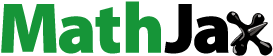
ABSTRACT
Competitive enzyme-linked immunosorbent assay (ELISA) is the most widely used method for rapidly screening of mycotoxins in contaminated food products; however, it involves the use of toxin-protein conjugates as a competitive antigen and toxin standards as calibrators. In this work, a single domain heavy chain antibody (VHH)-based environmentally friendly ELISA (VHH-ELISA) was developed to measure citrinin concentration in Monascus-fermented rice. With this novel indirect competitive VHH-ELISA, the use of a synthetic antigen and pure toxins for operation and environmental safety simultaneously avoided. The assay exhibited a limit of detection of 2.25 ng/mL, with a linear range of 5.0−500.0 ng/mL. VHH-ELISA was then utilized for sample testing, and excellent agreement was observed between VHH-ELISA and high-performance liquid chromatography as a reference. This study provided a novel platform to develop environmentally friendly immunoassays for other toxic small molecules in the application of anti-idiotypic VHHs.
Introduction
Immunoassays are the most widely used methods for rapidly screening of chemical pollutants in contaminated food products because of their excellent specificity, feasibility, and superior sensitivity (Tang et al., Citation2018; Wang et al., Citation2018; Zhang et al., Citation2017). In 2017, the global market share for food testing kits was USD 1.68 billion, which is projected to reach USD 2.38 billion by 2022; immunoassay-based food safety testing kits, such as ELISA kits and immunochromatograph test strips, accounted for the largest proportion in 2017 (R & M Co., Citation2018).
Chemical pollutants are small molecules that cannot be recognized simultaneously by two antibodies, so the format of competitive immunoassay has been developed to detect and quantify small molecules; however, a hapten is needed to compete with the hapten–protein conjugates (termed as competing or coating antigen) for antibody binding (Kavanagh et al., Citation2015; Kobayashi & Oyama, Citation2011). Besides specific antigen-binding antibody, a detection antigen (hapten-protein conjugates) is an extremely essential element in the development of commercial immunoassay kits of chemical pollutants (Lin et al., Citation2016; Lin et al., Citation2017; Zhou & Tang, Citation2020). The preparation of hapten-protein conjugates has long-term dependence on chemosynthetic methods (Esteve-Turrillas et al., Citation2018; Kalayu et al., Citation2017; Parra et al., Citation2012; Sathe et al., Citation2014; Singh et al., Citation2019). However, the preparation cost of traditional chemical hapten-conjugates is expensive (e.g. citrinin, which is a mycotoxin; Table S1), leading to an exorbitant price of the commercial immunoassay kits against those rapidly applied to agricultural products in developing countries. Therefore, safe, cost-effective and renewable surrogates of hapten and hapten-conjugates for immunoassays should be developed.
Single variable domain antibody fragments (VHHs), also called nanobodies because of their nanometer size, are derived from a subset of antibodies completely devoid of light chains in camelids (Muyldermans, Citation2013). In comparison with conventional antibodies, VHHs contain only three complementary determining regions (CDRs), resulting in a CDR3 region presenting a long protruding loop for antigenic binding and ideally shaped for interacting with cavities or crevices of target proteins (De Genst et al., Citation2006; MacCallum et al., Citation1996). Haptens are normally binding epitopes located in concave clefts (Collis et al., Citation2003; Spinelli et al., Citation2001). This structure of VHHs shows a clear advantage over conventional antibodies in the mimicry of small molecular haptens (Zarebski et al., Citation2005). The production of VHHs is simple, quick, low-cost, and high homogeneity because of some peculiar properties of VHHs, including high solubility and ease expression process in microbial systems (Gorlani et al., Citation2012; Zarschler et al., Citation2013). Thus, in comparison with immunoassays based on traditional chemically synthesized antigens, novel developed detection methods employing biosynthetic antigens of VHHs display preferable cost advantages and product stability, so they are suitable for commercial production and widespread use. Accordingly, several anti-idiotypic VHHs (AI-VHHs), including those for mycotoxin zearalenone, fumonisin B1, aflatoxin B1, Ochratoxin A, deoxynivalenol (DON), and other toxins, have been developed as toxin surrogates in immunoassays (Hao et al., Citation2020; Liu et al., Citation2018; Qiu et al., Citation2016; Shu et al., Citation2019; Wang et al., Citation2013; Wang et al., Citation2016; Zhang et al., Citation2019). However, these determination methods for mycotoxin surveillance only avoid the application one of the chemically synthetized mycotoxin conjugates or mycotoxin detection standard. Few studies have been conducted on unalloyed mycotoxin-free immunoassays by simultaneously using AI-VHHs as substitutes of mycotoxin conjugates and mycotoxin detection standard.
According to the principle of competitive format immunoassay, reforming antibody molecules with high antigen-binding affinities is the most frequently used strategy for improving sensitivity. Obtaining low-affinity competitive antigens for target antibodies through screening libraries that contain candidates of antigens with different affinity properties can also achieve the same goal. In view of this policy, the sensitivity of a competitive immunoassay can be significantly improved in accordance with an antigen-mediated principle. However, few studies have focused on this strategy.
A lower affinity of AI-VHH can be directly acquired from a naive library (Xu et al., Citation2015b). As such, a larger naive VHH library has a high probability of containing different affinity constants of AI-VHHs. The AI-VHH with the highest affinity can be used as a pure toxin, whereas the one with the lowest affinity is an ideal candidate as a coating competitive antigen. AI-VHH with high avidity may also compete with coating antigen for the binding sites of antibody, which can exhibit a similar competitive inhibition curve of a pure toxin, because of discrepancies in affinity. Thus, the AI-VHH seems to be a good candidate of surrogate calibrator candidate.
In this study, this hypothesis was tested by constructing a naive camel VHH library with a good diversity. Then, AI-VHHs were screened in terms of mimicry of citrinin (CIT) with a significant difference in affinity constants. The selected couple of AI-VHHs was used as a coating antigen and a mycotoxin standard surrogate. Lastly, an AI-VHH-mediated green immunoassay for CIT without mycotoxin was successfully developed. This study also provided a novel model to develop low-cost, high-sensitivity, and eco-friendly immunoassays for other toxic chemical pollutants.
