ABSTRACT
This study was to evaluate the protective effect of fucoidan on the intestinal mucosal barrier in non-obese diabetic mice (NOD). Twenty NOD mice were randomly assigned to two groups: the control group and the fucoidan intervention group which were fed with fucoidan at a concentration of 600 mg/kg BW (body weight) per day for five weeks. Mice treated with fucoidan had a significant improvement in glycemic control, and the proportions of Th1/Th2/Th17 cells and the levels of endotoxin, DAO and D-LA were reduced. Fucoidan treatment improved intestinal barrier function by increasing ZO-1, claudin-1 and Occludin protein expression. Moreover, the expression levels of phosphorylated ERK1/2, NF-кB p65 and p38 MAPK were decreased. Our result suggested that pre-treatment with fucoidan could attenuate the inflammatory response and maintain intestinal barrier function in NOD mice. Fucoidan-based functional food may be potential for the prevention of T1DM (type 1 diabetes mellitus).
Introduction
Type 1 diabetes mellitus (T1DM) is one of the most common metabolic disorders in young adults and children (Gülden et al., Citation2015). In the past few years, the incidence of T1DM has rapidly risen worldwide. As the escalating incidence, many drugs are clinically administered for diabetic therapy. However, they are costly and have many side effects and toxicity (Martel et al., Citation2017). Therefore, the key to the prevention and therapy for diabetes mellitus is to screen novel and effective functional food or medicines (Cheng et al., Citation2019).
T1DM is an autoimmune disease characterised by autoimmune-mediated destruction of pancreatic β cells, which led to insufficient insulin secretion. The intestinal mucosa is the first line of defense of the intestinal barrier (Wang et al., Citation2018). The intestinal epithelium provides a barrier preventing pathogenic bacteria from escaping from the intestinal and activating autoimmune system (Lee et al., Citation2010). Epithelial tight junctions (TJ) is a gate between the external environment and internal tissues, and TJ plays a vital role in maintaining the integrity of the intestine(Zhang et al., Citation2019). It may cause autoimmune disease (such as type 1 diabetes) (Secondulfo et al., Citation2004) in a susceptible individual when the integrity of the intestinal epithelial barrier function is impaired (Lee, Citation2000; Vaarala, Citation2011). Many studies suggested that increased gut permeability has been observed in humans with high-risk for diabetes and animal models of T1DM (Bosi et al., Citation2006; Sapone et al., Citation2006; Vaarala, Citation2012; Vaarala et al., Citation2008; Visser et al., Citation2009). Increased intestinal permeability preceded the onset of T1DM was also found in animal models of diabetes (Meddings et al., Citation1999; Neu et al., Citation2005). Also, a study showed increased gut permeability before the clinical onset of T1DM (Bosi et al., Citation2006). At the same time, in the NOD (non-obese diabetic) mice model of T1DM, gut permeability was increased before the development of diabetes (Lee et al., Citation2010).
Brown algae are a typical traditional diet in China, Japan and Korea, and may contribute to the low prevalence of diabetes in these countries (Cardoso et al., Citation2015; Kumar & Brown, Citation2013). Fucoidan is a complex L-fucose enriched sulfated polysaccharides (Ersoy & Kizilay, Citation2018) found in marine brown algae and some marine invertebrates (Klettner, Citation2016; Park et al., Citation2017). Fucoidan exhibits many different biological activities, such as anti-aggregation (Cumashi et al., Citation2007; Richard et al., Citation2006), anti-inflammatory effects (Kim & Lee, Citation2012; Park et al., Citation2011), anti-oxidation (Nagamine et al., Citation2014; Rocha de Souza et al., Citation2007), immunomodulatory (Raghavendran et al., Citation2011) and a regulatory effect on blood glucose homeostasis (Kim et al., Citation2012), which all seem to be beneficial for reducing the complications of diabetes. Thus, considerable interest has been generated in investigating the potential therapeutic effects of fucoidan on diabetes.
It was reported that under the influence of fucoidan, there was an increased level of insulin in serum, which led to hypoglycemic (Mukhamejanov et al., Citation2019). The use of fucoidan in a dose of 200 mg/kg prevented hyperglycemia in diabetic mice (Kim et al., Citation2012). Some studies found that the low molecular weight fucoidan took advantage over other medicines for diabetes (Liang et al., Citation2016), because it could prevent diabetic nephropathy (Chen et al., Citation2013; Wang et al., Citation2014), exert antithrombotic effect without affecting coagulation (Zhu et al., Citation2010), and improve diabetes-induced hypertension (Cui et al., Citation2014). Iraha et al. demonstrated that fucoidan could prevent the epithelial barrier from oxidative injury as well as barrier disruption by upregulating the expression of claudin-1 (Iraha et al., Citation2013). Furthermore, our previous study showed that fucoidan could prevent the development of autoimmune diabetes in mice (Xue et al., Citation2019).
In this study, we examined the protective effect of fucoidan on the intestinal mucosal barrier in NOD mice, which might provide a novel approach for the treatment of T1DM.
Materials and methods
Animal model preparation
Twenty female NOD mice (6 weeks old, 12–15 g) were obtained from Shanghai Laboratory Animal Center, Chinese Academy of Sciences. The mice were housed in plastic cages, an automated 12 h/12 h dark–light cycle, a constant temperature of 22°C ± 2°C, and relative humidity of 50%-60% throughout the entire experiment. The mice were free to access the standard dry diet as well as tap water ad libitum. All aspects of the experiment were conducted according to the Guide for the Care, and Use of Laboratory Animals (issued by the Ministry of Science and Technology of the People’s Republic of China in 2006 [398]) and this research was approved by the Review Committee for the Use of Human or Animal Subjects of the Medical College of Qingdao University (Shi et al., Citation2017; Xue et al., Citation2017).
