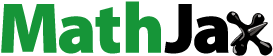
ABSTRACT
A monoclonal antibody against florfenicol amine (FFA) was produced, and a competitive enzyme-linked immunosorbent assay (ELISA) was developed for detecting florfenicol (FF) and FFA in eggs. This proposed method exhibited the 50% inhibiting concentrations (IC50) of 0.120 ng/mL for FF and 0.118 ng/mL for FFA under the optimization of experimental parameters. At the fortified levels of 0.4, 0.8, 2.4, and 30.0 μg/kg, the inter-assay recoveries of the target compounds ranged from 78.3 to 103.2%, and the intra-assay recoveries ranged from 81.5 to 104.1%, respectively. The coefficients of variation were all below 12%. Results for the determination of spiked samples by this ELISA showed a good relationship with the high-performance liquid chromatography (HPLC) technique. This newly developed method confirmed to be rapid, user-friendly, and sensitivity for simultaneous detecting FF and FFA in eggs.
Introduction
Florfenicol (FF) is an antibiotic of amphenicol family; the other two are chloramphenicol (CAP) and thiamphenicol (TAP) (Guidi et al., Citation2017). FF has a similar structure with CAP, which had once been widely utilized for therapeutic and prophylactic purposes. CAP had been prohibited by the European Union and many countries because of its adverse effect on human health. FF was introduced as a preferable substance since its obvious advantages including better activity against chloramphenicol-resisted strains, relatively high bioactivity in some species, and the effective treatment for bovine and porcine respiratory disease (Samsonova et al., Citation2012). FF was extensively employed in poultry, livestock and aquaculture industry. Previous studies had indicated that FF could convert to FFA after administration (Schwarz et al., Citation2004). Although the ratio of them varies in different foodstuffs of animal origin, FFA was always regarded as the main metabolite of FF for analysis (Saito-Shida et al., Citation2019; Santos & Ramos, Citation2018; Schwarz et al., Citation2004). The ADI of FF was 0–10 μg/kg.bw, which was issued by JECFA (Samsonova et al., Citation2012), and the MRLs had also been established as the sum of FF and FFA by different countries and organizations, ranging from 10 to 3700 μg/kg (Saito-Shida et al., Citation2019).
Some research was carried out to examine the occurrence of amphenicol drug residue in animal-producing foodstuffs (Imran et al., Citation2017). Although FF had been banned from applied in egg-laying animal, the occurrence of FF could still be observed in egg samples (Guidi et al., Citation2017; Wang et al., Citation2019; Xie et al., Citation2011; Xie et al., Citation2018). The level of FF in egg was from 1.7 to 2.5 μg/kg from the report from Spain (Azzouz & Ballesteros, Citation2015) and until now the highest levels of FF and FFA in eggs were 19 and 36 μg/kg in China, respectively (Guidi et al., Citation2017). The results of monitoring plans released by the Chinese State Administration for Market Regulation and local governments of many provinces in China also showed the FF residue in many batches of egg samples (Wang et al., Citation2019), which indicated the potential excessive and inappropriate administration of this drug. As a result, it is crucial to develop analytical methods with ideal selectivity and sensitivity for effective controlling of FF in concern foodstuffs (Qiao et al., Citation2018).
Previously, many physicochemical analysis methods had been developed for FF and its metabolite determination, such as liquid chromatography (LC) (Dasenaki & Thomaidis, Citation2015; Imran et al., Citation2017; Saito-Shida et al., Citation2019; Xie et al., Citation2018), gas chromatography (GC) (Shen et al., Citation2009) (Azzouz & Ballesteros, Citation2015), and high-performance liquid chromatography (HPLC) (Filazi et al., Citation2014; Wang et al., Citation2016; Wang et al., Citation2019; Xie et al., Citation2011) combined with different detector systems. Among these methods, HPLC was the most frequently applied technique partly due to its high sensitivity and selectivity. Despite the advantages of the above determination techniques, they are time-consuming and always require expensive instruments. Besides, they are not suitable for one-site monitoring purposes and tackling high-throughput samples.