Materials and methods
Chemicals and reagents
The anti-CIT monoclonal antibody (McAb) 4G6 used in this study was previously prepared in our laboratory. Mycotoxin CIT, bovine serum albumin (BSA), ovalbumin (OVA) and goat anti-mouse McAb-horseradish peroxidase (HRP) were obtained from Sigma (St. Louis, MO). Mouse anti-M13 phages McAb-HRP (Code:27-9421-01) and His-Trap Ni2+-NTA Sepharose columns (1 mL) were purchased from GE Healthcare (Piscataway, NJ). 3, 3′-5, 5′-Tetramethylbenzidine (TMB) substrate was purchased from Bio Basic Inc. (Toronto, Ontario). Other reagents of analytical grade or chromatographic were purchased from Sinopharm Chemical Corp. (Shanghai, China).
Construction of naive camel VHH library
Peripheral blood lymphocytes were isolated through Ficoll gradient centrifugation from 200 mL fresh blood of ten non-immunized Bactrian camels (Camelus bactrianus). Total RNA was extracted from 2 × 108 lymphocytes, reverse-transcribed and applied to produce a naive camel VHH library. The VHH genes were amplified through two-step nested PCR. In the first PCR, the regions of variable domains of the heavy chain (VH) were amplified using three different template concentrations (2, 5 and 10 μL) of cDNA with the primers CALL001 (GTCCTGGCTGCTCTTCTACAAGG) and CALL002 (GGTACGTGCTGTTGAACTGTTCC) that annealed to a well-conserved region of the leader signal sequence and the region of the second constant heavy-chain domain (CH2) of immunoglobulins (Linden et al., Citation2000). The first PCR fragments were loaded on a 1% agarose gel to separate conventional VH, and purified using gel extraction kit (Takara). From these PCR products, the VHH genes were re-amplified with the VHH-FR1 and VHH-shortR1/shortR2/longR primers ((a)) that contained SfiI and NotI restriction enzymes sites and annealed to VHH framework 1 and three different hinge regions of various camel IgG subclasses (IgG3, IgG2a, and IgG2c), respectively (Genst, et al., Citation2006). The second PCR fragments of around 500 bp were gel-purified.
Figure 1. (a) Primers used for amplification of camel VHH encoding regions Primer VHH-FR1 contains a SfiI site (lowercase), VHH-shortR1/shortR2/longR primers contain NotI restriction enzymes sites and anneal to three different hinge regions of various camel IgG subclasses (IgG3, IgG2c, and IgG2a), respectively. (b) Amplicons of stepwise PCR to recover VHH gene. Amplicons of the first PCR (left) with around 700 bp sizes of the VHH gene and part of hinge sequences were cut and gene cleaned (Lanes 1–3 representing cDNA input amount with 2, 5, 10 µL, respectively). Recovered DNAs were used as templates for the second PCR (right) to recover around 400 bp of VHH gene (Lanes 1–3 representing amplicons of IgG3, IgG2c, and IgG2a, respectively). (c) The correct insertion rate detected by colony PCR of 24 individual clones was over 90%. (d) 15 clones were randomly chosen from correct insertion clones above for sequencing to detect the diversity of the library. Alignment of amino acid sequence of 12 correct clones. The correct rate was 80%.
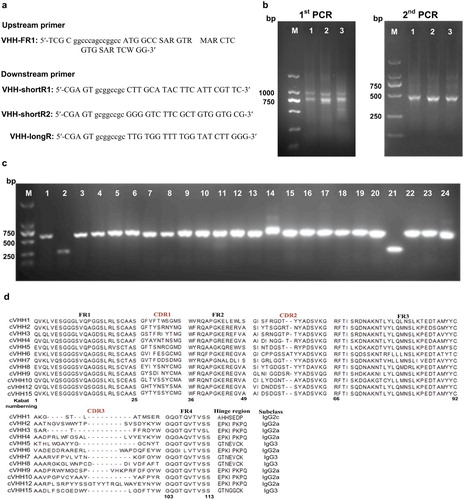
The purified VHH fragments and the phagemid pHEN1 were digested with SfiI and NotI and gel purified. 2 μg digested VHH fragments and 0.6 μg phagemid pHEN1 were ligated by T4 DNA ligase (Fermentas) at 4°C overnight in a total volume of 0.4 mL. After heat-inactivated at 65°C for 10 min, the ligation product was extracted by DNA Fragment Purification Kit (Takara) and eluted in 30 μL sterile milli-Q water. 5 μL of aliquots were electroporated into 50 μL freshly prepared E. coli TG1 electrocompetent cells. Transformation products were pooled and plated onto 6 Lauria-Bertani (LB) agar plates (Φ150 mm) containing 1% glucose and 100 μg/mL ampicillin. Library size was estimated through culturing 10 μL independent serial dilutions of transformed cells on LB plates. Colony PCR was performed on randomly selected colonies to confirm the cloning process. Transformants were grown at 37°C overnight, then scraped off the plates by LB broth containing 20% glycerol and stored at −80°C for further use.
Biopanning of phage displayed AI-VHHs for CIT
Approximately 0.5 mL of a library culture was amplified in 2 × YT medium (1% Yeast Extract, 1.6% Tryptone and 0.5% NaCl, pH 7.0) and infected with M13K07 helper phage. After amplification, the phage particles were precipitated with a PEG/NaCl solution (20% polyethylene glycol 8,000 and 2.5 M NaCl) and redissolved in PBS. Phage titers were estimated in terms of counting colony forming units (c.f.u).