After an adaptation period of one week, the mice were randomly assigned to the following two groups with ten mice in each group: the control group and the fucoidan intervention group. During the experiment, mice in the fucoidan intervention group were given an oral administration (gavage) of fucoidan (Sigma-Aldrich St. Louis, MO, USA) at a dose of 600 mg/kg BW once a day. Meanwhile, mice in the control group were given oral administration with normal saline. After five weeks, following overnight fasting, intraperitoneal glucose tolerance test (IPGTT) was performed in each group, the mice were sacrificed with diethyl ether, and blood was extracted from the mice eyeballs. The spleen, jejunal and colonic tissues were obtained for subsequent analysis.
Intraperitoneal glucose tolerance test
At the end of the experimental period, glucose solution (1 g glucose/kg BW) was administered i.p. to NOD mice after overnight fasting. After the injection of glucose, blood samples were taken at 0, 30, 60, 120 and 180 min time points. Then, the glucose level was determined with a glucometer (Accu-Chek, Switzerland).
The level of endotoxin, D-LA, DAO, and FABP2
The level of D-lactic acid (D-LA), diamine oxidase (DAO) and fatty acid-binding protein 2 (FABP2) in plasma were detected using an enzyme-linked immunosorbent assay (ELISA) kit (Cusabio, Wuhan, China). Besides, the level of endotoxin was determined using a chromogenic end-point tachypleus amebocyte lysate (CE TAL) assay kit (Ybscience, Shanghai, China). The experiment was conducted according to the manufacturers’ instructions.
Flow cytometric analysis of Th1/Th2/Th17
The subpopulation frequencies of T-helper 1 (Th1), T-helper 2 (Th2) and T-helper 17 (Th17) cells were evaluated by flow cytometry (Invitrogen, Carlsbad, California, USA). Spleen single-cell suspensions were prepared and stained with the following antibodies: anti-CD3-FITC, anti-CD4-FITC, respectively. Subsequently, the cells were treated with Fixation/Permeabilization Solution Kit for 20 min, then added anti-IFN-γ-PE, anti-IL-4-APC and anti-IL-17A-PE, respectively. Finally, the cells were incubated at 4°C for 30 min in the dark. Differentiation of Th1, Th2 and Th17 cells was confirmed by intracellular cytokine staining of IFN-γ, IL-4 and IL-17A. All antibodies were purchased from Merck-Millipore (Darmstadt, Germany).
Pathological observation of intestinal tissue
After mice were sacrificed, jejunal and colonic tissues were fixed in 10% neutral buffered formalin. The intestinal tissues were embedded with paraffin and dyed with HE (hematoxylin and eosin, Vector Laboratories, Burlingame, California). Subsequently, histological changes were observed with an optical microscope (Nikon Eclipse Ti light microscope, Tokyo, Japan).
Ultrastructural observation of intestinal tissue
The tissues were cut into 1 mm × 2 mm sections, fixed with 3% glutaraldehyde for 4 h, and washed three times in 0.1 mol L−1 phosphate buffer. Subsequently, the tissues were post-fixed with 1% OsO4 for 1 h and washed three times in the phosphate buffer. The tissues were dehydrated in a graded series of ethanol and embedded in epoxy resin. After stained with uranyl acetate and lead citrate, the sections were observed under TEM (Transmission Electron Microscopy) of Model Hitachi-800.
Western blot analysis
The jejunal and colonic tissues were homogenised with T-PER (tissue protein extraction reagent) (Biovision, San Francisco, USA). The proteins were extracted, and the BCA (bicinchoninic acid) protein assay kit (Biorbyt, Cambridge, UK) was used for quantifying the protein concentration. The proteins were separated using SDS-PAGE (sodium dodecyl sulfate-polyacrylamide gel electrophoresis) and then transferred to a PVDF (polyvinylidene difluoride) membrane (Seebio, Shanghai, China). The membrane was blocked with TBST(Tris-buffered saline, 0.1% Tween-20; pH 7.4, containing 5% nonfat dry milk) for one hour, and then incubated with specific primary antibodies (anti-ZO-1, Occludin, claudin-1, phospho-p38 MAPK, phospho-ERK1/2, phospho-JNK, NF-κB p65 and anti-actin) overnight at 4°C. After washed with tris-buffered saline three times, the membrane was incubated with corresponding secondary antibodies. The analysis of protein blots was done with the Image J software. All antibodies were purchased from Merck-Millipore (Darmstadt, Germany).
Immunofluorescence analysis
The sections were deparaffinized and hydrated through a graded series of ethanol. Subsequently, the tissues were repaired in a restoration solution and slowly cooled to room temperature. After washed three times with PBS (phosphate buffer saline), sections were added 3% H2O2 and incubated for 20 min. Then, sections were blocked with BSA for 30 min and incubated with the primary antibodies (ZO-1, 1:60) overnight at 4°C. After PBS wash, they were incubated with fluorescent secondary antibodies (1:60) at 37°C for 30 min in the dark. Sections were dyed with DAPI (4’,6-diamidino-2-phenylindole, Ybscience, Shanghai, China) and sealed with water-soluble tablet sealing liquid. At last, a fluorescence microscope (OlympusL, Tokyo, Japan) was used to observe and photograph.
Statistical analysis
Statistical analysis was performed using Prism 7.0 and SPSS v 23.0. Data were expressed as mean ± SD. Differences between the two groups were analyzed by the T-test. Statistical significance was set at P < 0.05.
