ABSTRACT
We aimed to determine whether dietary supplementation with spray-dried porcine plasma (SDP) is effective in mice exposed to a dual S. aureus enterotoxin B (SEB) and lipopolysaccharide (LPS) challenges inducing simultaneous inflammation in the gut and lung. Male C57BL/6 mice were fed control or SDP-supplemented diets from weaning for 14 days. At the end of the experimental period, animals received LPS intranasal followed by SEB intraperitoneal doses. The dual challenge increased leukocyte recruitment into mesenteric lymph nodes and lung tissue, the percentage of activated monocytes and neutrophils, as well as the expression of Tnf-α, Ifn-γ and Il-1β in lung tissue and jejunal mucosa, while SDP attenuated these effects. Moreover, SDP augmented the expression of Il-10, Tgf-β and Foxp3 in these tissues. Supplementation with animal plasma proteins attenuated lung and intestinal inflammation provoked by simultaneous administration of LPS and SEB toxins and increased the expression of anti-inflammatory cytokines.
Introduction
Farm animals are exposed to high pathogenic pressure, which induces multiple sites of infection that increases morbidity. In fact, the presence of several pathogens in farms causes an increase of post-weaning mortality with significant impact on pig production worldwide (Adewole et al., Citation2016; Wellenberg et al., Citation2004). The different kind of pathogenic microorganisms can enter into the host for different mucosal areas (mainly respiratory and gastrointestinal). Diarrhoea remains a major cause of morbidity and mortality in livestock and is one of the most common diseases in suckling and weaning piglets globally. Pathogenic E. coli strains are common agents causing a variety of intestinal disorders in neonatal and weaning piglets, which may be followed by terminal septicaemia, an important cause of economic loss for swine producers (Lee et al., Citation2008). Other bacteria involved in farm infections are S. aureus, which increases mortality in farms (Osadebe et al., Citation2013), furthermore, other pathogens like Mycoplasma hyopenumoniare or reproductive and respiratory syndrome virus (PRRSV) contribute to respiratory disease and impact significantly in economic losses to the swine industry (Cornelison et al., Citation2018; Helme et al., Citation2018).
Spray-dried plasma (SDP) supplement is more effective in a high pathogenic environment (Coffey & Cromwell, Citation1995), enhancing its performance and reducing mortality in piglets reared in a conventional environment compared with a clean environment (Coffey & Cromwell, Citation1995).
Dietary SDP supplementation has anti-inflammatory effects in an acute intestinal inflammatory model induced by S. aureus enterotoxin B in rodents (SEB; Moretó & Pérez-Bosque, Citation2009; Pérez-Bosque et al., Citation2016). SEB stimulates clonal expansion of T cells, leading mainly to the production of T helper 1 (Th1)-cytokines such as IL-2 and interferon (IFN)-γ, among other effector molecules (Fraser & Proft, Citation2008). Moreover, the addition of SDP to drinking water reduced mortality in turkeys exposed to a respiratory challenge with Pasteurella multocida (Campbell et al., Citation2004), and oral SDP was able to modulate lung inflammatory responses induced by a lipopolysaccharide (LPS) challenge in mice (Maijó et al., Citation2012a, Citation2012b). LPS stimulates the synthesis and release of pro-inflammatory cytokines by macrophages and monocytes, leading to increased concentrations of interleukin (IL)-1β, IL-6, and tumour-necrosis factor (TNF)-α (Beutler et al., Citation2003). Moreover, SDP supplementation increased the pregnancy rate in stressed mice by reducing uterine mucosal inflammation (Song et al., Citation2015). These results indicate that changes induced by luminal SDP in gut-associated lymphoid tissue (GALT) influence the immune response in other mucosal areas exposed to bacterial toxin and environmental challenges. However, in all these models, the animals were subjected to a single insult, while farm animals are exposed to a several pathogens simultaneously, which induces multiple sites of infection.
The aim of the present study was to assess whether animal plasma preparations are effective in mice exposed to a dual challenge, inducing simultaneous inflammation in both the gut and lung mucosae.