Microplate wells were coated with 100 μL of anti-CIT McAb 4G6 (100 μg/mL) solution at 4°C overnight to select VHH that specifically recognized the paratope of CIT-McAb. Nonspecific binding sites of the wells were blocked by incubating with 3% BSA or OVA solution at 37°C for 1 h. In the first round of panning, 100 μL of VHH phage-display library (1.2 × 1011 c.f.u) was added to the well containing anti-CIT McAb 4G6 and incubated at 37°C for 1 h. The phages were washed 10 times with PBST (containing 0.1% Tween-20). The binding phages were eluted using 100 μL of 0.2 M glycine-HCl (pH 2.2) and neutralized with 1 M Tris-HCl (pH 9.0). The elution solution was then amplified for the subsequent rounds of panning. In the three subsequent rounds of panning, the concentrations of the coating antibody were reduced to 70, 50, and 10 μg/mL. Elution buffer was changed to 100 μL of CIT standard for competitive elution (1.0, 0.5, and 0.05 μg/mL in 10% [v/v] methanol/PBS, respectively). After four rounds of selection, 96 individual clones were randomly selected from 2 × YT-GA (2% glucose and 100 μg/ml ampicillin) plates and rescued on a small scale in a 96-well deep-well plate (1 mL/well); their specific McAb binding activities were determined through phage competitive ELISA as previously described (Xu et al., Citation2015b). Phage VHH clones, which showed an inhibitory binding effect on anti-CIT McAb via the CIT standard, were identified as positive clones and subsequently sequenced.
Expression of AI-VHH
The selected clones were subcloned into pET-25b (+) (Novagen) and transformed into Rosetta (DE3) cells to express soluble AI-VHHs. Proteins were expressed and purified as our previous report (Xu et al., Citation2015a). The purity and size of the prepared soluble AI-VHH were verified through 15% SDS- polyacrylamide gel electrophoresis (SDS-PAGE). The concentrations of the purified AI-VHHs were determined with the bicinchoninic acid (BCA) method (Preety et al., Citation2018) and stored at −20 °C for the next use.
Characterization of AI-VHH
A conventional ELISA with CIT-OVA as a coating antigen was developed to evaluate the simulation authenticity of soluble AI-VHHs for CIT used as surrogates of the CIT standard solution. In brief, the plates were coated with 0.17 μg/well of CIT-OVA in PBS and incubated at 4°C overnight. The plates were then blocked with 4% skim milk in PBS at 37°C for 1 h. A mixture of 50 μL of McAb 4G6 and 50 μL of serial dilution of CIT standard solution or purified soluble AI-VHH for CIT were added to the plate and incubated at 37°C for 1 h. After the plates were washed, 100 μL of goat anti-mouse McAb-HRP (1:2000) was added to detect the captured McAb 4G6. Absorbance at 450 nm which was determined as described in a previous section.
A comparative study was performed to evaluate the correlation between CIT and CIT surrogates (AI-VHH). A linear regression curve was established by plotting the AI-VHH concentrations (as X axis) in a series of inhibition values in a linear range against CIT concentrations (as Y axis) at the same inhibition levels. The CIT concentration was calculated in two steps. The test inhibition values of the samples were converted to the AI-VHH concentration by using the surrogate calibration curve. The inhibition value was determined as follows: B/B0 × 100, where B is the absorbance with the CIT, and B0 is absorbance without CIT. The surrogate calibrator curve was drawn on the basis of a linear logistic equation:
where y is the inhibition value, x is the AI-VHH concentration, a is the slope parameter, b is the intercept. Then, the AI-VHH concentration was converted into its corresponding CIT concentration with the linear regression equation.
The binding kinetics of soluble AI-VHH for CIT to McAb 4G6 was estimated through bio-layer interferometry by using a BLitz™ instrument (ForteBio). A Ni-NTA biosensor (Catalog no. 18-5101, ForteBio) was utilized to immobilize AI-VHH with His-tag in accordance with the manufacturer’s instructions (Abdiche et al., Citation2008). Data were globally fitted to a 1:1 binding model with the built-in BLItz software to determine the association (kon), dissociation (koff), and affinity (KD) constants.
The AI-VHH solution was incubated for 20 min at different temperatures (50°C, 60°C, 70°C, and 80°C) to evaluate the thermal stability of the selected AI-VHH as a surrogate of the standard CIT solution. Its competitive inhibition as a surrogate of the CIT standard solution was plotted as curves as described previously.
Green ELISA for CIT without mycotoxin
A green ELISA for CIT without mycotoxin was constructed as follows. The expressed soluble AI-VHH with the lowest affinity to McAb 4G6 was used as a coating antigen, and incubated in plate wells (0.2 μg/well), and blocked with 5% skim milk in PBS at 37°C for 1.5 h. After the wells were washed with PBST, 50 μL of McAb 4G6 (0.6 μg/well) and 50 μL of a serial concentration of CIT surrogates (AI-VHH with the highest affinity to McAb 4G6) in 10% (v/v) methanol/PBS solution extracts of CIT-negative rice samples were added to the respective wells and incubated at 37°C for 50 min. Afterward, 100 μL of 1:2000 dilution of goat anti-mouse McAb-HRP was added to detect the captured antibody. The standard curve of green ELISA was drawn on the basis of the inhibition value B/B0% correlated with various concentrations of CIT surrogates. The real CIT concentration was calculated by the linear logistic equation of the surrogate calibrator curve.
The practicality and reliability of green ELISA without mycotoxin were determined by analyzing Monascus-fermented rice samples. The ELISA results were further compared with those of high-performance liquid chromatography (HPLC). The level of agreement between the two methods was assessed using intraclass correlation coefficients (ICCs) and a two-way mixed-effects model with consistency of agreement. Statistical analysis was performed using SPSS14.0 (IBM). According to the Rosner’s criteria, ICCs below 0.40, 0.40–0.75, and 0.75 and above indicate poor agreement, fair to good agreement, and excellent agreement, respectively (Rosner, Citation2011).
Preparation of rice samples
Eight CIT-contaminated rice samples were prepared on a laboratory scale. In brief, rice was soaked in pure water at room temperature overnight. Afterward, 15 g of pre-immersed rice was placed in a 250 mL Erlenmeyer flask and mixed well with 10 mL of pure water. The mixture was sterilized at 121°C for 30 min, and 1 mL of the spore solution (approximately 1 × 106 spores/mL) of Monascus aurantiacus, which was identified to produce CIT on a rice culture medium, was inoculated. Eight rice samples were fermented at 28°C under stationary conditions for 14 days.