Result
Effect of fucoidan-treatment on fasting glucose levels and glycemic control
After five weeks of intervention, an intraperitoneal glucose tolerance test was performed on fasted mice (12-week-old). NOD mice were injected i.p. with 1 g glucose/kg BW glucose solution and sampled for blood glucose levels. As showed in , the mice that received fucoidan had significantly better fasting glucose levels and glycemic control. Trends in blood glucose of NOD mice in the two groups exhibited a similar pattern with blood glucose levels peaking at 30 min after glucose load. The mice in the control group had significantly higher blood glucose levels than that in the fucoidan group (P < 0.05). Fucoidan treatment resulted in lower blood glucose level at 60 and 120 min after glucose load than those in the control group, and the speed of glucose decline was increased compared to the control group.
Figure 1. Effect of fucoidan on insulin and blood glucose. For both groups, IPGTT was performed before sacrificed. The results showed that glucose tolerance was significantly increased after fucoidan treatment. Compared to the control group, fucoidan treatment significantly lowered blood glucose levels at 60 and 120 min after glucose load (P < 0.05). (A) Insulin level. (B) blood glucose level. *P < 0.05 vs the control group.
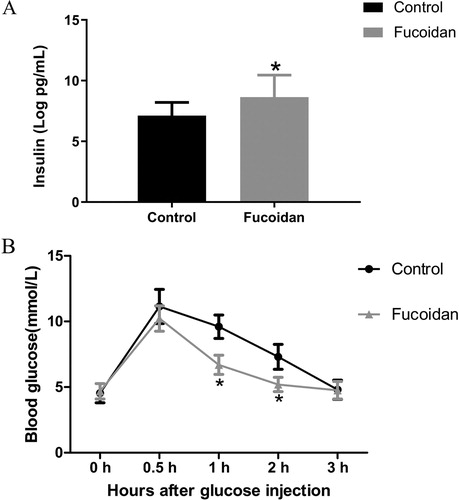
The pathological changes of the jejunal and colonic tissues
We analyzed the pathological changes of the jejunal and colonic tissuesin mice (). In the control group, the villi were loose, and the intestinal wall was damaged. NOD mice showed an irregular arrangement of cell and inflammatory infiltration of the tissues. After treated with fucoidan, the length of the intestinal villi was increased, and the structure was evident. The histopathological features were significantly improved, and less inflammatory infiltration was observed. This result suggested that fucoidan could restore the damaged mucosa to some degree.
Figure 2. Pathological changes of the jejunal and colonic tissues. (A) Histopathological changes of intestinal. To study the effect of fucoidan on the intestinal tissue of NOD mice, the colonic and jejunal tissues were obtained and dyed with HE. Tissue from the control group exhibited histological damage with increased infiltration of inflammatory cells. However, fucoidan attenuated the pathological changes in the jejunal and colonic tissues of NOD mice. (B) Ultrastructure observations of intestinal tissues. The tight junction became fuzzy and slight relaxation with low electron density in the control group. In the fucoidan group, histological morphology and the tight junctions were improved. 1, tight junction; 2, adherens junction; 3, Desmosome. *P<0.05 vs the control group.
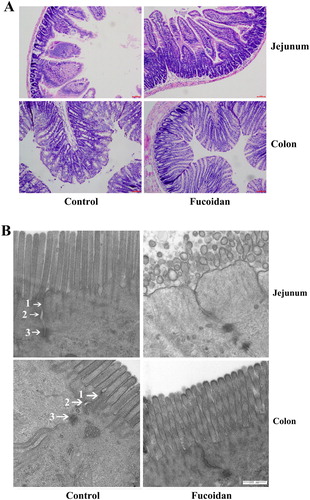
The intercellular junction mainly includes tight junctions, adherens junctions, desmosomes. As shown in (B), under the transmission electron microscopy the tight junction became fuzzy and slight relaxation in the control group. Furthermore, the electron density of the tight junction was reduced in the control group. In the fucoidan-treated mice, the tight junctions were improved and the narrow of the intercellular spaces were observed.
Effect of fucoidan on intestinal permeability in NOD mice
DAO, D-LA, FABP2 and endotoxin are markers of intestinal permeability. ELISA data showed that fucoidan treatment reduced the levels of DAO, D-LA and endotoxin as compared to control mice (P < 0.05). Intestinal permeability fluorescence results indicated that the continuity of fluorescent substance was restored, and intestinal permeability was much improved after fucoidan treatment ().
Figure 3. Effect of fucoidan on intestinal permeability. The levels of DAO, D-LA, FABP2 and endotoxin were detected by ELISA. The data showed that after fucoidan treatment, the levels of DAO, D-LA and endotoxin were reduced compared with those in the control group (P < 0.05). Intestinal permeability fluorescence results showed that loss of continuity of the fluorescent substance and lacked closure was observed in the control group. However, in the fucoidan group, the fluorescent substance was restored continuity, and intestinal permeability was much improved. *P<0.05 vs the control group.
