosphorylation in RAW 264.7 cells and primary spleen cells isolated from ICR male mice very significantly. Splenocytes proliferation is associated with the NF-κB activation and CLE reversed the splenocytes proliferation against inflammatory response LPS induction. Moreover, our study showed suppression of MAPK (p38), ERK and JNK activation which is the mediator of NF-κB phosphorylation and translocate into the nucleus in RAW 264.7 cells. On the other side, CLE modulated inhibitor of NF-κB alpha (IκB-α) expression that binds with NF-κB and inhibit to translocating into the nucleus. Previously reported that MAPKs phosphorylate IκB in LPS-induced cell. CLE modulated IκB-α expression that suppressed NF-κB activation. Consequently, CLE restored the pro-inflammatory cytokine gene expression such as TNF-α, IL-1β, IFN-γ, IL-6 effectively through activated NF-κB in LPS-induced murine macrophages. TNF-α, IL-1β and IL-6 are dominant cytokines that found increased in colitis mucosa in DSS induced mice (Hasnat et al., Citation2015). Moreover, CLE significantly reduced iNOS and COX-2 mRNA expression.
To conclude, the CLE is rich in gallic acid and other bioactive compounds that have been reported tremendous effects against inflammation (BenSaad, Kim, Quah, Kim, & Shahimi, Citation2017; Kroes, Van Den Berg, Van Ufford, Van Dijk, & Labadie, Citation1992) and gallic acid (GA) also acts as a regulator of NF-κB activation (Gupta et al., Citation2010). Furthermore, we investigated almost similar CLE consist of many natural bio-functional components such as phenolics, polysaccharides, flavonoids compounds and so on. CLE confirmed the effect of anti-inflammatory effect is mediated by its bioactive compounds with the modulation in murine macrophage and primary spleen cells. Moreover, these findings suggest metabolism, characterizations of the bioactive components and bioavailability of CLE for further studies. Our study firmly envisaged Chicory leaf could be considered as a functional food which used as a natural anti-inflammatory agent to treat against inflammatory and liver diseases with promising outcomes for further uses.
Acknowledgements
This research was supported by Konkuk University in 2018.
Disclosure statement
No potential conflict of interest was reported by the author(s).
Additional information
Funding
References
- Al Mijan, M., & Lim, B. O. (2018). Diets, functional foods, and nutraceuticals as alternative therapies for inflammatory bowel disease: Present status and future trends. World Journal of Gastroenterology, 24(25), 2673. doi: 10.3748/wjg.v24.i25.2673
- Alberto, M. E., Russo, N., Grand, A., & Galano, A. (2013). A physicochemical examination of the free radical scavenging activity of Trolox: Mechanism, kinetics and influence of the environment. Physical Chemistry Chemical, 15(13), 4642–4650. doi: 10.1039/c3cp43319f
- Alim, I., Caulfield, J. T., Chen, Y., Swarup, V., Geschwind, D. H., Ivanova, E., … Marie, E. J. (2019). Selenium drives a transcriptional adaptive program to block ferroptosis and treat stroke. Cell, 177(5), 1262–1279.e1225. doi: 10.1016/j.cell.2019.03.032
- Azzini, E., Maiani, G., Garaguso, I., Polito, A., Foddai, M. S., & Venneria, E. (2016). The potential health benefits of polyphenol-rich extracts from Cichorium intybus L. Studied on Caco-2 cells model. Oxidative Medicine and Cellular Longevity, 2018(9). doi: 10.1155/2016/1594616
- Bayazid, A. B., Kim, J. G., Park, S. H., & Lim, B. O. (2020). Antioxidant, anti-inflammatory, and antiproliferative activity of Mori Cortex Radicis extracts. Natural Product Communications, 15(1). doi: 10.1177/1934578x19899765
- BenSaad, L. A., Kim, K. H., Quah, C. C., Kim, W. R., & Shahimi, M. (2017). Anti-inflammatory potential of ellagic acid, gallic acid and punicalagin A&B isolated from Punica granatum. BMC Complementary and Alternative Medicine, 17(1), 47. doi: 10.1186/s12906-017-1555-0
- Cásedas, G., González-Burgos, E., Smith, C., López, V., & Gómez-Serranillos, M. P. J. (2018). Sour cherry (Prunus cerasus L.) juice protects against hydrogen peroxide-induced neurotoxicity by modulating the antioxidant response. Journal of Functional Foods, 46, 243–249. doi: 10.1016/j.jff.2018.04.055
- Chandel, N. S., & Tuveson, D. A. (2014). The promise and perils of antioxidants for cancer patients. New England Journal of Medicine, 371(2), 177–178. doi: 10.1056/NEJMcibr1405701
- Chen, H.-W., Yang, M.-Y., Hung, T.-W., Chang, Y.-C., & Wang, C.-J. (2019). Nelumbo nucifera leaves extract attenuate the pathological progression of diabetic nephropathy in high-fat diet-fed and streptozotocin-induced diabetic rats. Journal of Food and Drug Analysis, 27(3), 736–748. doi: 10.1016/j.jfda.2018.12.009
- Chen, L., Teng, H., Jia, Z., Battino, M., Miron, A., & Yu, Z. (2018). Intracellular signaling pathways of inflammation modulated by dietary flavonoids: The most recent evidence. Critical Reviews in Food Science and Nutrition, 58(17), 2908–2924. doi: 10.1080/10408398.2017.1345853
- Chen, Y., Zhang, H., Liu, R., Mats, L., Zhu, H., Pauls, K. P., … Tsao, R. (2019). Antioxidant and anti-inflammatory polyphenols and peptides of common bean (Phaseolus vulga L.) milk and yogurt in Caco-2 and HT-29 cell models. Journal of Functional Foods, 53, 125–135. doi: 10.1016/j.jff.2018.12.013
- Chou, H.-J., Kuo, J.-T., & Lin, E.-S. (2009). Comparative antioxidant properties of water extracts from different parts of Beefsteak plant (Perilla frutescens). Journal of Food & Drug Analysis, 17(6), 489–496.