Monascus-fermented rice samples were pretreated as follows: 1 g of the samples was pulverized and mixed with 5 mL of 10% (v/v) methanol/PBS. The mixture was ultrasonically extracted centrifuged for 20 min and centrifuged at 8,000 g for 10 min. The supernatant was collected and further diluted 50 times with 10% (v/v) methanol/PBS for ELISA detection.
Results and discussion
Construction of a naive camel phage displayed VHH library
Total RNA was isolated from 10 non-immunized Bactrian camels for cDNA synthesis by using random and oligo-dT primers to generate a large and highly diverse VHH naive library. Heavy chains of all immunoglobulins from the leader sequence to the CH2 exon were amplified through PCR under different conditions to maximally preserve the initial diversity. As shown in (b), VHHs (∼ 750 bp) were separated from the conventional VH containing the CH1 region (∼ 1,000 bp) on agarose gel and purified. In the second step of PCR, three subtypes of VHH (IgG3, IgG2a, and IgG2c) were re-amplified using degenerated primers that annealed to three hinge regions and introduced the SfiI/NotI restriction sites as well ((b)). After the ligated VHH genes and the phagemid vector pHEN1 were transformed into E. coli TG1 cells, the primary size of the library contained about 7.2 × 108 independent colonies based on the dilution plating of the cultured library.
The functional library dimension was evaluated through colony PCR, and the assay performed with 24 random clones confirmed the presence of an insert having the expected size (around 600 bp) in more than 90% of the analyzed clones ((c)). Afterward, 15 clones were arbitrarily picked from the 22 correct clones above and sequenced to evaluate the diversity of the library. The sequencing results showed that 80% of the clones expressed right VHH sequences. Moreover, the deduced amino acid sequence of the VHH protein was further compared using the web-based programme IGMT/3Dstructure-DB. As presented in (d), the analyzed sequences covered three VHH subtypes, and five clones contained an additional pair of cysteines located in CDR1 and CDR3 regions. This feature was typical in the case of Camelus bactrianus and Camelus dromedarius (Genst, et al., Citation2006).
The actual size of the VHH library was 5 × 108. Considering that we started from 2 × 108 lymphocytes, the proportion of B cell-producing antibodies was 20%–30% of the total of lymphocytes, and VHHs represented 50% of the total camel antibodies (Monegal et al., Citation2009; Sabir et al., Citation2014). The theoretical maximal library diversity would be 3 × 107, so the size of the naive camel VHH library covered the whole genetic diversity based on the initial lymphocytes. The titer of the naive phage-displayed VHH library was 1.2 × 1013 c.f.u/mL after it was rescued by M13K07 helper phage infection.
Selection of phage displayed AI-VHH for CIT
A solid-phase competition elution strategy with a subtractive CIT concentration in 2–4 panning rounds was applied to bio-panning specific AI-VHHs for CIT. The enrichment factor of the output phage increased to 126-fold (from 2.1 × 106 c.f.u to 2.7 × 108 c.f.u) after four rounds of biopanning were performed. Ninety-six random phage clones from the fourth panning round were selected and identified through phage ELISA. The results showed that over 60% of competitive eluted VHH phages showed an inhibitory binding activity to the primary McAb 4G6 by CIT standard (100 ng/mL, Figure S1). The sequencing of the positive clones revealed five different amino sequences of AI-VHHs (Phage-Clone No. A9, A7, C1, C2, and D5; (a)). Sequence alignment analysis showed five clones with high specificity to McAb 4G6 by presenting nearly the same three CDRs, whereas several different amino sequences in conserved regions (FRs) might be contributed to influence the binding affinity of isolated clones. This possibility prompted us to further analyze relevant properties of these AI-VHHs. The competitive inhibition curves of five verified phage displayed AI-VHH were established ((b)). Among the curves, the P-A9 clone had the lowest IC50 (14.28 ng/mL). By contrast, P-D5 and P-C1 showed higher IC50 (141.43 and 211.18 ng/mL, respectively), indicating that the binding affinity of P-D5 and C1 to McAb 4G6 could be higher than that of P-A9.
Figure 2. (a) Sequence alignment of five positive colonies. (b) Calibration curves of five clones for CIT analysis by phage ELISA. Binding percentages were calculated as follows: B/B0 × 100%, herein B is the absorbance of CIT and phage AI-VHHs and B0 is the absorbance of the blank solution. (c) Performance of the five purified AI-VHH fragments using as a surrogate of CIT standard in conventional indirect competitive ELISA. Error bars are standard deviations of the mean with n = 3.
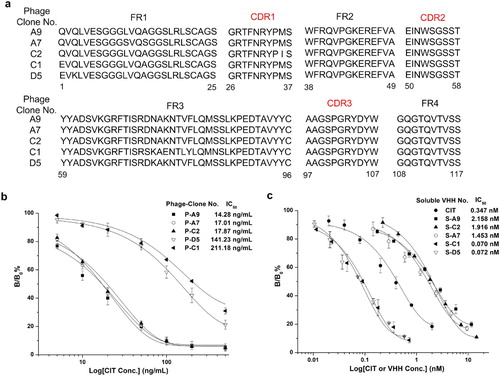
Characterization of soluble AI-VHH
The phage format of AI-VHH was difficult to quantify accurately, so soluble AI-VHHs were expressed through periplasmic preparation in E. coli (Figure S2). The simulation authenticity of five positive clones was further identified with soluble fragments (named as S-A9, S-A7, S-C1, S-C2, and S-D5) through indirect competitive ELISA. In (c), the inhibition values of immunoassay declined as AI-VHH concentration increased, indicating that the prepared soluble AI-VHHs still exhibited a competitive binding ability to McAb 4G6 with CIT-OVA conjugates. Furthermore, IC50 of the CIT surrogates S-D5 and S-C1 (0.072 and 0.070 nM, respectively) in ELISA were significantly lower than that of ELISA based on the natural CIT solution (IC50 = 0.347 nM).