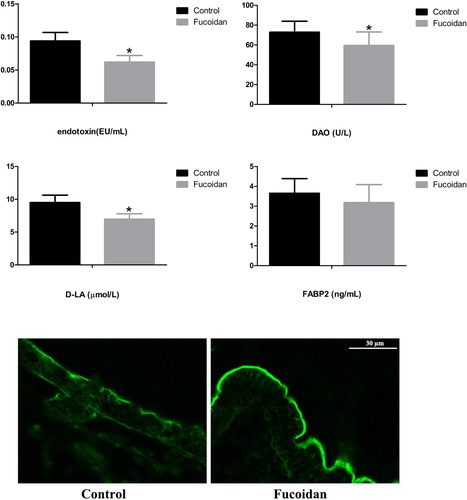
Effect of fucoidan on the equilibrium of the Th1/Th2/Th17
The leading indices to evaluate the function of the intestinal mucosal immunity were T-helper cells. Many diseases involved dyshomeostasis in the activities of T Th1/Th2/Th17 cells, which were related to immune regulation. Therefore, we detected the levels of the representative cytokines secreted by the Th1, Th2 and Th17 cells, IFN-γ, IL-4 and IL-17A, respectively (). The results demonstrated that fucoidan-treatment notably reduced the levels of IFN-γ, IL-4 and IL-17A and down-regulated the IFN-γ/IL-4 ratio in mice, compared with those in the control group (P < 0.05). Those cytokines level represents the change in T-helper cells, and IFN-γ/IL-4 ratio represents the Th1/Th2 immune balance. In a word, our data suggested that fucoidan mediated the equilibrium of the Th1/Th2/Th17 ratio to maintain the immune balance.
Effect of fucoidan on the expression of Occludin, claudin-1 and ZO-1 in the jejunal and colonic tissues
To further evaluated the intestinal mucosal barrier function, the expressions of claudin-1, Occludin and ZO-1 in the jejunal and colonic tissues of NOD mice were determined by western blots. β-actin was performed as an internal standard (). Compared to the control group, fucoidan significantly increased the expressions of Occludin, claudin-1 and ZO-1 in the jejunal and colonic tissues (P < 0.05).
Figure 5. Effect of fucoidan on the expressions of Occludin, claudin-1 and ZO-1. Western blot analysis was used to detect the expressions of Occludin, claudin-1 and ZO-1; β-actin was used as an internal standard. After fucoidan treatment, the levels of Occludin, claudin-1 and ZO-1 were significantly higher than those in the control group. As shown in the fluorescence microscope, the intestinal tissues in the control group displayed significantly weaker ZO-1 staining compared to the fucoidan group. *P < 0.05 vs. the control group; **P < 0.01 vs. the control group.
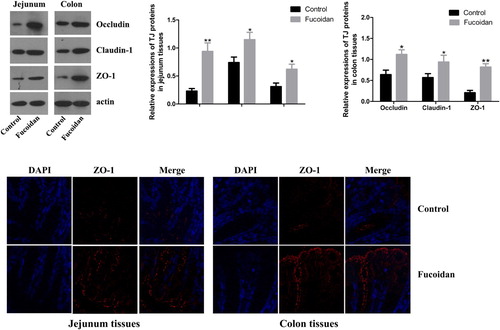
As shown in the immunofluorescence assay of ZO-1, compared to the control group, the mice in the fucoidan group displayed significantly stronger ZO-1 staining. The result confirmed that fucoidan treatment upregulated the ZO-1 expression.
Effect of fucoidan on p-p38 MAPK, p-ERK1/2, p-JNK and NF-κB p65
The involvement of various intracellular signalling pathways, such as MAPKs and NF-κB, in inflammatory mediators induction, has been reported (Park et al., Citation2011; Zhang & Dong, Citation2007). Thus, we analyzed the expressions of MAPKs and NF-κB by western blots. As shown in , the levels of p-p38 MAPK, p-ERK1/2 and NF-κB p65 in the fucoidan group were lower than those in the control group (P < 0.05). However, there was no statistical significance in the level of p-JNK.
Figure 6. Effect of fucoidan on p-p38 MAPK, p-ERK1/2, p-JNK and NF-κB p65. We analyzed the expressions of p-p38 MAPK, p-ERK1/2, p-JNK and NF-κB p65 by western blots. The levels of p-p38 MAPK, p-ERK1/2 and NF-κB p65 in the fucoidan group were lower than those in the control group. However, there was no statistical significance in p-JNK level. *P < 0.05 vs. the control group; **P < 0.01 vs. the control group.
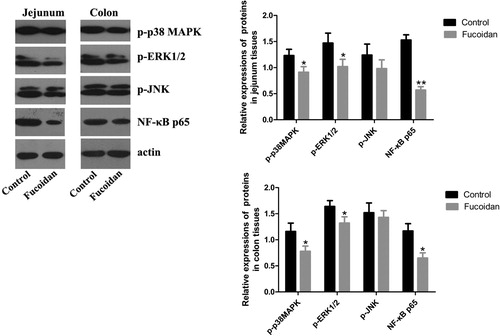
Discussion
Fucoidan is considered to be a potential active substance for disease prevention and therapy, and the essential bioactive ingredient was the sulfated polysaccharide (Rajapakse & Kim, Citation2011). In this study, we evaluated the effect of dietary fucoidan on the intestinal mucosal barrier and inflammation in NOD mice to reveal the mechanism of fucoidan in the prevention of T1DM. Recent studies have suggested that fucoidan reduced the rate absorption of glucose from the gut to maintain glucose homeostasis (Mukhamejanov et al., Citation2019). Moreover, fucoidan decreased glycemia in STZ rats (Liang et al., Citation2016). Our result was in line with the recent study, we found that mice received fucoidan had significantly better fasting glucose levels and glycemic control.
Anti-inflammatory effects were one of the biological activities of fucoidan (Kiec-Wilk et al., Citation2016; Penny et al., Citation2015; Zhong et al., Citation2017). T1DM was an autoimmune disease characterised by autoimmune-mediated destruction of pancreatic β cells. T-helper 1 (Th1) cells through their cytokines (such as IFN-γ) orchestrated the destruction of beta-cells in T1DM. In contrast, T-helper 2 (Th2) cells through their cytokines (such as IL-4) counteracted T-helper 1 cell function, protected pancreatic β cells (Bachmann & Oxenius, Citation2007; Opal & DePalo, Citation2000; Stechova et al., Citation2007). Autoimmunity was related to the activities of Th1/Th2 immune balance (Chatzigeorgiou et al., Citation2010). In addition to Th1 and Th2 cells, T helper cells also differentiate to Th17 cells, which were involved in tissue inflammation and autoimmunity (Bettelli et al., Citation2008). Several studies in NOD mice suggested that Th17 might have a role in T1DM (Abdel-Moneim et al., Citation2018; Jain et al., Citation2008; Li et al., Citation2015). In our research, fucoidan-treatment down-regulated the IFN-γ/IL-4 ratio in mice and notably reduced the expression of IFN-γ, IL-4 and IL-17A.