Recently, increasing research has been focused on immunological methods for the detection of amphenicol family members, encompassing conventional methods and novel designed methodologies with optimized modifications (Samsonova et al., Citation2012). Wu et al. (Citation2008) applied a polyclonal antibody-based competitive enzyme-linked immunosorbent assay (ELISA) to detect FFA in animal edible tissues. Pengjie Luo et al. established a heterologous ELISA for the simultaneous determination of FF and FFA in swine muscle (Luo et al., Citation2009). An ELISA method based on the monoclonal antibody was established for FF detection in fish feed (Pengjie Luo et al., Citation2009). One study conducted by Fodey et al. (Citation2013) has employed immunochemical methods to determine TAP, FF, and FFA with satisfactory sensitivity. An indirect ELISA has also been introduced for the simultaneous detection of FF and TAP in edible animal tissue, with a satisfactory correlation between the ELSIA method and HPLC-MS/MS method (An et al., Citation2016). In another study (Li et al., Citation2018), a polyclonal antibody was obtained for the establishment of an ic-ELISA. FF and TAP were analysed in animal meats and urine, with LOD of 0.12 ng/mL for FF, and 0.15 ng/mL for TAP. In the latest report which focused on the determination of amphenicol family members in eggs (Lei et al., Citation2017), a convenient and sensitive ic-ELISA technique was established with the appropriate performance criteria. Recently, the lateral flow immunoassay (LFA) combined with fluorescent microspheres (FMs) was designed for the qualification of FF, TAP, and CAP in milk (Wang et al., Citation2017). However, no immunoassay technique for the simultaneous detection of FF and FFA in eggs has been reported. This study was set out to design the novel haptens of FF and FFA, and then establish a reliable ELISA for the determination of the target compounds in eggs. The proposed method was also compared with the HPLC method and it exhibited satisfactory sensitivity, specificity, accuracy, and reproducibility when analysing FF and FFA in incurred samples.
Reagents
All chemical reagents involved were of analytical grade. N,N-dicyclohexylcarbodiimide (DCC), N-hydroxysuccinamide (NHS), N,N-dimethylformamide (DMF), Freund’s complete adjuvant (FCA), Freund’s incomplete adjuvant (FIA), bovine serum albumin (BSA), ovalbumin (OVA), tetramethylbenzidine (TMB), Formaldehyde (F), Trolamine (TEOA) were all from Acros Organics (Worcester, MA, USA). FF, FFA, TAP, and CAP were from Schering-Plough Corp. Tween 20, gelatine, and methanol were from Sinopharm Chemical Reagent Co. Ltd (Beijing, China). The horseradish peroxidase (HRP) conjugate of goat anti-mouse IgG was from Sigma-Aldrich. Other regents were obtained from Beijing Chemical Reagent Co. (Beijing, China).
Instruments
The equipment of microplate absorbance reader was from Thermo Inc. (Beijing, China). Cell culture plates and microtiter plates were from Costar Inc. (Cambridge, MA, USA).
Working solutions
Carbonate buffer solution (0.05 mol/L, pH 9.6) was utilized as the coating buffer. Phosphate-buffered solution (PBS, pH 7.4, 0.01 mol/L) contained 8.0 g NaCl, 0.2 g KCl, 0.2 g KH2PO4, 2.9 g Na2HPO4·12H2O and 1 L of distilled water. Assay buffer (PBS1, pH 7.2) was a phosphate buffer solution of 0.1 mol/L, containing 5.5 g of NaH2PO4·2H2O, 25.8 g of Na2HPO4·12H2O, and 12.5 g of NaCl in 1 L of distilled water. Blocking buffer mixture was prepared with the PBS solution and 0.9% casein. Tween 20 was diluted to 0.06% (v/v) in PBS for producing PBST as washing solution. HRP-IgG solution was comprised of 5% fetal bovine serum with PBS. Sample extraction buffer (PBS2) was prepared with 0.02 mol/L of PB, containing 0.15 mol/L NaCl and 0.062 mol/L trichloroacetic acid. Substrate buffer was made with 0.1 mol/L citrate buffer with the pH value of 5.5. The HRP substrate buffer was made by 1 mL of substrate buffer solution, 200 μL of 1% mixture of TMB diluted in dimethyl sulphoxide, and 220 μL of 2% urea hydrogen peroxide dissolved in 20 mL of deionized water. The stop solution was a solution of 2 mol/L H2SO4.