The reaction kinetics between soluble AI-VHH (S-A9, S-D5, and S-C1) and McAb 4G6 was detected through bio-layer interferometry analysis. In , the sensorgram curves obtained were well behaved, and the binding response unit caused by McAb 4G6 binding increased as the concentration of the antibody increased. This result was in accordance with the indirect immunoassay principle. S-A9:4G6, S-D5:4G6, S-C1:4G6, and CIT-OVA: 4G6 had KD of 154, 62.5, 38.4, and 68 nM, respectively, indicating that the binding affinities of S-D5 and S-C1 to 4G6 were higher than that of chemosynthetic CIT antigen.
Figure 3. The kinetics binding analysis of AI-VHHs to McAb 4G6 was performed by bio-layer interferometry (BLItz) Hexa-Histidine fused VHH was immobilized on Ni-NTA biosensors. (a-c) Sensorgrams showing the interaction between VHH (S-A9, S-D5, S-C1) and anti-CIT McAb, respectively. (d) The results of determination of kinetics and equilibrium dissociation constants. # The kinetic binding result of CIT-OVA to McAb was determined in our previous study (Xu et al., Citation2015a).
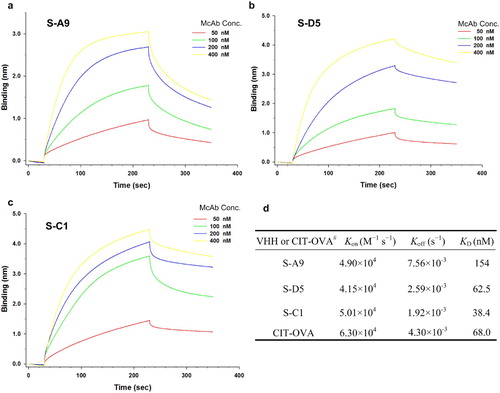
To improve the sensitivity of hapten competitive immunoassay, the main strategies focus on creating antibody molecules with higher antigen-binding affinities through affinity maturation in vitro and vivo based on bioengineering technology (Kobayashi & Oyama, Citation2011; Sheedy et al., Citation2007) and optimizing the chemical structure of immunizing haptens through computer molecular modeling studies (Ballesteros et al., Citation1998). However, no particular hapten design and immunization strategy could significantly influence the antibody characteristics regarding immunoassay sensitivity and selectivity (Lee et al., Citation1998; Sanvicens et al., Citation2012). So far, studies have yet to further investigate how the affinity modification of competitive detection antigen influences the immunoassay sensitivity. In our previous work, the sensitivity of immunoassay with AI-VHH (named N-28) as a DON surrogate can be improved through site-saturation mutagenesis to change the functional amino acid sequence of the primary N-28 antibody. Structural analysis revealed that the affinity of the mutant N-28-T102Y decreased because of the increased steric hindrance with a large side chain. Thus, we speculated that a lower binding-affinity mimetic antigen might contribute to the improvement of the sensitivity of competitive immunoassays (Qiu et al., Citation2016). In the present work, S-A9 and S-C1 were selected to work as coating antigens in ELISA and validate whether the coating-detection antigen with a lower affinity to McAb could improve the sensitivity of immunoassay. Standard curves were then conducted. In , the sensitivity of AI-VHH-ELISA with S-A9 (IC50 = 46.3 ng/mL) as a coating antigen was better than that of the S-C1-based ELISA (IC50 = 303.6 ng/mL). The result was consistent with our predictions, demonstrating that S-A9 with a lower affinity was a suitable coating antigen, and S-C1 was an appropriate standard CIT solution surrogate.
Figure 4. Inhibition curves of VHH-ELISA using AI-VHH S-A9 and S-C1 as coating antigens, respectively. Serial dilutions of CIT standards (5.0, 10.0, 20.0, 50.0, 100.0, 200.0, and 500.0 ng/mL) in 10% methanol/PBS were mixed with an equal amount of anti-CIT McAb 100 μL of the mixture was added into wells. The captured McAbs were detected by adding 100 μL of mouse anti-mouse McAb-HRP (1:2000). Error bars are standard deviations of the mean with n = 3.
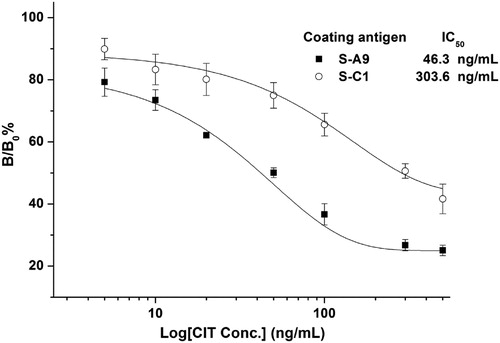
AI-VHH mediated ELISA for CIT
Before the development of AI-VHH mediated ELISA for CIT without mycotoxin, the quantitative conversion relation between the CIT surrogates (AI-VHH) and the natural CIT standard solution was determined. In this work, S-A9, which showed the lowest affinity to McAb 4G6, was used as a coating antigen. A serial concentration of CIT surrogates (S-C1; 0.1, 0.2, 0.4, 0.8, 1.6, 3.2, 6.4, 12.8, and 25.6 μg/mL) and natural standard CIT solution (0, 2.5, 5.0, 10.0, 20.0, 50.0, 100.0, 200.0, 300.0, and 500.0 ng/mL) were mixed with McAb 4G6 and added to the S-A9 coated wells, respectively. As shown in (a and b), the calibrator inhibition curve of the surrogate was similar to that of the CIT standards. When the concentrations of CIT were 5.0–500.0 ng/mL (corresponding inhibition value B/B0% = 25–80%), the standard CIT curve fitted with a linear logistic equation:(1)
(1)
Figure 5. Calibration curves using CIT standard (a) and surrogate of AI-VHH C1 (b), respectively. Error bars are standard deviations of the mean with n = 5. (c) Correlation of results between AI-VHH C1 and CIT. The linear regression analysis yielded a good correlation between the calibrators (y = 58.50x – 16.31, R2 = 0 999). (d) The thermal stability of AI-VHH C1 as standard surrogate was estimated by VHH-ELISA using AI-VHH A9 as coating antigen. The solutions of C1 were treated for 20 min at different temperatures. Then, a gradient double ratio dilution of C1 solutions were mixed with an equal volume of anti-CIT McAb and 100 μL of the mixture was added into wells. Error bars are standard deviations of the mean with n = 3.