Inflammation involved many different signal pathways, such as NF-κB and MAPKS (Park et al., Citation2011; Zhang & Dong, Citation2007). It was reported that fucoidan inhibited the activation of JNK, ERK and p38 MAPKs in diabetes (Li et al., Citation2008). Besides, NF-κB was a critical transcription factor involved in the inflammatory response. Once activated by inflammatory stimulants (such as lipopolysaccharide), NF-κB was released into the nucleus and then led to transcriptional induction of inflammation-associated genes. Our data showed that after fucoidan-treatment, the levels of p-p38 MAPK, p-ERK1/2, NF-κB p65 in NOD mice were decreased. Thus, we considered that fucoidan improved the development of autoimmune diseases by regulated the inflammation through NF-κB, ERK1/2 and p38 MAPKs.
In addition, intestinal permeability also seems to be an essential regulator of autoimmune diabetes. The intercellular junction mainly includes adherens junctions (AJs), tight junctions (TJs), gap junctions, desmosomes, etc. (Anderson & Van Itallie, Citation1995). TJ was a multi-protein complex composed of the claudin family, Occludin, junctional adhesion molecule, zonulin, and scaffold proteins ZO-1 (Vaarala, Citation2011). Among them, the claudin, Occludin and ZO-1 were associated with the intestinal barrier (Suzuki, Citation2013; Zhang et al., Citation2020). The early onset of autoimmune diabetes in BB-rats was associated with high gut permeability (Visser et al., Citation2010). Moreover, the expression of claudin-1 and Occludin was decreased in bio-breeding diabetes-prone rats (Neu et al., Citation2005). Increased permeability might allow the entry of bacterial antigens or viral that could lead to the destruction of β cells, and trigger the induction of autoimmunity (Vaarala, Citation2012). These findings were compatible with the opinion that the intestinal barrier provided a new way for the prevention of T1DM. The intact intestinal barrier could prevent DAO, D-LA and endotoxin from infiltrating the internal circulation, which was markers of intestinal permeability. After fucoidan-treatment, the levels of DAO, D-LA and endotoxin were reduced, and the expression of Occludin, claudin-1 and ZO-1 was increased. The results were concordant with our hypothesis that fucoidan could repair the intestinal barrier by enhancing the expression of Occludin, ZO-1 and claudin-1.
Conclusions
In conclusion, the present study may provide evidence for the fucoidan as a regulator of intestinal in the treatment of autoimmune diabetes. However, the intestinal barrier was a complex environment, and the regulation of barrier permeability cannot be elucidated using animal models alone. More detailed research studies are needed to explore further the relationship between fucoidan and T1DM, and the mechanism of the protectioneffect of fucoidan on diabetes.
Acknowledgments
The authors gratefully acknowledge the funds supports.
Disclosure statement
No potential conflict of interest was reported by the author(s).
Additional information
Funding
References
- Abdel-Moneim, A., Bakery, H. H., & Allam, G. (2018). The potential pathogenic role of IL-17/Th17 cells in both type 1 and type 2 diabetes mellitus. Biomedicine & Pharmacotherapy, 101, 287–292. https://doi.org/10.1016/j.biopha.2018.02.103
- Anderson, J. M., & Van Itallie, C. M. (1995). Tight junctions and the molecular basis for regulation of paracellular permeability. American Journal of Physiology, 269, G467–G475. https://doi.org/10.1152/ajpgi.1995.269.4.g467
- Bachmann, M. F., & Oxenius, A. (2007). Interleukin 2: From immunostimulation to immunoregulation and back again. EMBO Reports, 8(12), 1142–1148. https://doi.org/10.1038/sj.embor.7401099
- Bettelli, E., Korn, T., Oukka, M., & Kuchroo, V. K. (2008). Induction and effector functions of T(H)17 cells. Nature, 453(7198), 1051–1057. https://doi.org/10.1038/nature07036
- Bosi, E., Molteni, L., Radaelli, M. G., Folini, L., Fermo, I., Bazzigaluppi, E., Piemonti, L., Pastore, M. R., & Paroni, R. (2006). Increased intestinal permeability precedes clinical onset of type 1 diabetes. Diabetologia, 49(12), 2824–2827. https://doi.org/10.1007/s00125-006-0465-3
- Cardoso, S. M., Pereira, O. R., Seca, A. M., Pinto, D. C., & Silva, A. M. (2015). Seaweeds as preventive agents for cardiovascular diseases: From nutrients to functional foods. Marine Drugs, 13(11), 6838–6865. https://doi.org/10.3390/md13116838
- Chatzigeorgiou, A., Harokopos, V., Mylona-Karagianni, C., Tsouvalas, E., Aidinis, V., & Kamper, E. F. (2010). The pattern of inflammatory/anti-inflammatory cytokines and chemokines in type 1 diabetic patients over time. Annals of Medicine, 42(6), 426–438. https://doi.org/10.3109/07853890.2010.495951