Synthesis of artificial antigen
As we all know, hapten heterology was better than homologous assay (Luo et al., Citation2011). FFA-BSA and FF-OVA were synthesized as the immunogen and coating antigen in this study, respectively (). The formaldehyde coupling method was applied for the production of FFA-BSA immunogen, which was consistent with the previous study conducted by Pengjie Luo et al. (Citation2009). 50 mg FFA (0.202 mmol) and 150 mg BSA (0.0022 mmol) were mixed and stirred with 10 mL of PBS and 2 mL of DMF, with a pH value of 6.5. 3 mL of 1% formaldehyde solution was poured into the mixture followed by mixing at room temperature for 6 min and dialyzed under circumstances of PBS for 3 days at 4°C. 100 mg of FF and 15 mL of 4-(chlorocarbonyl)benzoic acid were dissolved in 3 mL of DMF, and 4 mL of TEOA was added to the mixture at 0°C and reacted for 1 h. The solution of sodium hydrogen carbonate was added for the quenching of the reaction. The compounds were concentrated and evaporated under reduced pressure. FF hapten was obtained. 100 μL FF hapten, 10 mg of NHS, and 20 mg of DCC were all dissolved in 500 μL of DMF. The solution was gently mixed for 8 h at room temperature in dark place. The above compound was added dropwise to 10% DMF solution containing 40 mg of OVA, and stirred for 1 h. The solution was separated by dialysis against 0.01 mol/L of PBS for 2 days at 4°C. The final derivatives were stored at −20°Cfor further use.
Production of antibodies
Monoclonal antibody toward FFA was produced by the injection of FFA-BSA into six female BALB/c mice with the age from 6 to 8 weeks at the total amount of 80 mg/kg of body weight (BW). Preliminarily, 100 μg of FFA-BSA was mixed with 1 mL of NaCl (0.9%) and emulsified in the solution of FCA (1:1, v/v) completely. The solution was injected into mice back at multi-sites. 50 μg of immunogen emulsified in FIA was applied for subsequent booster immunizations every two weeks. The titre of the antibody was examined by the ELISA method. Three days before the cell fusion, the final immunization was performed at the dosage of 20 μg via caudal vein. The mouse was exsanguinated and the serum with highest titre was harvested by coagulation and centrifugation, then stored at −20°C until application. The splenocytes were obtained from spleens and were fused with cell lines of SP2/0 myeloma by the polyethylene glycol (PEG) method. After fusion, the cells were screened by culturing in HAT and HT medium. After positive hybridoma cells detected, the positive reaction between the hybridomas and FFA was subcloned by conducting the limiting dilution procedure. The applicable cell lines were cultured for expansion and injected into the mice for the production of ascites. The collection and purification of supernatant of the positive hybridomas was performed by the two-step methods: caprylic acid and ammonium sulphate precipitation. The proposed technique had been reported with some modifications (An et al., Citation2016; Lei et al., Citation2017; Wu et al., Citation2008).
ELISA optimization
An ELISA could be optimized by checkerboard method for the preferable concentration of coating antigen, monoclonal antibody, and other immunoreagents. Other experimental parameters, such as the pH values, ionic strengths, and sample volume, had been optimized. These performance characteristic introduced for the evaluation of the method performance was IC50 (level at which connecting antibody to coating antigen is inhibited by 50%), which was used for evaluating the sensitivity of ELISA.
Indirect competitive ELISA
The microplate was prepared with 100 μL/well FF-OVA at the dilution fold of 1:20,000, at 25°C for 2 h. After incubation, PBS containing 0.06% Tween 20 (PBST) was applied to wash the plate for 4 times, followed by the blocking buffer (150 μL/well) for 1 h at 25°C. 50 μL of analytical solution and 50 μL of 1:20,000 antibody dilution were added to each well, followed by 100 μL/well of enzyme tracer HRP-IgG solution (1:1000 in PBS). The plate was incubated for 15 min at room temperature, the solution was aspirated and the plated was washed 4 times, then 100 μL of the substrate solution was added to each well. After incubation for 5 min, the colour development was inhibited by adding 50 μL of stop solution to each well, and all the absorbance values were detected at 450 nm.