Material and methods
Ethics statement
All animal experiments were carried out in strict adherence to the Guide for the Care and Use of Laboratory Animals and were approved by the Ethics Committee for Animal Experimentation of the University of Barcelona and the Catalan government (ref. 165/10 and 5414, respectively).
Animals and diets
Male C57BL/6 mice were provided by Envigo (Bresso, Italy) and kept at the animal facilities of the Facultat de Farmàcia i Ciències de l’Alimentació de la Universitat de Barcelona under conditions of constant temperature and humidity, with a 12h:12 h light/dark cycle. Animals were weaned on day 19 after birth and randomly distributed into two groups and fed the experimental diets (control and SDP; ) until day 33. Mice were monitored for food intake and body weight throughout the experimental period.
Table 1. Composition of experimental diets.
SDP is a feed ingredient obtained by centrifuging blood from healthy pigs and spray-drying to obtain a stable powder. The maintenance of the native protein was confirmed by immuno-electrophoresis and Western blot (Borg et al., Citation2002). Diets were balanced for energy and total nitrogen, and lysine and methionine were formulated to meet the National Research Council requirements (NRC, Citation1995; nutrient requirements) for laboratory animals. The nitrogen content of the control diet was adjusted with milk protein. All diets were prepared by APC-Europe, S.L.U. (Granollers, Spain).
Experimental design
The animals were exposed to different inflammatory conditions (LPS, SEB and LPS-SEB, or vehicle; ). The dual challenge (LPS-SEB) consisted of intranasal administration of 12.5 μg LPS from E. coli (O128:B12, Sigma-Aldrich, Saint Louis, MI, USA) and intraperitoneal injection of 25 μg SEB (Toxin Technology Inc., Sarasota, FL, USA), both dissolved in PBS. Non-challenged animals received PBS (vehicle). Mice were anaesthetized as previously described (Maijó et al., Citation2012a). On day 32, the mice were administered LPS and 6 h later they were challenged with SEB. The LPS-treated animals (LPS) received only the lipopolysaccharide challenge and the SEB-treated animals (SEB) received only the enterotoxin challenge. On day 33, blood was drawn by cardiac puncture under anaesthesia and the animals were killed by cervical dislocation at the end of the experiment.
Figure 1. Experimental design. Mice were fed with the experimental diets for 14 days starting at weaning. At age 32 d, animals received an intranasal dose of LPS (12.5 µg) and 6 h after, they were administered an intraperitoneal dose of SEB (25 µg). Animals were killed 24 h after LPS administration.
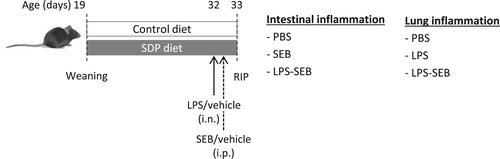
Mesenteric lymph node cell isolation
Leukocytes from MLN were obtained as previously described (Pérez-Bosque et al., Citation2016). Cell viability was higher than 85%.
Bronchoalveolar lymphocytes (BALF)
Bronchoalveolar lavage was performed by intra-tracheal instillation of 1 mL PBS (Woolard et al., Citation2005). BALF was obtained as previously explained (Maijó et al., Citation2012a). The cells were counted, and viability was determined with a solution of acridine orange and ethidium bromide. In all cases, cell viability was higher than 95%.
Cell staining
3 × 105 cells were incubated with primary mouse monoclonal antibodies for 30 min at 4°C, as previously described (Pérez-Bosque et al., Citation2016). The following primary antibodies were used: anti-CD45 APC-A750 (eBiosciences, San Diego, CA, USA) for leukocytes, lymphocytes, anti-CD68 A700 (eBiosciences, San Diego, CA, USA) for monocytes, anti-Ly6G FITC (BD Pharmingen, Franklin Lakes, NJ, USA) for neutrophils and anti-CD14 PE (eBiosciences, San Diego, CA, USA) for activated neutrophils/monocytes and isotype control. Then, the cells were washed and maintained in paraformaldehyde 4% until further analysis in the Aria cell sorter (Becton Dickinson, Franklin Lakes, NJ, USA) located at the Cytometry Unit of the Technical Services of the University of Barcelona at the Barcelona Science Park. The results were analysed using the Flowjo Software (Treestar Inc., Ashland, OR, USA).