- Chen, J., Wang, W., Zhang, Q., Li, F., Lei, T., Luo, D., Zhou, H., Yang, B., & Câmara, N. O. S. (2013). Low molecular weight fucoidan against renal ischemia-reperfusion injury via inhibition of the MAPK signaling pathway. PLoS One, 8(2), e56224. https://doi.org/10.1371/journal.pone.0056224
- Cheng, Y., Sibusiso, L., Hou, L., Jiang, H., Chen, P., Zhang, X., Wu, M., & Tong, H. (2019). Sargassum fusiforme fucoidan modifies the gut microbiota during alleviation of streptozotocin-induced hyperglycemia in mice. International Journal of Biological Macromolecules, 131, 1162–1170. https://doi.org/10.1016/j.ijbiomac.2019.04.040
- Cui, W., Zheng, Y., Zhang, Q., Wang, J., Wang, L., Yang, W., Guo, C., Gao, W., Wang, X., & Luo, D. (2014). Low-molecular-weight fucoidan protects endothelial function and ameliorates basal hypertension in diabetic Goto-Kakizaki rats. Laboratory Investigation, 94(4), 382–393. https://doi.org/10.1038/labinvest.2014.12
- Cumashi, A., Ushakova, N. A., Preobrazhenskaya, M. E., D'Incecco, A., Piccoli, A., Totani, L., Tinari, N., Morozevich, G. E., Berman, A. E., Bilan, M. I., Usov, A. I., Ustyuzhanina, N. E., Grachev, A. A., Sanderson, C. J., Kelly, M., Rabinovich, G. A., Iacobelli, S., & Nifantiev, N. E. (2007). A comparative study of the anti-inflammatory, anticoagulant, antiangiogenic, and antiadhesive activities of nine different fucoidans from brown seaweeds. Glycobiology, 17(5), 541–552. https://doi.org/10.1093/glycob/cwm014
- Ersoy, O., & Kizilay, G. (2018). Effects of fucoidan on diabetic rat testicular tissue. Biotechnic & Histochemistry, 93(4), 277–285. https://doi.org/10.1080/10520295.2018.1434679
- Gülden, E., Wong, F. S., & Wen, L. (2015). The gut microbiota and type 1 diabetes. Clinical Immunology, 159(2), 143–153. https://doi.org/10.1016/j.clim.2015.05.013
- Iraha, A., Chinen, H., Hokama, A., Yonashiro, T., Kinjo, T., Kishimoto, K., Nakamoto, M., Hirata, T., Kinjo, N., Higa, F., Tateyama, M., Kinjo, F., & Fujita, J. (2013). Fucoidan enhances intestinal barrier function by upregulating the expression of claudin-1. World Journal of Gastroenterology, 19(33), 5500–5507. https://doi.org/10.3748/wjg.v19.i33.5500
- Jain, R., Tartar, D. M., Gregg, R. K., Divekar, R. D., Bell, J. J., Lee, H. H., Yu, P., Ellis, J. S., Hoeman, C. M., Franklin, C. L., & Zaghouani, H. (2008). Innocuous IFNgamma induced by adjuvant-free antigen restores normoglycemia in NOD mice through inhibition of IL-17 production. Journal of Experimental Medicine, 205(1), 207–218. https://doi.org/10.1084/jem.20071878
- Kiec-Wilk, B., Matejko, B., Razny, U., Stankiewicz, M., Skupien, J., Klupa, T., & Malecki, M. T. (2016). Hypoglycemic episodes are associated with inflammatory status in patients with type 1 diabetes mellitus. Atherosclerosis, 251, 334–338. https://doi.org/10.1016/j.atherosclerosis.2016.05.002
- Kim, K. J., & Lee, B. Y. (2012). Fucoidan from the sporophyll of Undaria pinnatifida suppresses adipocyte differentiation by inhibition of inflammation-related cytokines in 3T3-L1 cells. Nutrition Research, 32(6), 439–447. https://doi.org/10.1016/j.nutres.2012.04.003
- Kim, K. J., Yoon, K. Y., & Lee, B. Y. (2012). Fucoidan regulate blood glucose homeostasis in C57BL/KSJ m+/+db and C57BL/KSJ db/db mice. Fitoterapia, 83(6), 1105–1109. https://doi.org/10.1016/j.fitote.2012.04.027
- Klettner, A. (2016). Fucoidan as a potential therapeutic for major blinding diseases–A hypothesis. Marine Drugs, 14(2), https://doi.org/10.3390/md14020031
- Kumar, S. A., & Brown, L. (2013). Seaweeds as potential therapeutic interventions for the metabolic syndrome. Reviews in Endocrine and Metabolic Disorders, 14(3), 299–308. https://doi.org/10.1007/s11154-013-9254-8
- Lee, V. H. L. (2000). Membrane transporters. European Journal of Pharmaceutical Sciences, 2, S41–S50. https://doi.org/10.1016/S0928-0987(00)00163-9
- Lee, A. S., Gibson, D. L., Zhang, Y., Sham, H. P., Vallance, B. A., & Dutz, J. P. (2010). Gut barrier disruption by an enteric bacterial pathogen accelerates insulitis in NOD mice. Diabetologia, 53(4), 741–748. https://doi.org/10.1007/s00125-009-1626-y
- Li, Y., Liu, Y., & Chu, C. Q. (2015). Th17 cells in type 1 diabetes: Role in the pathogenesis and regulation by gut microbiome. Mediators of Inflammation, 2015, 638470. https://doi.org/10.1155/2015/638470
- Li, B., Lu, F., Wei, X., & Zhao, R. (2008). Fucoidan: Structure and bioactivity. Molecules, 13(8), 1671–1695. https://doi.org/10.3390/molecules13081671