Cross-reactivity
Cross-reactivity (CR) was detected by performing the competitive assays. The values of CR for FFA, FF, TAP, and CAP were calculated by the following equation:(1)
(1)
Sample preparation
The standard solutions at different levels were added to samples free of FF and FFA. 2.0 g of spiked sample was extracted with 8 mL of PBS2 and was homogenized on a Vortex mixer for 3 min, then centrifuged at 3500 g for 6 min. The supernatant was removed and diluted 5-fold in the assay buffer before further detection.
Comparison between the ELISA and HPLC
The experimental characteristics of newly developed ELISA were compared with those of the HPLC technique (An et al., Citation2016). The egg samples were examined at the six spiked levels (0.4, 0.5, 2.0, 10.0, 30.0, and 50.0 μg/kg) in triplicate at each concentration, and simultaneously detected by the ELISA procedure and HPLC method. The ELISA analysis was undertaken as described above. The HPLC method was performed on the basis of procedures performed by the former literature with some modifications (Filazi et al., Citation2014; Xie et al., Citation2011).
Results and discussion
Design of immunogen and coating antigen
It is well accepted that antibody is a key element in the experimental design, which allows high sensitivity and selectivity of immunological analysis (Samsonova et al., Citation2012). Since FF and FFA are low-weight moleculars, different types of haptens had been designed against FF and FFA. It was hypothesized that the methylsulfonylphenyl and fluorine moieties were the key groups for the FF analysis (Luo et al., Citation2011; Song et al., Citation2010). The complexity and rigidity of the hapten should be taken into account, such as heterocyclic chemicals, benzene ring, branch structure, length of linking spacer, and the proper exposure of the hapten. FFA-BSA was made by the formaldehyde coupling method, in which formaldehyde-facilitated reaction occured primarily by the immonium ion pathway to link amine groups. FF was selected as material, and 4-(chlorocarbonyl) benzoic acid was introduced to FF for the synthesis of the novel haptens. Since the active group of the FFA and FF derivatives was the carboxyl group, the conjugation with carrier protein was conducted through traditional active ester method (Samsonova et al., Citation2012; Song et al., Citation2010). After coupled with protein, the immunogen still maintained the entire immunodominant positions of hapten and obtained the capacity to produce high affinity and specificity antibody.
Optimization of immunoassay
There is now a general consensus that levels and dilution folds of the antibody and coating antigen, pH values and ionic composition of assay buffer play a significantly effect on the sensitivity. The inhibition curve was developed under different conditions for better performance criteria. The influence of some physicochemical parameters about ELISA performance is summarized in . The highest sensitivity was obtained when the working dilutions of monoclonal antibody were 1:200,000 based on IC50 and maximum OD values. As a water-soluble drug for the amine, the recognition between FFA and antibody could be influenced by ionic strength and pH. Meanwhile, organic solvents could accelerate the dissolution of FF and decrease non-specific recognition. The results suggested that the assay buffer with methanol (1%,v/v, pH 7.2) and 0.35 mol/L NaCl gave a lower IC50 of 0.118 ng/mL for FFA and 0.120 ng/mL for FF. The lowest IC50 value of 0.41 ng/mL was observed when the reaction temperature was controlled at 25°C. The time period of 15 min incubation and the antigen–antibody reaction temperature of 25°C were selected for the analysis. Thus, the optimal assay buffer was chosen as the 0.1 mol/L PBS1 containing 0.35 mol/L NaCl, methanol (1%, v/v), with the optimal pH of 7.2. The blocking period of two hours and 25°C antigen–antibody reaction temperature for 15 min were also selected. The optimum diluted fold of antibody was 1:200,000, with the goat anti-mouse IgG-HRP of 1:1000, while the coating antigen FF-OVA was chosen to be 35 ng/well. The linearity of standard curve for FFA was from 0.02 to 1.62 ng/mL, and the IC50 value was 0.118 ng/mL ( ). As the CR of the antibody to TAP was low (1%), it could not detect TAP residues. The CR of the antibody to FF was 98.3%, and the residue marker of animal origin products is FFA or FF or both of them, so that the ELISA is suitable for the simultaneous detection of FFA and FF. The limit of detection (LOD) was calculated as the average value of 20 blank samples spiked with the mean standard deviations in triplicate.