- Debnath, T., Park, P.-J., Nath, N. C. D., Samad, N. B., Park, H. W., & Lim, B. O. (2011). Antioxidant activity of Gardenia jasminoides Ellis fruit extracts. Food Chemistry, 128(3), 697–703. doi: 10.1016/j.foodchem.2011.03.090
- Di Meo, S., Reed, T. T., Venditti, P., & Victor, V. M. (2016). Harmful and beneficial role of ROS. Oxidative Medicine and Cellular Longevity, 2016. doi: 10.1155/2016/7909186
- Ghosh, M., Kim, I., Lee, Y., Hong, S., Lee, T., Lim, J., … Lim, B. (2018). The effects of aronia melanocarpa ‘viking’extracts in attenuating RANKL-induced osteoclastic differentiation by inhibiting ROS generation and c-FOS/NFATc1 signaling. Molecules, 23(3), 615. doi: 10.3390/molecules23030615
- Graziani, G., D’argenio, G., Tuccillo, C., Loguercio, C., Ritieni, A., Morisco, F., … Romano, M. (2005). Apple polyphenol extracts prevent damage to human gastric epithelial cells in vitro and to rat gastric mucosa in vivo. J. Gut, 54(2), 193–200. doi: 10.1136/gut.2004.046292
- Greten, F. R., & Grivennikov, S. I. (2019). Inflammation and cancer: Triggers, mechanisms, and Consequences. Immunity, 51(1), 27–41. doi: 10.1016/j.immuni.2019.06.025
- Gupta, S. C., Sundaram, C., Reuter, S., & Aggarwal, B. B. (2010). Inhibiting NF-κB activation by small molecules as a therapeutic strategy. Biochimica et Biophysica Acta (BBA)-Gene Regulatory Mechanisms, 1799(10–12), 775–787. doi: 10.1016/j.bbagrm.2010.05.004
- Hasnat, M. A., Pervin, M., Cha, K. M., Kim, S. K., & Lim, B. O. (2015). Anti-inflammatory activity on mice of extract of Ganoderma lucidum grown on rice via modulation of MAPK and NF-κB pathways. Phytochemistry, 114, 125–136. doi: 10.1016/j.phytochem.2014.10.019
- Hendrich, A. B. (2006). Flavonoid-membrane interactions: Possible consequences for biological effects of some polyphenolic compounds 1. Acta Pharmacologica Sinica, 27(1), 27–40. doi: 10.1111/j.1745-7254.2006.00238.x
- Ingram, N., Perry, S. L., Scott, N., Coletta, P., & Hull, M. (2010). PWE-003 Loss of interleukin-4 receptor [alpha] function drives initiation, but not progression, of azoxymethane-induced colorectal carcinogenesis in BALB/c mice. Gut, 59, A86. doi: 10.1136/gut.2009.209072y
- Jancic, D., Todorovic, V., Sircelj, H., Dodevska, M., Beljkas, B., Znidarcic, D., & Sobajic, S. (2017). Biologically active compounds and antioxidant capacity of Cichorium intybus L. Leaves from Montenegro. Italian Journal of Food Science, 29(4). doi: 10.14674/IJFS-789
- Jeong, H., Sung, J., Yang, J., Kim, Y., Jeong, H. S., & Lee, J. J. (2018). Effect of sucrose on the functional composition and antioxidant capacity of buckwheat (Fagopyrum esculentum M.) sprouts. Journal of Functional Foods, 43, 70–76. doi: 10.1016/j.jff.2018.01.019
- Jimenez, A. D. P., Viriyakosol, S., Walls, L., Datta, S., Kirkland, T., Heinsbroek, S., … Fierer, J. (2008). Susceptibility to Coccidioides species in C57BL/6 mice is associated with expression of a truncated splice variant of Dectin-1 (Clec7a). Genes & Immunity, 9, 338–348. doi: 10.1038/gene.2008.23
- Kim, Y.-S., Kim, E.-K., Dong, X., Park, J.-S., Shin, W.-B., Kim, S.-J., … Lim, B.-O. (2019). Lindera glauca (Siebold et Zucc.) Blume Stem Extracts protect against tert-Butyl hydroperoxide-induced oxidative stress. Journal of Medicinal Food, 22(5), 508–520. doi: 10.1089/jmf.2018.4289