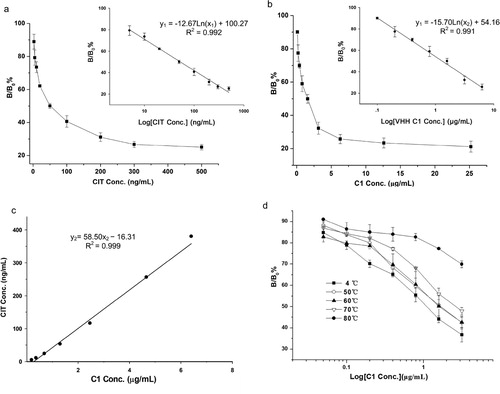
where y1 is the inhibition value, and x1 is the CIT concentration ((a)). Similarly, a linear logistic equation of the S-C1 concentration between 0.1 and 6.4 μg/mL (corresponding B/B0% = 25 − 90%) was obtained:(2)
(2)
where y1 is the inhibition value and x2 is the S-C1 concentration ((b)). On the basis of the coincident linear range of the two standard curves above, we calculated the concentrations of CIT and S-C1 corresponding to the same inhibition value between 25% and 80% and established a linear relationship. In (c), the linear regression equation obtained from the plot of the standard CIT concentrations (y2) against the S-C1 concentration (x2) was expressed as follows:(3)
(3)
Overall, a quantitative conversion correlation between S-C1 and CIT was successfully established. These results clearly demonstrated that the S-C1 could replace CIT as a new standard material for indirect competitive ELISA.
Previous studies showed that the good thermostability of reagents indicates high stability and long shelf life in immunoassay product development (Wang et al., Citation2013; Zhang et al., Citation2019). In the present study, the thermal stability of S-C1 used as a CIT substitute was evaluated. The S-C1 solutions (3.2 μg/mL) were incubated for 20 min at 50°C, 60°C, 70°C, and 80°C. Afterward, they were cooled to room temperature, and their competitive inhibition as a surrogate of CIT was plotted as a curve with a gradient double ratio dilution of C1 concentrations. In (d), the competitive inhibition rate of S-C1 increased as temperatures increased from 50°C to 80°C. This result indicated that the binding capacity of the treated S-C1 with anti-CIT McAb 4G6 gradually weakened as temperature increased. However, in comparison with the inhibition curve of S-C1 stored at 4°C, the change in the curve shape was distinct at 80°C. Consequently, S-C1 retained its competitive binding ability after it was heated at 70°C for 20 min. These results revealed that the thermostability of S-C1 was good, so it was a suitable alternative reagent for immunoassays.
Validation of AI-VHH-mediated ELISA for CIT
Two-step calculation was conducted to detect CIT by using the developed AI-VHH-based green ELISA with a surrogate calibrator. First, the B/B0 value of each sample was obtained in terms of OD450nm measured with VHH-based ELISA. From this value, the corresponding S-C1 concentration was calculated using Equation 2. Second, the S-C1 concentration was converted into the CIT concentration via quantitative conversion (Equation 3). With this two-step approach, the novel developed AI-VHH-based green ELISA exhibited a detection limit of 2.25 ng/ml (IC10) and a good linear range for the CIT detection of 5.0−500.0 ng/mL (IC25−IC80) for CIT detection.
A comparative analysis in the eight Monascus-fermented rice samples between AI-VHH-mediated ELISA and HPLC method was conducted to assess the practicality and reliability of the novel green ELISA. The results of green ELISA agreed with those of HPLC (). The result of ICC was 0.976 with 95% confidence interval (0.886−0.995). Based on Rosner’s criterion, excellent agreement was found between these two methods.
Table 1. CIT detection results of green ELISA and HPLC in Monascus-fermented rice samples.
Conclusions
In this study, a green ELISA method based on AI-VHHs was developed. The surrogate calibrator curve and the CIT standard curve had similar shapes. A good correlation (R2 = 0.999) was observed between the two standard materials. The results of the green ELISA of Monascus-fermented rice samples were also consistent with those of HPLC. Once the quantitative conversion correlation between CIT and S-C1 expressed per batch was established, only S-C1 as a calibrator was needed for the detection and quantification of CIT in the samples, and CIT standards were no longer necessary. The novel assay minimized the impact of toxins on both the environment and operators. However, AI-VHH should be selected and separated for each primary antibody that binds to a specific antigen, because AI-VHH depends on amino acid residues to mimic antigens and bind to antibody epitopes. Even though, in comparison with conventional AI-VHH preparation, the proposed strategy could avoid complex animal immunity process, which directly isolated AI-VHHs as small molecular mimicry from a naive camel VHH library. Naive VHH libraries are theoretically universal, allowing the selection of AI-VHHs for mimicry of any target, especially toxic and small molecular chemical pollutants. Meanwhile, VHHs are suitable alternative reagents for immunoassays because of their properties, including easy production in microbial systems, high solubility, high thermal and solvent tolerance, and sustainable source. This study provided a novel platform to develop a green analytical method for other toxic small molecules in AI-VHH applications.
Supplemental Material
Download MS Word (3.3 MB)Disclosure statement
No potential conflict of interest was reported by the author(s).