Jejunum and lung cytokine determination
Total RNA was extracted with TRIzol reagent (Life Technologies, Carlsbad, CA, USA) following the manufacturer’s instructions. RNA extraction and retrotranscription were carried out as previously described (Miró et al., Citation2017). The mouse primers used are listed in . Real-time PCR was performed using a cDNA template in a 20 µL reaction containing 0.2 μmol/L of each primer and SsoAdvanced™ Universal SYBR® Green Supermix (Bio-Rad, Hercules, CA, USA) and carried out on a MiniOpticon Real-Time PCR System (Bio-Rad, Hercules, CA, USA). Samples were tested in duplicate and average values were used for the quantification, which was carried out with the 2−ΔΔCT method (Schmittgen & Livak, Citation2008). To confirm PCR amplification of the intended product, representative samples were analysed by electrophoresis on a 4% agarose gel. Product fidelity was confirmed by melt-curve analysis.
Table 2. Primers used for real-time PCR.
Statistical analysis
The results of the experiments are presented as the mean ± SEM. The data were analysed by two-way ANOVA followed by Fisher’s least significant difference post-hoc test, using GraphPad Prism® software (version 6, GraphPad Software, Inc., La Jolla, CA, USA). The factors considered were diet (control and SDP-supplemented feed) and inflammatory conditions (vehicle, SEB or LPS alone and LPS-SEB). Differences were considered significant at P < .05.
Results
Effects of SEB challenge versus dual challenge (LPS-SEB) in intestinal mucosa
The administration of SEB alone showed a slight effect on cell recruitment into the MLN, while the LPS and SEB challenges doubled the cell number (P = .002 and P = .001, respectively; (a)). SDP supplementation reduced the number of cells recruited into MLN (P = .036) in both inflammatory conditions, but had no effect on this variable in non-challenged animals (Interaction, Int. P = .047). The administration of either SEB alone or both toxins (LPS-SEB) increased the percentage of activated monocytes and neutrophils in MLN (all P < .01; (b,c)), although not in the same magnitude. SDP reduced the percentage of activated monocytes in all conditions (P = .001). SDP clearly reduced the number of activated neutrophils (P = .006), but without changing the percentage of this population in non-challenged mice (Int. P = .008).
Figure 2. Effects of SDP supplementation on immune cells in mesenteric lymph nodes (MLN) after SEB or LPS-SEB administration. Panel (a) shows leukocyte recruitment into MLN. Panels (b) and (c) show the percentage of activated monocytes and activated neutrophils, respectively. Between inflammatory conditions, means without a common letter differ, P < .05. Results are expressed as mean ± SEM (n = 8). Act., activated; Int., interaction between both factors (challenge and diet).
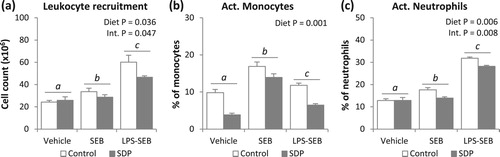
Both inflammatory conditions (SEB and LPS-SEB) increased the expression of Tnf-α, Ifn-γ and Il-1β in intestinal mucosa (all P < .001; (a–c)). The SDP diet reduced the expression of this pro-inflammatory cytokine in challenged animals (all P < .005). In Tnf-α and Il-1β expression, there was an interaction between diet and inflammatory condition (Int. P < .001 and P = .043, respectively). Dual challenge increased mucosal expression of Il-10 (P = .015; (d)), but had no effect on the expression of Tgf-β ((e)). In contrast, SEB alone did not modify the intestinal expression of any of the anti-inflammatory cytokines analysed (Il-10 and Tgf-β). Supplementation with SDP increased Il-10 expression (P = .001) but only in challenged animals (Int. P = .001), while in Tgf-β, the supplement increased expression in both challenged and non-challenged animals (P = .001). In animals challenged with SEB and with the dual challenge (LPS-SEB), Foxp3 expression was decreased (P = .004, (f)). In both inflammatory conditions and in non-challenged mice, dietary SDP supplementation augmented Foxp3 expression (P = .001).