Table 1. Effect of various factors on the sensitivity of the ELISA (n = 3).
Characterization of antibody
To investigate the specificity of the antibodies, the CR (%) and IC50 of FFA analog were calculated as index factors and compared. FF and FFA were both residue markers, the CR was the main criterion used to select the optimum coating antigen. According to our study, hapten heterology was better than homologous assay for FFA. Therefore, the immunizing hapten is structure-differently designed compared to the plate-coating hapten and could improve ELISA sensitivity and change specificity. FF-OVA was applied as coating antigen and the analogs of FF, CAP, and TAP were used as competitors to develop ELISA inhibition curve. As summarized in , the results implied that the antibodies evoked by derivatives had negligible affinity with original target chemicals. Meanwhile, the IC50 values of CAP and other antibiotics were more than 1000 μg/kg, and CR values were less than 1%. The results of specificity also proved the conclusion of former studies (An et al., Citation2016; Fodey et al., Citation2013) that the methylsulfonylphenyl and fluorine moieties could potentially affect the immunological reactivity of the hapten.
Table 2. IC50 and cross-reactivity (CR) values of the antibody with FFA and other structurally related compounds.
Sample preparation and assay validation
To effectively reduce the matrix effects, pre-treatment procedures of egg samples were modified on the basis of literature (An et al., Citation2016; Guidi et al., Citation2017). Trichloroacetic acid could precipitate protein and increase the recovery of FFA owing to an alkaline drug (Azzouz & Ballesteros, Citation2015). It was applied to generate a sample extraction buffer (PBS2) to eliminate the matrix effect and boost the sensitivity of the proposed method. Samples were diluted with PBS2 by 20-fold before analysis. The LOD value was 0.3 μg/kg for FFA and FF. To evaluate the effectiveness of sample preparation and reproducibility of the ELISA, recovery determination of spiked sample was performed. The recovery rates of FFA and FF at four different concentrations () ranged from 78.3 to 104.1% for FFA and 78.8 to 101.2% for FF. The coefficients of variation were less than 12% for both FFA and FF, which suggested the satisfactory precision of this method.
Table 3. Recoveries of FFA and FF from samples using optimized ELISA methods (n = 5).
Comparison of the ELISA and HPLC method
Six spiked egg samples were detected by the newly established ELISA technique and the conventional HPLC technique (), which was used for evaluating the reliability of the proposed technique. The data from the ELISA were similar to those by the HPLC analysis. However, the concentration values of No.1 and No.2 samples were not detected completely, which were lower than the LOD of the HPLC method. The concentrations of the target chemicals analyzed by the ELISA showed a little higher than those conducted by the HPLC. The result could be explained by the existing matrix interference in the samples and the instrumental features of HPLC equipment.
Table 4. FFAa or FFa concentration in spiked egg samples (n = 3).
Conclusion
One sensitive and rapid ELISA was introduced for the determination of FF and its main metabolite FFA in eggs. The performance criteria of the proposed method were all within acceptable ranges, including the detection of limits, selectivity, recovery rates, and precision. When applied to the same samples, the ELISA technique confirmed to be reliable and accurate. In other words, the proposed method could be employed for the simultaneous detection of FF and FFA in high-throughput eggs.
Disclosure statement
No potential conflict of interest was reported by the author(s).