- Kroes, B. V., Van Den Berg, A., Van Ufford, H. Q., Van Dijk, H., & Labadie, R. P. (1992). Anti-inflammatory activity of gallic acid. Planta Medica, 58(06), 499–504. doi: 10.1055/s-2006-961535
- Lander, H. M. (1997). An essential role for free radicals and derived species in signal transduction. The FASEB Journal, 11(2), 118–124. doi: 10.1096/fasebj.11.2.9039953
- Liu, T., Zhang, L., Joo, D., & Sun, S.-C. (2017). NF-κB signaling in inflammation. Journal of Physical Therapy Science, 2, 17023.
- Miao, L., Tao, H., Peng, Y., Wang, S., Zhong, Z., El-Seedi, H., … Wang, Y. J. (2019). The anti-inflammatory potential of Portulaca oleracea L. (purslane) extract by partial suppression on NF-κB and MAPK activation. Food Chemistry, 290, 239–245. doi: 10.1016/j.foodchem.2019.04.005
- Mijan, M. A., Kim, J.-Y., Moon, S.-Y., Choi, S.-H., Nah, S.-Y., & Yang, H.-J. (2019). Gintonin enhances proliferation, late stage differentiation, and cell survival from endoplasmic reticulum stress of oligodendrocyte lineage cells. Frontiers in Pharmacology, 10, 1211. doi: 10.3389/fphar.2019.01211
- Montefusco, A., Semitaio, G., Marrese, P. P., Iurlaro, A., De Caroli, M., Piro, G., … Lenucci, M. S. (2015). Antioxidants in varieties of chicory (Cichorium intybus L.) and wild poppy (Papaver rhoeas L.) of Southern Italy. Journal of Chemistry, 2015(8). doi: 10.1155/2015/923142
- Ng, R. F. L., Zainal Abidin, N., Shuib, A. S., & Israf Ali, D. A. (2015). Inhibition of nitric oxide production by Solanum melongena and Solanum macrocarpon on RAW 264.7 cells. Frontiers in Life Science, 8(3), 241–248. doi: 10.1080/21553769.2015.1051241
- Nwafor, I. C., Shale, K., & Achilonu, M. C. (2017). Chemical composition and nutritive benefits of chicory (Cichorium intybus) as an ideal complementary and/or alternative livestock feed supplement. The Scientific World Journal, 2017(11). doi: 10.1155/2017/7343928
- Ohshima, H., Yoshie, Y., Auriol, S., & Gilibert, I. (1998). Antioxidant and pro-oxidant actions of flavonoids: Effects on DNA damage induced by nitric oxide, peroxynitrite and nitroxyl anion. Free Radical Biology and Medicine, 25(9), 1057–1065. doi: 10.1016/S0891-5849(98)00141-5
- Peng, L., Ai-lati, A., Ji, Z., Chen, S., & Mao, J. (2019). Polyphenols extracted from Huangjiu have anti-inflammatory activity in lipopolysaccharide stimulated RAW2647 cells. RSC Advances, 9(10), 5295–5301. doi: 10.1039/C8RA09671F
- Pervin, M., Hasnat, M. A., Lim, J.-H., Lee, Y.-M., Kim, E. O., Um, B.-H., & Lim, B. O. (2016). Preventive and therapeutic effects of blueberry (Vaccinium corymbosum) extract against DSS-induced ulcerative colitis by regulation of antioxidant and inflammatory mediators. The Journal of Nutritional Biochemistry, 28, 103–113. doi: 10.1016/j.jnutbio.2015.10.006
- Rahman, M. M., & McFadden, G. (2011). Modulation of NF-κB signalling by microbial pathogens. Nature Reviews Microbiology, 9(4), 291. doi: 10.1038/nrmicro2539
- Sahan, Y., Gurbuz, O., Guldas, M., Degirmencioglu, N., & Begenirbas, A. (2017). Phenolics, antioxidant capacity and bioaccessibility of chicory varieties (Cichorium spp.) grown in Turkey. Food Chemistry, 217, 483–489. doi: 10.1016/j.foodchem.2016.08.108