Figure 3. Effects of SDP supplementation on cytokine and transcription factor expression in jejunum mucosa after SEB or LPS-SEB administration. Panels (a) to (f) show the expression of Tnf-α, Ifn-γ, Il-1β, Il-10, Tgf-β and Foxp3, respectively. Between inflammatory conditions, means without a common letter differ, P < .05. Results are expressed as mean ± SEM (n = 8). Int., interaction between both factors (challenge and diet).
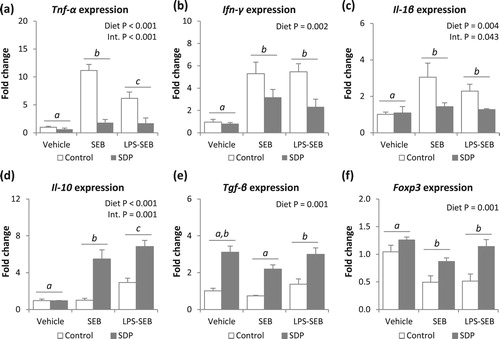
Effects of LPS challenge versus dual challenge (LPS-SEB) in lung tissue
The administration of LPS alone or LPS-SEB increased leukocyte recruitment in lung tissue (both P < .005, (a)), and the percentage of activated monocytes and neutrophils (all P < .001, (b,c)). SDP supplementation reduced leukocyte recruitment (P =_ .027) in challenged animals (P = .017), as well as the percentage of activated monocytes and neutrophils (both P = .004).
Figure 4. Effects of SDP supplementation on immune cells in lung tissue after LPS or LPS-SEB administration. Panel (a) shows leukocyte recruitment into MLN. Panels (b) and (c) show the percentage of activated monocytes and activated neutrophils, respectively. Between inflammatory conditions, means without a common letter differ, P < .05. Results are expressed as mean ± SEM (n = 8). Act., activated; Int., interaction between both factors (challenge and diet).
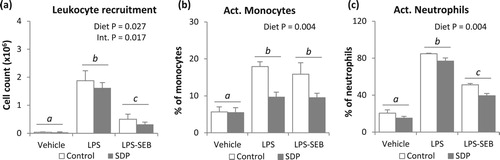
Both challenge with LPS or LPS and SEB increased mucosal expression of pro-inflammatory cytokines (Tnf-α, Ifn-γ and Il-1β, all P < .005, (a–c)). Moreover, mice challenged with LPS alone showed higher Ifn-γ expression than mice received both toxins (P = .016). The SDP diet reduced pro-inflammatory cytokine expression (P < .005, in all pro-inflammatory cytokines), but there was a significant interaction since SDP supplementation did not change the expression of Tnf-α, Ifn-γ and Il-1β in non-challenged mice (Int. P = .030, Int. P = .016 and Int. P = .012, respectively). The dual challenge, as well as SDP supplementation, increased lung Il-10 expression (P < .05 and P < .001, respectively; (d)), and there was also an interaction between the inflammatory condition and diet since SDP-supplemented animals undergoing the dual challenge showed higher Il-10 expression (Int. P = .006). In contrast, none of the inflammatory conditions (LPS alone or LPS-SEB) modified Tgf-β or Foxp3 expression ((e,f)). Moreover, animals fed the SDP supplementation increased expression of Tgf-β and Foxp3 in lung tissue in all groups (challenged and non-challenged conditions, P = .001 in both variables).
Figure 5. Effects of SDP supplementation on cytokine and transcription factor expression in lung tissue after LPS or LPS-SEB administration. Panels (a) to (f) show the expression of Tnf-α, Ifn-γ, Il-1β, Il-10, Tgf-β and Foxp3, respectively. Between inflammatory conditions, means without a common letter differ, P < .05. Results are expressed as mean ± SEM (n = 8). Int., interaction between both factors (challenge and diet).