Additional information
Funding
References
- An, L., Wang, Y., Pan, Y., Tao, Y., Chen, D., Liu, Z., Yang, W., Peng, D., & Yuan, Z. (2016). Development and validation of a sensitive indirect competitive enzyme-linked immunosorbent assay for the screening of florfenicol and thiamphenicol in edible animal tissue and feed. Food Analytical Methods, 9(9), 2434–2443. https://doi.org/10.1007/s12161-016-0436-3
- Azzouz, A., & Ballesteros, E. (2015). Multiresidue method for the determination of pharmacologically active substances in egg and honey using a continuous solid-phase extraction system and gas chromatography-mass spectrometry. Food Chemistry, 178, 63–69. https://doi.org/10.1016/j.foodchem.2015.01.044
- Dasenaki, M. E., & Thomaidis, N. S. (2015). Multi-residue determination of 115 veterinary drugs and pharmaceutical residues in milk powder, butter, fish tissue and eggs using liquid chromatography-tandem mass spectrometry. Analytica Chimica Acta, 880, 103–121. https://doi.org/10.1016/j.aca.2015.04.013
- Filazi, A., Sireli, U. T., Yurdakok, B., Aydin, F. G., & Kucukosmanoglu, A. G. (2014). Depletion of florfenicol and florfenicol amine residues in chicken eggs. British Poultry Science, 55(4), 460–465. https://doi.org/10.1080/00071668.2014.935701
- Fodey, T. L., George, S. E., Traynor, I. M., Delahaut, P., Kennedy, D. G., Elliott, C. T., & Crooks, S. R. (2013). Approaches for the simultaneous detection of thiamphenicol, florfenicol and florfenicol amine using immunochemical techniques. Journal of Immunological Methods, 393(1-2), 30–37. https://doi.org/10.1016/j.jim.2013.04.003
- Guidi, L. R., Tette, P. A., Fernandes, C., Silva, L. H., & Gloria, M. B. (2017). Advances on the chromatographic determination of amphenicols in food. Talanta, 162, 324–338. https://doi.org/10.1016/j.talanta.2016.09.068
- Imran, M., Fazal, E. H., Tawab, A., Rauf, W., Rahman, M., Khan, Q. M., Asi, M. R., & Iqbal, M. (2017). LC-MS/MS based method development for the analysis of florfenicol and its application to estimate relative distribution in various tissues of broiler chicken. Journal of Chromatography B, 1063, 163–173. https://doi.org/10.1016/j.jchromb.2017.08.029
- Lei, X., Xu, L., Song, S., Liu, L., & Kuang, H. (2017). Development of an ultrasensitive ic-ELISA and immunochromatographic strip assay for the simultaneous detection of florfenicol and thiamphenicol in eggs. Food and Agricultural Immunology, 29(1), 254–266. https://doi.org/10.1080/09540105.2017.1371114
- Li, R., Lin, Z.-J., Yang, J.-Y., Xu, Z.-L., Wang, H., Lei, H.-T., Sun, Y.-M., & Shen, Y.-D. (2018). An indirect competitive enzyme-linked immunosorbent assay for simultaneous determination of florfenicol and thiamphenicol in animal meat and urine. Chinese Journal of Analytical Chemistry, 46(8), 1321–1328. https://doi.org/10.1016/s1872-2040(18)61104-1
- Luo, P., Cao, X., Wang, Z., Jiang, H., Zhang, S., Chen, X., Wang, J., Feng, C., & Shen, J. (2009). Development of an enzyme-linked immunosorbent assay for the detection of florfenicol in fish feed. Food and Agricultural Immunology, 20(1), 57–65. https://doi.org/10.1080/09540100802712741
- Luo, P. J., Jiang, W. X., Chen, X., Shen, J. Z., & Wu, Y. N. (2011). Technical note: Development of an enzyme-linked immunosorbent assay for the determination of florfenicol and thiamphenicol in swine feed. Journal of Animal Science, 89(11), 3612–3616. https://doi.org/10.2527/jas.2010-3403
- Luo, P., Jiang, H., Wang, Z., Feng, C., He, F., & Shen, J. (2009). Simultaneous determination of florfenicol and its metabolite florfenicol amine in swine muscle tissue by a heterologous enzyme-linked immunosorbent assay. Journal of AOAC International, 92(3), 981–988. https://doi.org/10.1093/jaoac/92.3.981
- Qiao, M., Ying, G.-G., Singer, A. C., & Zhu, Y.-G. (2018). Review of antibiotic resistance in China and its environment. Environment International, 110, 160–172. https://doi.org/10.1016/j.envint.2017.10.016