- Schappe, M. S., Szteyn, K., Stremska, M. E., Mendu, S. K., Downs, T. K., Seegren, P. V., … Stipes, E. J. (2018). Chanzyme TRPM7 mediates the Ca2+ influx essential for lipopolysaccharide-induced toll-like receptor 4 endocytosis and macrophage activation. Immunity, 48(1), 59–74.e55. doi: 10.1016/j.immuni.2017.11.026
- Sinkovič, L., Demšar, L., Žnidarčič, D., Vidrih, R., Hribar, J., & Treutter, D. (2015). Phenolic profiles in leaves of chicory cultivars (Cichorium intybus L.) as influenced by organic and mineral fertilizers. Food Chemistry, 166, 507–513. doi: 10.1016/j.foodchem.2014.06.024
- Sinkovič, L., Hribar, J., & Vidrih, R. J. C. (2014). Influence of cultivar and storage of chicory (Cichorium intybus L.) plants on polyphenol composition and antioxidative potential. Czech Journal of Food Sciences, 32(1), 10–15. doi: 10.17221/520/2012-CJFS
- Taciak, B., Białasek, M., Braniewska, A., Sas, Z., Sawicka, P., Kiraga, Ł, … Król, M. (2018). Evaluation of phenotypic and functional stability of RAW 264.7 cell line through serial passages. PLOS ONE, 13(6), e0198943. doi: 10.1371/journal.pone.0198943
- Tang, K., Yu, Y., Zhu, L., Xu, P., Chen, J., Ma, J., … Wei, K. (2019). Hypoxia-reprogrammed tricarboxylic acid cycle promotes the growth of human breast tumorigenic cells. Oncogene, 38(44), 6970–6984. doi: 10.1038/s41388-019-0932-1
- Tietze, M. K., Wuestefeld, T., Paul, Y., Zender, L., Trautwein, C., Manns, M. P., & Kubicka, S. (2000). Iκbα gene therapy in tumor necrosis factor-α-and chemotherapy-mediated apoptosis of hepatocellular carcinomas. Cancer Gene Therapy, 7(10), 1315. doi: 10.1038/sj.cgt.7700247
- Tilg, H., Zmora, N., Adolph, T. E., & Elinav, E. (2020). The intestinal microbiota fuelling metabolic inflammation. Nature Reviews Immunology, 20, 40–54. doi: 10.1038/s41577-019-0198-4
- Wong, E. T., & Tergaonkar, V. (2009). Roles of NF-κB in health and disease: Mechanisms and therapeutic potential. Clinical Science, 116(6), 451–465. doi: 10.1042/CS20080502
- Yang, G.-Y., Liao, J., Kim, K., Yurkow, E. J., & Yang, C. S. (1998). Inhibition of growth and induction of apoptosis in human cancer cell lines by tea polyphenols. Carcinogenesis, 19(4), 611–616. doi: 10.1093/carcin/19.4.611
- Yang, G., Kyoung Seo, E., Lee, J. H., & Young Lee, J. (2015). Suppression of splenic lymphocyte proliferation by Eucommia ulmoides and genipin. Chemistry & Biodiversity, 12(4), 538–546. doi: 10.1002/cbdv.201400376
- Yuan, F., Chen, J., Sun, P.-P., Guan, S., & Xu, J. (2013). Wedelolactone inhibits LPS-induced pro-inflammation via NF-kappaB pathway in RAW 264.7 cells. Journal of Biomedical Science, 20(1), 84. doi: 10.1186/1423-0127-20-84
- Yuandani, I. J., Ilangkovan, M., Husain, K., & Chan, K. M. (2016). Inhibitory effects of compounds from Phyllanthus amarus on nitric oxide production, lymphocyte proliferation, and cytokine release from phagocytes. Drug Design, Development and Therapy, 10, 1935.
- Zhang, H., Wu, M.-Y., Guo, D.-J., Wan, C.-W., Lau, C.-C., Chan, C.-O., … Chan, S.-W. (2013). Gui-ling-gao (turtle jelly), a traditional Chinese functional food, exerts anti-inflammatory effects by inhibiting iNOS and pro-inflammatory cytokine expressions in splenocytes isolated from BALB/c mice. Journal of Functional Foods, 5(2), 625–632. doi: 10.1016/j.jff.2013.01.004