- Liang, Z., Zheng, Y., Wang, J., Zhang, Q., Ren, S., Liu, T., Wang, Z., & Luo, D. (2016). Low molecular weight fucoidan ameliorates streptozotocin-induced hyper-responsiveness of aortic smooth muscles in type 1 diabetes rats. Journal of Ethnopharmacology, 191, 341–349. https://doi.org/10.1016/j.jep.2016.06.054
- Martel, J., Ojcius, D. M., Chang, C. J., Lin, C. S., Lu, C. C., Ko, Y. F., Tseng, S.-F., Lai, H.-C., & Young, J. D. (2017). Anti-obesogenic and antidiabetic effects of plants and mushrooms. Nature Reviews Endocrinology, 13(3), 149–160. https://doi.org/10.1038/nrendo.2016.142
- Meddings, J. B., Jarand, J., Urbanski, S. J., Hardin, J., & Gall, D. G. (1999). Increased gastrointestinal permeability is an early lesion in the spontaneously diabetic BB rat. American Journal of Physiology, 276, 951–957. https://doi.org/10.1152/ajpgi.1999.276.4.g951
- Mukhamejanov, E., Kon, G., Erjanova, S., & Kirgizbaeva, A. (2019). Fucoidan—new principle prevention and treatment of diabetes. Journal of Pharmacy and Pharmacology, 7, 316–322. https://doi.org/10.17265/2328-2150/2019.06.005
- Nagamine, T., Nakazato, K., Tomioka, S., Iha, M., & Nakajima, K. (2014). Intestinal absorption of fucoidan extracted from the brown seaweed, Cladosiphon okamuranus. Marine Drugs, 13(1), 48–64. https://doi.org/10.3390/md13010048
- Neu, J., Reverte, C. M., Mackey, A. D., Liboni, K., Tuhacek-Tenace, L. M., Hatch, M., Li, N., Caicedo, R. A., Schatz, D. A., & Atkinson, M. (2005). Changes in intestinal morphology and permeability in the biobreeding rat before the onset of type 1 diabetes. Journal of Pediatric Gastroenterology and Nutrition, 40(5), 589–595. https://doi.org/10.1097/01.MPG.0000159636.19346.C1
- Opal, S. M., & DePalo, V. A. (2000). Anti-inflammatory cytokines. Chest, 117(4), 1162–1172. https://doi.org/10.1378/chest.117.4.1162
- Park, J., Cha, J. D., Choi, K. M., Lee, K. Y., Han, K. M., & Jang, Y. S. (2017). Fucoidan inhibits LPS-induced inflammation in vitro and during the acute response in vivo. International Immunopharmacology, 43, 91–98. https://doi.org/10.1016/j.intimp.2016.12.006
- Park, H. Y., Han, M. H., Park, C., Jin, C. Y., Kim, G. Y., Choi, I. W., Kim, N. D., Nam, T.-J., Kwon, T. K., & Choi, Y. H. (2011). Anti-inflammatory effects of fucoidan through inhibition of NF-κB, MAPK and Akt activation in lipopolysaccharide-induced BV2 microglia cells. Food and Chemical Toxicology, 49(8), 1745–1752. https://doi.org/10.1016/j.fct.2011.04.020
- Penny, H. A., Leeds, J. S., Kurien, M., Averginos, A., Hopper, A. D., Hadjivassiliou, M., Tesfaye, S., & Sanders, D. S. (2015). The relationship between inflammatory bowel disease and type 1 diabetes mellitus: A study of relative prevalence in comparison with population controls. Journal of Gastrointestinal and Liver Diseases, 24, 125–126.
- Raghavendran, H. R., Srinivasan, P., & Rekha, S. (2011). Immunomodulatory activity of fucoidan against aspirin-induced gastric mucosal damage in rats. International Immunopharmacology, 11(2), 157–163. https://doi.org/10.1016/j.intimp.2010.11.002
- Rajapakse, N., & Kim, S. K. (2011). Nutritional and digestive health benefits of seaweed. Advances in Food and Nutrition Research, 64, 17–28. https://doi.org/10.1016/B978-0-12-387669-0.00002-8
- Richard, B., Bouton, M. C., Loyau, S., Lavigne, D., Letourneur, D., Jandrot-Perrus, M., & Arocas, V. (2006). Modulation of protease nexin-1 activity by polysaccharides. Thrombosis and Haemostasis, 95(2), 229–235. https://doi.org/10.1160/TH05-08-0546
- Rocha de Souza, M. C., Marques, C. T., Guerra Dore, C. M., Ferreira da Silva, F. R., Oliveira Rocha, H. A., & Leite, E. L. (2007). Antioxidant activities of sulfated polysaccharides from brown and red seaweeds. Journal of Applied Phycology, 19(2), 153–160. https://doi.org/10.1007/s10811-006-9121-z
- Sapone, A., de Magistris, L., Pietzak, M., Clemente, M. G., Tripathi, A., Cucca, F., Lampis, R., Kryszak, D., Carteni, M., Generoso, M., Iafusco, D., Prisco, F., Laghi, F., Riegler, G., Carratu, R., Counts, D., & Fasano, A. (2006). Zonulin upregulation is associated with increased gut permeability in subjects with type 1 diabetes and their relatives. Diabetes, 55(5), 1443–1449. https://doi.org/10.2337/db05-1593
- Secondulfo, M., Iafusco, D., Carratù, R., deMagistris, L., Sapone, A., Generoso, M., Mezzogiorno, A., Sasso, F. C., Cartenı`, M., De Rosa, R., Prisco, F., & Esposito, V. (2004). Ultrastructural mucosal alterations and increased intestinal permeability in non-celiac, type I diabetic patients. Digestive and Liver Disease, 36(1), 35–45. https://doi.org/10.1016/j.dld.2003.09.016