Additional information
Funding
References
- Abdiche, Y., Malashock, D., Pinkerton, A., & Pons, J. (2008). Determining kinetics and affinities of protein interactions using a parallel real-time label-free biosensor, the Octet. Analytical Biochemistry, 377(2), 209–217. https://doi.org/10.1016/j.ab.2008.03.035
- Ballesteros, B., Barceló, D., Sanchez-Baeza, F., Camps, F., & Marco, M. P. (1998). Influence of the hapten design on the development of a competitive ELISA for the determination of the antifouling agent Irgarol 1051 at trace levels. Analytical Chemistry, 70(19), 4004–4014. https://doi.org/10.1021/ac980241d
- Collis, A. V., Brouwer, A. P., & Martin, A. C. (2003). Analysis of the antigen combining site: Correlations between length and sequence composition of the hypervariable loops and the nature of the antigen. Journal of Molecular Biology, 325(2), 337–354. https://doi.org/10.1016/S0022-2836(02)01222-6
- De Genst, E., Silence, K., Decanniere, K., Conrath, K., Loris, R., Kinne, J., Muyldermans, S., & Wyns, L. (2006). Molecular basis for the preferential cleft recognition by dromedary heavy-chain antibodies. Proceedings of the National Academy of Sciences, 103(12), 4586–4591. https://doi.org/10.1073/pnas.0505379103
- Esteve-Turrillas, F. A., Agulló, C., Mercader, J. V., Abad-Somovilla, A., & Abad-Fuentes, A. (2018). Rationally designed haptens for highly sensitive monoclonal antibody-based immunoanalysis of fenhexamid. The Analyst, 143(17), 4057–4066. https://doi.org/10.1039/C8AN00827B
- Genst, E. D., Saerens, D., Muyldermans, S., & Conrath, K. (2006). Antibody repertoire development in camelids. Developmental and Comparative Immunology, 30(1-2), 187–198. https://doi.org/10.1016/j.dci.2005.06.010
- Gorlani, A., de Haard, H., & Verrips, T. (2012). Expression of VHHs in Saccharomyces cerevisiae. Methods in Molecular Biology, 911, 277–286. https://doi.org/10.1007/978-1-61779-968-6_17
- Hao, J., Li, Y., Wang, J., Xu, C., Gao, M., Chen, W., Zhang, X., Hu, X., Liu, Y., & Liu, X. (2020). Screening and activity identification of an anti-idiotype nanobody for Bt Cry1F toxin from the camelid naive antibody phage display library. Food and Agricultural Immunology, 31(1), 1–16. https://doi.org/10.1080/09540105.2019.1691156
- Kalayu, Y. S., Ling, S., Yang, Y., Yuan, J., & Wang, S. (2017). The preparation and identification of a monoclonal antibody against citrinin and the development of detection via indirect competitive ELISA. Toxins, 9(3), 110. https://doi.org/10.3390/toxins9030110
- Kavanagh, O., Elliott, C. T., & Campbell, K. (2015). Progress in the development of immunoanalytical methods incorporating recombinant antibodies to small molecular weight biotoxins. Analytical and Bioanalytical Chemistry, 407(10), 2749–2770. https://doi.org/10.1007/s00216-015-8502-z
- Kobayashi, N., & Oyama, H. (2011). Antibody engineering toward high-sensitivity high-throughput immunosensing of small molecules. The Analyst, 136(4), 642–651. https://doi.org/10.1039/C0AN00603C
- Lee, N. J., McAdam, D. P., & Skerritt, J. H. (1998). Development of immunoassays for type II synthetic pyrethroids. 1. Hapten design and application to heterologous and homologous assays. Journal of Agricultural and Food Chemistry, 46(2), 520–534. https://doi.org/10.1021/jf970438r
- Lin, Y., Zhou, Q., & Tang, D. (2017). Dopamine-loaded liposomes for in-situ amplified photoelectrochemical immunoassay of AFB1 to enhance photocurrent of Mn2+-doped Zn3(OH)2V2O7 nanobelts. Analytical Chemistry, 89(21), 11803–11810. https://doi.org/10.1021/acs.analchem.7b03451
- Lin, Y., Zhou, Q., Tang, D., Niessner, R., Yang, H., & Knopp, D. (2016). Silver nanolabels-assisted ion-exchange reaction with CdTe quantum dots mediated exciton trapping for signal-on photoelectrochemical immunoassay of mycotoxins. Analytical Chemistry, 88(15), 7858–7866. https://doi.org/10.1021/acs.analchem.6b02124
- Linden, R. V. D., Geus, B. D., Stok, W., Bos, W., Wassenaar, D. V., Verrips, T., & Frenken, L. (2000). Induction of immune responses and molecular cloning of the heavy chain antibody repertoire of Lama glama. Journal of Immunological Methods, 240(1-2), 185–195. https://doi.org/10.1016/S0022-1759(00)00188-5
- Liu, Y., Lin, M., Wu, J., Hu, X., Zhang, X., Xu, C., … Liu, X. (2018). Generation of panels of anti-idiotypic single-chain variable fragments mimicking Cry2Aa toxin using the chain shuffling technique. Food and Agricultural Immunology, 29(1), 735–743. https://doi.org/10.1080/09540105.2018.1440535
- MacCallum, R. M., Martin, A. C., & Thornton, J. M. (1996). Antibody-antigen interactions: Contact analysis and binding site topography. Journal of Molecular Biology, 262(5), 732–745. https://doi.org/10.1006/jmbi.1996.0548
- Monegal, A., Ami, D., Martinelli, C., Huang, H., Aliprandi, M., Capasso, P., Francavilla, C., Ossolengo, G., & de Marco, A. (2009). Immunological applications of single-domain llama recombinant antibodies isolated from a naïve library. Protein Engineering, Design and Selection, 22(4), 273–280. https://doi.org/10.1093/protein/gzp002
- Muyldermans, S. (2013). Nanobodies: Natural single-domain antibodies. Annual Review of Biochemistry, 82(1), 775–797. https://doi.org/10.1146/annurev-biochem-063011-092449
- Parra, J., Mercader, J. V., Agulló, C., Abad-Somovilla, A., & Abad-Fuentes, A. (2012). Generation of anti-azoxystrobin monoclonal antibodies from regioisomeric haptens functionalized at selected sites and development of indirect competitive immunoassays. Analytica Chimica Acta, 715, 105–112. https://doi.org/10.1016/j.aca.2011.12.014
- Preety, Chauhan, N., Sharma, S., & Hooda, V. (2018). Improved protein determination assays obtained after substitution of copper sulfate by copper oxide nanoparticles. Analytical Biochemistry, 547, 19–25. https://doi.org/10.1016/j.ab.2018.02.011
- Qiu, Y., He, Q., Xu, Y., Wang, W., & Liu, Y. (2016). Modification of a deoxynivalenol-antigen-mimicking nanobody to improve immunoassay sensitivity by site-saturation mutagenesis. Analytical and Bioanalytical Chemistry, 408(3), 895–903. https://doi.org/10.1007/s00216-015-9181-5
- R & M Co. (2018). Food testing kits market by target tested (pathogens, meat species, GMOs, allergens, mycotoxins), technology (PCR, immunoassay, enzyme substrate based), sample (meat, packaged food, cereals, grains, dairy, nuts), region - global forecast to 2022. (https://www.researchandmarkets.com/reports/4454664).