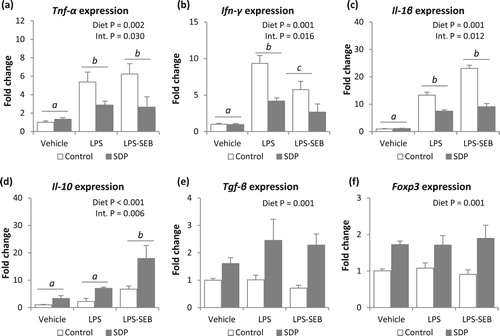
Discussion
Farm animals are exposed to multiple pathogens, which increase the rates of animal morbidity and mortality (Wellenberg et al., Citation2004). Dietary supplementation with SDP has anti-inflammatory effects in acute intestinal inflammation induced by SEB in rodents (Moretó & Pérez-Bosque, Citation2009; Pérez-Bosque et al., Citation2016), as well as in acute lung inflammation induced by LPS (Maijó et al., Citation2012a, Citation2012b). The aim of the present study was to assess whether animal plasma preparations are effective when mice are challenged simultaneously with both toxins SEB and LPS, inducing a concurrent inflammation in the gut and lung mucosae.
In the intestine, dual challenge induced higher leukocyte recruitment into MLN than SEB alone, indicating that LPS enhances the effects of SEB in mice, as found in other studies (Hudson Reichenberg et al., Citation2017). SEB alone, or administered together with LPS, increased the activation of monocytes and neutrophils from MLN. The minor effect of SEB administration alone on neutrophil activation is consistent with the low capacity of SEB to activate neutrophils, whose recruitment and activation is due to the release of pro-inflammatory mediators such as cytokines and chemokines (Desouza et al., Citation2006). SEB challenge alone, as well as dual challenge, increased the expression of pro-inflammatory cytokines several folds, indicating a general GALT activation (Pérez-Bosque et al., Citation2010). SDP supplementation reduced leukocyte recruitment into MLN, as well as monocyte and neutrophil activation in SEB-challenged mice, confirming previous results (Pérez-Bosque et al., Citation2016), and it was also effective in reducing intestinal inflammation induced by dual challenge. Moreover, plasma protein supplementation diminished the intestinal expression of pro-inflammatory cytokines, which is relevant since both TNF-α and IL-1β can induce neutrophilia and neutrophil activation (Dinarello, Citation2009). These effects of SDP reduce the magnitude of the inflammatory response and improve intestinal integrity (Moretó & Pérez-Bosque, Citation2009).
Regulatory Th lymphocytes (Treg) are responsible for maintaining immune homeostasis and suppressing intestinal inflammation resulting from intense immune responses to self-antigens and commensal bacteria (Ueno et al., Citation2013). The expression of Foxp3 is indispensable for Treg to carry out their anti-inflammatory function (Tang & Bluestone, Citation2008). SDP supplementation prevented the low Foxp3 expression found in mice challenged with either SEB or LPS-SEB. Similar effects of plasma protein supplements were observed in a colitis mouse model (Pérez-Bosque et al., Citation2016). The importance of these effects lies in the role of Treg in the suppression of cell activation and recruitment through the secretion of anti-inflammatory cytokines such as IL-10 and TGF-β (Wan & Flavell, Citation2008). Moreover, TGF-β also regulates tight-junction proteins and the permeability properties of the mucosa, which determine the severity of the inflammatory syndrome (Howe et al., Citation2005). Xiao et al. (Citation2016) also showed, in a model of LPS-induced intestinal inflammation, that a whey protein concentrate protects intestinal integrity via a mechanism that also involves TGF-β.