- Saito-Shida, S., Shiono, K., Narushima, J., Nemoto, S., & Akiyama, H. (2019). Determination of total florfenicol residues as florfenicol amine in bovine tissues and eel by liquid chromatography-tandem mass spectrometry using external calibration. Journal of Chromatography B, 1109, 37–44. https://doi.org/10.1016/j.jchromb.2019.01.018
- Samsonova, J. V., Cannavan, A., & Elliott, C. T. (2012). A Critical Review of Screening methods for the detection of chloramphenicol, thiamphenicol, and florfenicol residues in foodstuffs. Critical Reviews in Analytical Chemistry, 42(1), 50–78. https://doi.org/10.1080/10408347.2012.629951
- Santos, L., & Ramos, F. (2018). Antimicrobial resistance in aquaculture: Current knowledge and alternatives to tackle the problem. International Journal of Antimicrobial Agents, 52(2), 135–143. https://doi.org/10.1016/j.ijantimicag.2018.03.010
- Schwarz, S., Kehrenberg, C., Doublet, B., & Cloeckaert, A. (2004). Molecular basis of bacterial resistance to chloramphenicol and florfenicol. FEMS Microbiology Reviews, 28(5), 519–542. https://doi.org/10.1016/j.femsre.2004.04.001
- Shen, J., Xia, X., Jiang, H., Li, C., Li, J., Li, X., & Ding, S. (2009). Determination of chloramphenicol, thiamphenicol, florfenicol, and florfenicol amine in poultry and porcine muscle and liver by gas chromatography-negative chemical ionization mass spectrometry. Journal of Chromatography B, 877(14–15), 1523–1529. https://doi.org/10.1016/j.jchromb.2009.03.040
- Song, J., Wang, R.-M., Wang, Y.-Q., Tang, Y.-R., & Deng, A.-P. (2010). Hapten design, modification and preparation of artificial antigens. Chinese Journal of Analytical Chemistry, 38(8), 1211–1218. https://doi.org/10.1016/s1872-2040(09)60063-3
- Wang, Y., Li, X., Zhang, Z., Ding, S., Jiang, H., Li, J., Shen, J., & Xia, X. (2016). Simultaneous determination of nitroimidazoles, benzimidazoles, and chloramphenicol components in bovine milk by ultra-high performance liquid chromatography-tandem mass spectrometry. Food Chemistry, 192, 280–287. https://doi.org/10.1016/j.foodchem.2015.07.033
- Wang, J., Wang, Q., Zheng, Y., Peng, T., Yao, K., Xie, S., Zhang, X., Xia, X., Li, J., & Jiang, H. (2017). Development of a quantitative fluorescence-based lateral flow immunoassay for determination of chloramphenicol, thiamphenicol and florfenicol in milk. Food and Agricultural Immunology, 29(1), 56–66. https://doi.org/10.1080/09540105.2017.1359498
- Wang, B., Zhao, X., Xie, X., Xie, K., Zhang, G., Zhang, T., & Liu, X. (2019). Development of an accelerated solvent extraction approach for quantitative analysis of chloramphenicol, thiamphenicol, florfenicol, and florfenicol amine in poultry eggs. Food Analytical Methods, 12(8), 1705–1714. https://doi.org/10.1007/s12161-019-01517-4
- Wu, J., Chang, C., Ding, W., & He, D. (2008). Determination of florfenicol amine residues in animal edible tissues by an indirect competitive ELISA.pdf. Journal of Agricultural and Food Chemistry, 56(18), 8261–8267. https://doi.org/10.1021/jf8010454
- Xie, K., Jia, L., Yao, Y., Xu, D., Chen, S., Xie, X., Pei, Y., Bao, W., Dai, G., Wang, J., & Liu, Z. (2011). Simultaneous determination of thiamphenicol, florfenicol and florfenicol amine in eggs by reversed-phase high-performance liquid chromatography with fluorescence detection. Journal of Chromatography B, 879(23), 2351–2354. https://doi.org/10.1016/j.jchromb.2011.06.027
- Xie, X., Wang, B., Pang, M., Zhao, X., Xie, K., Zhang, Y., Wang, Y., Guo, Y., Liu, C., Bu, X., Wang, R., Shi, H., Zhang, G., Zhang, T., Dai, G., & Wang, J. (2018). Quantitative analysis of chloramphenicol, thiamphenicol, florfenicol and florfenicol amine in eggs via liquid chromatography-electrospray ionization tandem mass spectrometry. Food Chemistry, 269, 542–548. https://doi.org/10.1016/j.foodchem.2018.07.045