- Shi, H., Chang, Y., Gao, Y., Wang, X., Chen, X., Wang, Y., Xue, C., & Tang, Q. (2017). Dietary fucoidan of Acaudina molpadioides alters gut microbiota and mitigates intestinal mucosal injury induced by cyclophosphamide. Food & Function, 8(9), 3383–3393. https://doi.org/10.1039/C7FO00932A
- Stechova, K., Bohmova, K., Vrabelova, Z., Sepa, A., Stadlerova, G., Zacharovova, K., & Faresjö, M. (2007). High T-helper-1 cytokines but low T-helper-3 cytokines, inflammatory cytokines and chemokines in children with high risk of developing type 1 diabetes. Diabetes/Metabolism Research and Reviews, 23(6), 462–471. https://doi.org/10.1002/dmrr.718
- Suzuki, T. (2013). Regulation of intestinal epithelial permeability by tight junctions. Cellular and Molecular Life Sciences, 70(4), 631–659. https://doi.org/10.1007/s00018-012-1070-x
- Vaarala, O. (2011). The gut as a regulator of early inflammation in type 1 diabetes. Current Opinion in Endocrinology, Diabetes and Obesity, 18(4), 241–247. https://doi.org/10.1097/MED.0b013e3283488218
- Vaarala, O. (2012). Is the origin of type 1 diabetes in the gut? Immunology and Cell Biology, 90(3), 271–276. https://doi.org/10.1038/icb.2011.115
- Vaarala, O., Atkinson, M. A., & Neu, J. (2008). The “perfect storm” for type 1 diabetes: The complex interplay between intestinal microbiota, gut permeability, and mucosal immunity. Diabetes, 57(10), 2555–2562. https://doi.org/10.2337/db08-0331
- Visser, J. T., Lammers, K., Hoogendijk, A., Boer, M. W., Brugman, S., Beijer-Liefers, S., Zandvoort, A., Harmsen, H., Welling, G., Stellaard, F., Bos, N. A., Fasano, A., & Rozing, J. (2010). Restoration of impaired intestinal barrier function by the hydrolysed casein diet contributes to the prevention of type 1 diabetes in the diabetes-prone BioBreeding rat. Diabetologia, 53(12), 2621–2628. https://doi.org/10.1007/s00125-010-1903-9
- Visser, J., Rozing, J., Sapone, A., Lammers, K., & Fasano, A. (2009). Tight junctions, intestinal permeability, and autoimmunity: Celiac disease and type 1 diabetes paradigms. Annals of the New York Academy of Sciences, 1165(1), 195–205. https://doi.org/10.1111/j.1749-6632.2009.04037.x
- Wang, J., Liu, H., Li, N., Zhang, Q., & Zhang, H. (2014). The protective effect of fucoidan in rats with streptozotocin-induced diabetic nephropathy. Marine Drugs, 12(6), 3292–3306. https://doi.org/10.3390/md12063292
- Wang, X., Yu, H., Shan, A., Jin, Y., Fang, H., Zhao, Y., Shen, J., Zhou, C., Zhou, Y., Fu, Y., Wang, J., & Zhang, J. (2018). Toxic effects of zearalenone on intestinal microflora and intestinal mucosal immunity in mice. Food and Agricultural Immunology, 29(1), 1002–1011. https://doi.org/10.1080/09540105.2018.1503233
- Xue, M., Liang, H., Ji, X., Liu, Y., Ge, Y., Hou, L., & Sun, T. (2019). Fucoidan prevent murine autoimmune diabetes via suppression TLR4-signaling pathways, regulation DC/Treg induced immune tolerance and improving gut microecology. Nutrition & Metabolism, 16(1), 87. https://doi.org/10.1186/s12986-019-0392-1
- Xue, M., Liang, H., Tang, Q., Xue, C., He, X., Zhang, L., & Li, Z. (2017). The protective and immunomodulatory effects of fucoidan against 7,12-dimethyl benz[a]anthracene-induced experimental mammary carcinogenesis through the PD1/PDL1 signaling pathway in rats. Nutrition and Cancer, 69(8), 1234–1244. https://doi.org/10.1080/01635581.2017.1362446
- Zhang, Y., Chen, S., Zong, X., Wang, C., Shi, C. Y., Wang, F. Q., Wang, Y., & Lu, Z. (2020). Peptides derived from fermented soybean meal suppresses intestinal inflammation and enhances epithelial barrier function in piglets. Food and Agricultural Immunology, 31(1), 120–135. https://doi.org/10.1080/09540105.2019.1705766
- Zhang, Y., & Dong, C. (2007). Regulatory mechanisms of mitogen-activated kinase signaling. Cellular and Molecular Life Sciences, 64(21), 2771–2789. https://doi.org/10.1007/s00018-007-7012-3
- Zhang, H., Jin, Y., Peng, A., Guo, S., Loor, J., & Wang, H. (2019). L-arginine protects ovine intestinal epithelial cells from lipopolysaccharide-induced intestinal barrier injury. Food and Agricultural Immunology, 30(1), 1067–1084. https://doi.org/10.1080/09540105.2019.1664417
- Zhong, J., Gong, Q., & Mima, A. (2017). Inflammatory regulation in diabetes and metabolic dysfunction. Journal of Diabetes Research, 2017, 5165268. https://doi.org/10.1155/2017/5165268
- Zhu, Z., Zhang, Q., Chen, L., Ren, S., Xu, P., Tang, Y., & Luo, D. (2010). Higher specificity of the activity of low molecular weight fucoidan for thrombin-induced platelet aggregation. Thrombosis Research, 125(5), 419–426. https://doi.org/10.1016/j.thromres.2010.02.011