In lung tissue, LPS exposure increased leukocyte recruitment and neutrophil and monocyte activation in BALF, as reported earlier (Hakansson et al., Citation2012; Maijó et al., Citation2012a). The effects of the dual challenge on cell recruitment and neutrophil activation were lower than with the single LPS challenge, indicating a certain interference between the two immune responses. Moreover, consistent with what happens at the cellular level, LPS challenge strongly stimulated the expression of pro-inflammatory cytokines but these effects were not enhanced by a simultaneous SEB challenge (or were even reduced in the case of Ifn-γ expression). The intestinal inflammatory response following the dual (LPS-SEB) challenge was, in general, higher than the response observed when only SEB was administered. However, the lung inflammatory response after the dual challenge was similar (or even lower) than that observed following the single LPS challenge. One hypothetical reason might be that, at weaning, the mucosal immune system is still immature (Bailey et al., Citation2005), and therefore weaned animals might be unable to develop efficient immune responses in different mucosal areas, leaving the mucosal interface slightly immunocompromised. The deregulation of the effector mechanisms of immune responses frequently results in tissue inflammation and damage (Bailey et al., Citation2005). In this regard, SDP promotion of mucosal immune regulation might explain why animals receiving plasma supplementation showed enhanced performance and lower mortality than non- supplemented animals in a high pathogenic environment (Coffey & Cromwell, Citation1995).
Plasma supplement reduced leukocyte recruitment as well as the activation of innate immunity in LPS-induced acute lung inflammation, consistent with the effects described by Maijó et al. (Citation2012a). The effects of SDP on lung leukocyte infiltration might explain the better performance and survival of turkey exposed to Pasteurella multocida (Campbell et al., Citation2004). Moreover, as observed in intestinal mucosa, SDP increased the expression of Il-10, Tgf-β and Foxp3 in mice challenged with either LPS alone or the dual challenge, which is consistent with previous research (Maijó et al., Citation2012b) and indicates that SDP effects are mediated by enhancing anti-inflammatory mediators.
Conclusions
SDP supplement modulates immune response by enhancing the expression of Il-10, Tgf-β and Foxp3. This promotes an “anti-inflammatory status” that lowers the magnitude of the inflammatory responses, allowing weaned animal to respond efficiently to multiple infection sites.
Disclosure statement
L. Miró and J. Polo are employed by APC-Europe SLU. C. Amat, M. Moretó, A. Pérez-Bosque have no conflicts of interests.
Additional information
Funding
References
- Adewole, D. I., Kim, I. H., & Nyachoti, C. M. (2016). Gut health of pigs: Challenge models and response criteria with a critical analysis of the effectiveness of selected feed additives – A review. Asian-Australasian Journal of Animal Sciences, 29, 909–924. https://doi.org/10.5713/ajas.15.0795
- Bailey, M., Haverson, K., Inman, C., & Harris, C. (2005). The development of the mucosal immune system pre- and post-weaning: Balancing regulatory and effector function. Proceedings of the Nutrition Society, 64, 451–457. https://doi.org/10.1079/PNS2005452
- Beutler, B., Hoebe, K., Du, X., & Ulevitch, R. J. (2003). How we detect microbes and respond to them: The Toll-like receptors and their transducers. Journal of Leukocyte Biology, 74, 479–485. https://doi.org/10.1189/jlb.0203082
- Borg, B. S., Campbell, J. M., Polo, J., Russell, L. E., Rodríguez, C., & Ródenas, J. (2002). Evaluation of the chemical and biological characteristics of spray-dried plasma protein collected from various locations around the world. American Association of Swine Veterinarians, 33, 97–100.