- Rosner, B. (2011). Fundamental of biostatistics (7th ed., p. 569). Brooks/Cole.
- Sabir, J. S., Atef, A., Domyati, F. M. E., Edris, S., Hajrah, N., Alzohairy, A. M., & Bahieldin, A. (2014). Construction of naïve camelids VHH repertoire in phage display-based library. Comptes Rendus Biologies, 337(4), 244–249. https://doi.org/10.1016/j.crvi.2014.02.004
- Sanvicens, N., Varela, B., Ballesteros, B., & Marco, M. P. (2012). Development of an immunoassay for terbutryn: Study of the influence of the immunization protocol. Talanta, 89, 310–316. https://doi.org/10.1016/j.talanta.2011.12.033
- Sathe, M., Srivastava, S., Merwyn, S., Agarwal, G. S., & Kaushik, M. P. (2014). Competitive immunochromatographic assay for the detection of thiodiglycol sulfoxide, a degradation product of sulfur mustard. The Analyst, 139(20), 5118–5126. https://doi.org/10.1039/C4AN00720D
- Sheedy, C., MacKenzie, C. R., & Hall, J. C. (2007). Isolation and affinity maturation of hapten-specific antibodies. Biotechnology Advances, 25(4), 333–352. https://doi.org/10.1016/j.biotechadv.2007.02.003
- Shu, M., Xu, Y., Dong, J., Zhong, C., Hammock, B. D., Wang, W., & Wu, G. (2019). Development of a noncompetitive idiometric nanobodies phage immunoassay for the determination of fumonisin B1. Food and Agricultural Immunology, 30(1), 510–521. https://doi.org/10.1080/09540105.2019.1604637
- Singh, G., Velasquez, L., & Huet, A. C. (2019). Development of a sensitive polyclonal antibody-based competitive indirect ELISA for determination of citrinin in grain-based foods. Food Additives and Contaminants Part A, 301, 7. https://doi.org/10.1080/19440049.2019.1640895
- Spinelli, S., Tegoni, M., Frenken, L., van Vliet, C., & Cambillau, C. (2001). Lateral recognition of a dye hapten by a llama VHH domain. Journal of Molecular Biology, 311(1), 123–129. https://doi.org/10.1006/jmbi.2001.4856
- Tang, D., Lin, Y., & Zhou, Q. (2018). Carbon dots prepared from Litchi chinensis and modified with manganese dioxide nanosheets for use in a competitive fluorometric immunoassay for aflatoxin B1. Microchimica Acta, 185(10), 476. https://doi.org/10.1007/s00604-018-3012-2
- Wang, X., He, Q., Xu, Y., Liu, X., Shu, M., Tu, Z., Li, Y., Wang, W., & Cao, D. (2016). Anti-idiotypic VHH phage display-mediated immuno-PCR for ultrasensitive determination of mycotoxin zearalenone in cereals. Talanta, 147, 410–415. https://doi.org/10.1016/j.talanta.2015.09.072
- Wang, Y., Li, P., Zuzana, M., Bever, C. R. S., Kim, H. J., Zhang, Q., Dechant, J. E., Gee, S. J., & Hammock, B. D. (2013). Isolation of alpaca anti-idiotypic heavy-chain single-domain antibody for the aflatoxin immunoassay. Analytical Chemistry, 85(17), 8298–8303. https://doi.org/10.1021/ac4015885
- Wang, J., Mukhtar, H., Ma, L., Pang, Q., & Wang, X. H. (2018). VHH antibodies: Reagents for mycotoxin detection in food products. Sensors, 18(2), 485. https://doi.org/10.3390/s18020485
- Xu, Y., Xiong, L., Li, Y., Xiong, Y., Tu, Z., Fu, J., & Tang, X. (2015a). Citrinin detection using phage-displayed anti-idiotypic single-domain antibody for antigen mimicry. Food Chemistry, 177, 97–101. https://doi.org/10.1016/j.foodchem.2015.01.007
- Xu, Y., Xiong, L., Li, Y., Xiong, Y., Tu, Z., Fu, J., & Chen, B. (2015b). Anti-idiotypic nanobody as citrinin mimotope from a naïve alpaca heavy chain single domain antibody library. Analytical Bioanalytical Chemistry, 407(18), 5333–5341. https://doi.org/10.1007/s00216-015-8693-3
- Zarebski, L. M., Urrutia, M., & Goldbaum, F. A. (2005). Llama single domain antibodies as a tool for molecular mimicry. Journal of Molecular Biology, 349(4), 814–824. https://doi.org/10.1016/j.jmb.2005.03.072
- Zarschler, K., Witecy, S., Kapplusch, F., Foerster, C., & Stephan, H. (2013). High-yield production of functional soluble single-domain antibodies in the cytoplasm of Escherichia coli. Microbial Cell Factories, 12(1), 97. https://doi.org/10.1186/1475-2859-12-97
- Zhang, Y., Yang, J., Lu, Y., Ma, D., Qi, M., & Wang, S. (2017). A competitive direct enzyme-linked immunosorbent assay for the rapid detection of deoxynivalenol: Development and application in agricultural products and feedstuff. Food and Agricultural Immunology, 28(3), 516–527. https://doi.org/10.1080/09540105.2017.1306491
- Zhang, C., Zhang, Q., Tang, X., Zhang, W., & Li, P. (2019). Development of an anti-idiotypic VHH antibody and toxin-free enzyme immunoassay for ochratoxin A in cereals. Toxins, 11(5), 280. https://doi.org/10.3390/toxins11050280
- Zhou, Q., & Tang, D. (2020). Recent advances in photoelectrochemical biosensors for analysis of mycotoxins in food. TrAC Trends in Analytical Chemistry, 124, 115814. https://doi.org/10.1016/j.trac.2020.115814