- Campbell, J. M., Quigley, J. D., 3rd, & Russell, L. E. (2004). Impact of spray-dried bovine serum and environment on Turkey performance. Poultry Science, 83, 1683–1687. https://doi.org/10.1093/ps/83.10.1683
- Coffey, R. D., & Cromwell, G. L. (1995). The impact of environment and antimicrobial agents on the growth response of early-weaned pigs to spray-dried porcine plasma. Journal of Animal Science, 73, 2532–2539. doi: 10.2527/1995.7392532x
- Cornelison, A. S., Karriker, L. A., Williams, N. H., Haber, B. J., Stalder, K. J., Schulz, L. L., & Patience, J. F. (2018). Impact of health challenges on pig growth performance, carcass characteristics, and net returns under commercial conditions. Translational Animal Science, 2, 50–61. https://doi.org/10.1093/tas/txx005
- Desouza, I. A., Franco-Penteado, C. F., Camargo, E. A., Lima, C. S., Teixeira, S. A., Muscará, M. N., De Nucci, G., & Antunes, E. (2006). Acute pulmonary inflammation induced by exposure of the airways to staphylococcal enterotoxin type B in rats. Toxicology and Applied Pharmacology, 217, 107–113. https://doi.org/10.1016/j.taap.2006.07.001
- Dinarello, C. A. (2009). Inflammation in human disease: Anticytokine therapy. Biology of Blood and Marrow Transplantation, 15, 134–136. https://doi.org/10.1016/j.bbmt.2008.11.006
- Fraser, J. D., & Proft, T. (2008). The bacterial superantigen and superantigen-like proteins. Immunological Reviews, 225, 226–243. https://doi.org/10.1111/j.1600-065X.2008.00681.x
- Hakansson, H. F., Smailagic, A., Brunmark, C., Miller-Larsson, A., & Lal, H. (2012). Altered lung function relates to inflammation in an acute LPS mouse model. Pulmonary Pharmacology & Therapeutics, 25, 399–406. https://doi.org/10.1016/j.pupt.2012.08.001
- Helme, E. T., Outhouse, A. C., Schwartz, K. J., Lonergan, S. M., Curry, S. M., Dekkers, J. C. M., & Gabler, N. K. (2018). Metabolic adaptation of pigs to a Mycoplasma hyopneumoniae and Lawsonia intracellularis dual challenge. Journal of Animal Science, 96, 3196–3207. https://doi.org/10.1093/jas/sky220
- Howe, K. L., Reardon, C., Wang, A., Nazli, A., & McKay, D. M. (2005). Transforming growth factor-β regulation of epithelial tight junction proteins enhances barrier function and blocks enterohemorrhagic Escherichia coli O157:H7-induced increased permeability. American Journal of Pathology, 167, 1587–1597. https://doi.org/10.1016/S0002-9440(10)61243-6
- Hudson Reichenberg, L. C., Garg, R., Fernalld, R., Bost, K. L., & Piller, K. J. (2017). Systemic cytokine and chemokine responses in immunized mice challenged with staphylococcal enterotoxin B. Toxicon, 133, 82–90. https://doi.org/10.1016/j.toxicon.2017.05.005
- Lee, S. H., Shinde, P., Choi, J., Park, M., Ohh, S., Kwon, I. K., Pak, S. I., & Chae, B. J. (2008). Effects of dietary iron levels on growth performance, hematological status, liver mineral concentration, fecal microflora, and diarrhea incidence in weanling pigs. Biological Trace Element Research, 126(Suppl 1), S57–S68. https://doi.org/10.1007/s12011-008-8209-5
- Maijó, M., Miró, L., Polo, J., Campbell, J., Russell, L., Crenshaw, J., Weaver, E., Moretó, M., & Pérez-Bosque, A. (2012a). Dietary plasma proteins attenuate the innate immunity response in a mouse model of acute lung injury. British Journal of Nutrition, 107, 867–875. https://doi.org/10.1017/S0007114511003655
- Maijó, M., Miró, L., Polo, J., Campbell, J., Russell, L., Crenshaw, J., Weaver, E., Moretó, M., & Pérez-Bosque, A. (2012b). Dietary plasma proteins modulate the adaptive immune response in mice with acute lung inflammation. Journal of Nutrition, 142, 264–270. https://doi.org/10.3945/jn.111.149070
- Miró, L., Garcia-Just, A., Amat, C., Polo, J., Moretó, M., & Pérez-Bosque, A. (2017). Dietary animal plasma proteins improve the intestinal immune response in senescent mice. Nutrients, 9(12), pii: E1346. https://doi.org/10.3390/nu9121346
- Moretó, M., & Pérez-Bosque, A. (2009). Dietary plasma proteins, the intestinal immune system, and the barrier functions of the intestinal mucosa. Journal of Animal Science, 87(14 Suppl), E92–E100. https://doi.org/10.2527/jas.2008-1381
- National Research Council. (1995). Nutrient requirements of laboratory animals (4th ed). National Academies Press.
- Osadebe, L. U., Hanson, B., Smith, T. C., & Heimer, R. (2013). Prevalence and characteristics of Staphylococcus aureus in Connecticut swine and swine farmers. Zoonoses and Public Health, 60, 234–243. https://doi.org/10.1111/j.1863-2378.2012.01527.x
- Pérez-Bosque, A., Miró, L., Amat, C., Polo, J., & Moretó, M. (2016). The anti-inflammatory effect of spray-dried plasma is mediated by a reduction in mucosal lymphocyte activation and infiltration in a mouse model of intestinal inflammation. Nutrients, 8, E657. https://doi.org/10.3390/nu8100657
- Pérez-Bosque, A., Miró, L., Polo, J., Russell, L., Campbell, J., Weaver, E., Crenshaw, J., & Moretó, M. (2010). Dietary plasma protein supplements prevent the release of mucosal proinflammatory mediators in intestinal inflammation in rats. Journal of Nutrition, 140, 25–30. https://doi.org/10.3945/jn.109.112466
- Schmittgen, T. D., & Livak, K. J. (2008). Analyzing real-time PCR data by the comparative CT method. Nature Protocols, 3, 1101–1108. https://doi.org/10.1038/nprot.2008.73
- Song, M., Liu, Y., Lee, J. J., Che, T. M., Soares-Almeida, J. A., Chun, J. L., … Pettigrew, J. E. (2015). Spray-dried plasma attenuates inflammation and improves pregnancy rate of mated female mice. Journal of Animal Science, 93, 298–305. https://doi.org/10.2527/jas.2014-7259
- Tang, Q., & Bluestone, J. A. (2008). The Foxp3+ regulatory T cell: A jack of all trades, master of regulation. Nature Immunology, 9, 239–244. https://doi.org/10.1038/ni1572
- Ueno, A., Jijon, H., Chan, R., Ford, K., Hirota, C., Kaplan, G. G., Beck, P. L., Lacucci, M., Gasia, M. F., Barkema, H. W., Panaccione, R., & Ghosh, S. (2013). Increased prevalence of circulating novel IL-17 secreting Foxp3 expressing CD4+ T cells and defective suppressive function of circulating Foxp3+ regulatory cells support plasticity between Th17 and regulatory T cells in inflammatory bowel disease patients. Inflammatory Bowel Diseases, 19, 2522–2534. https://doi.org/10.1097/MIB.0b013e3182a85709
- Wan, Y. Y., & Flavell, R. A. (2008). TGF-beta and regulatory T cell in immunity and autoimmunity. Journal of Clinical Immunology, 28, 647–659. https://doi.org/10.1007/s10875-008-9251-y
- Wellenberg, G. J., Stockhofe-Zurwieden, N., Boersma, W. J., De Jong, M. F., & Elbers, A. R. (2004). The presence of co-infections in pigs with clinical signs of PMWS in The Netherlands: A case-control study. Research in Veterinary Science, 77, 177–184. https://doi.org/10.1016/j.rvsc.2004.03.007
- Woolard, M. D., Hudig, D., Tabor, L., Ivey, J. A., & Simecka, J. W. (2005). NK cells in gamma-interferon-deficient mice suppress lung innate immunity against Mycoplasma spp. Infection and Immunity, 73, 6742–6751. https://doi.org/10.1128/IAI.73.10.6742-6751.2005
- Xiao, K., Jiao, L., Cao, S., Song, Z., Hu, C., & Han, X. (2016). Whey protein concentrate enhances intestinal integrity and influences transforming growth factor-β1 and mitogen-activated protein kinase signalling pathways in piglets after lipopolysaccharide challenge. British Journal of Nutrition, 115, 984–993. https://doi.org/10.1017/S0007114515005085