ABSTRACT
Copper has been shown to plays an important role in immune functions. The aim of the study was to determine the effect of inorganic and organic forms of copper on the concentrations of cytokines, acute phase proteins and prostanoids in the peripheral blood and liver of poultry. The concentration of copper in serum and tissues was also determined. A total of 900 1-day-old Ross 308 male chickens were used. The rearing period was 42 days. The material for analysis comprised samples of peripheral blood, liver, intestine and muscle of experimental chickens. The obtained results indicate that the supplementation of broilers feed with copper chelates promotes secretion of pro and anti-inflammatory cytokines. Increased synthesis of the immunoregulatory serum amyloid A (SAA) and alpha-1-acid glycoprotein (α-AGP) and prostanoids following the administration of copper-glycine chelates in the diet indicates the stimulatory effect of copper on cellular and humoral immune mechanisms.
Introduction
The recent ban on the use of antibiotics as feed additives has prompted the feed industry and poultry farmers to search for alternative substances and strategies to modulate the immune system of birds, ensuring increased productivity and welfare (Huyghebaert et al., Citation2011; Patterson & Burkholder, Citation2003). These goals are achieved in part with the use of feed supplemented with various additives, such as trace elements, pro- and prebiotics, enzymes, organic acids, herbal formulations, and detoxifiers (Akinleye et al., Citation2008; Patterson & Burkholder, Citation2003). One important element with documented health-promoting effects is copper (Cu) (Lim & Paik, Citation2006). Copper has been shown to stimulate growth in chickens, to exert antimicrobial effects, to be a component of enzyme systems involved in metabolic processes regulating the functions of the nervous, digestive and circulatory systems, and to mediate biosynthesis of immunologically active compounds (Baker et al., Citation1991; Klasing, Citation1998; Lim & Paik, Citation2006; Pesti & Bakalli, Citation1996, Citation1998). Copper plays an important role in immune response processes (Chatterjee et al., Citation2009; Jarosz et al., Citation2018). Copper deficiency is associated with developmental disturbances of the lymphoid organs, a decrease in the percentage of T cells, impairment of their maturation in the spleen and reactivity to mitogens, and a reduction in antibody synthesis and the phagocytic index of cells (Das et al., Citation2014; Hong et al., Citation2002; Jarosz et al., Citation2018; Lim & Paik, Citation2006). Copper deficiency also delays the acute phase response, which is linked to impairment of synthesis of acute phase proteins (APP) such as ceruloplasmin, cytokine synthesis, and the generation of reactive oxygen species during phagocytosis (Das et al., Citation2014; Jarosz et al., Citation2018).
Excess copper in the diet also has a negative effect (Karimi et al., Citation2011). Copper concentrations above 120 mg/kg in poultry feed have been shown to lead to metabolic disorders and impairment of the structure of cells of the spleen, liver and intestine (Attia et al., Citation2011). The copper compounds most commonly used as poultry feed additives are inorganic salts, carbonates, chlorides, oxides, and sulphates (Jegede et al., Citation2012; Wang et al., Citation2011; Zhao et al., Citation2010). In poultry farming, to avoid deficiencies of trace metals and increase the growth of birds, these compounds are often used in excessive amounts, 2–10 times higher than recommended by the National Research Council (Esenbuğa et al., Citation2008; Zhao et al., Citation2010). However, an increased concentration of trace minerals, including copper, in feed can interfere with the bioavailability of other elements (Wapnir, Citation1998). Organic forms of this element show more favourable effects in poultry in terms of absorption and availability. Used as feed additives, they also exert positive health-promoting effects (Attia et al., Citation2011; Jarosz et al., Citation2018; Jegede et al., Citation2012). One of the organic copper compounds most commonly used as a feed additive for poultry is copper-methionine chelate (Cu-Met) (Chowdhury et al., Citation2004; Lim & Paik, Citation2006). It has been demonstrated that the use of Cu in chelated form makes it possible to reduce its concentrations in feed, which improves production while at the same time protecting the farm environment (Brugger & Windisch, Citation2015; Paik, Citation2001). With respect to the effect of elements on immunity, it is significant that copper-methionine chelates stimulate maturation of T cells, activation of B cells, and synthesis of antibodies taking part in the immune response (Hong et al., Citation2002; Wang et al., Citation2011). Furthermore, these compounds have been shown to stimulate multiplication of beneficial intestinal bacteria, mainly Lactobacillus and Bifidobacterium, while reducing the number of harmful coliforms and decreasing mortality in birds (Mei et al., Citation2010). Differences in the bioavailability and effects of organic forms of copper on the immune system of poultry have prompted the search for new organic copper compounds containing modified chelate complexes with stronger effects on the immune system (Jarosz et al., Citation2018). One of these compounds, used as a feed additive, is copper-glycine chelate. The effect of copper-glycine chelates and sulphates on immune mechanisms in poultry is not yet fully understood. The high percentage of CD8+ T cells and high concentrations of IL-2 and ceruloplasmin in birds receiving copper-glycine chelate, reported by Jarosz et al. (Citation2018), suggests progressive development of an acute phase response to excessive copper intake. Moreover, activation of the immune system through a pro-inflammatory Th1 cytokine profile leads to damage to cells and tissues, mainly enterocytes, as a result of developing focal inflammation (Jarosz et al., Citation2018). Local gastrointestinal inflammation induced by exogenous and endogenous factors, including feed additives containing elements, is usually accompanied by a systemic reaction known as the acute phase response (APR) (Murata et al., Citation2004). The proteins synthesized during this response, known as acute phase proteins (APP), play a crucial role in defence mechanisms, including killing infectious microbes, repair of tissue damage, and restoration health (Cray et al., Citation2009; Murata et al., Citation2004; O'Reilly et al., Citation2018). Inflammatory processes and the nature of the immune response are also significantly influenced by prostanoids, such as prostacyclin (PGI) and thromboxanes (TX) (Dennis et al., Citation2003). These compounds act by modulating the tissue response and/or by directly regulating the activity of immune cells (Goetzl et al., Citation1995; Tilley et al., Citation2001). Apart from their pro-inflammatory effect, thromboxanes can stimulate certain functions of lymphocytes and macrophages, which translates to a direct immunomodulatory effect (Tilley et al., Citation2001; Dennis et al., Citation2003). Similarly, prostacyclin (PGI2), by modulating the function of dendritic cells, macrophages, monocytes, endothelial cells and eosinophils, exerts an anti-inflammatory or immunosuppressive effect, reducing synthesis of Th1 and Th2 cytokines (Dorris & Peebles, Citation2012).
Determination of concentrations of APPs, cytokines and prostanoids, therefore, enables early detection of disturbances in homeostasis and identification of inflammation and provides the basis for rapid intervention to restore homeostasis. Phytase occurs widely throughout nature in certain plant-sourced feed ingredients and in wheat, rye, barley and their by-products in particular (Godoy et al., Citation2005). Studies conducted in the last century prove that phytase supplementation improves nitrogen retention in broiler chickens and also improves digestibility of nitrogen and amino acids. Phytase also increases the utilization of methionine, lysine, valine, isoleucine and total amino acids in broiler diets (Biehl & Baker, Citation1997). However, information on the effect of combining phytase and copper on pro-inflammatory cytokines and acute phase proteins in poultry has not been reviewed. The objective of this paper is to study the effects of combining phytase and copper in the inorganic and organic form on concentrations of acute phase proteins CRP, SAA, α-AGP, Hp and TRF; cytokines IL-2, TNF-α IL-6, IL-4 and IL-10; thromboxane A2 (TXA2) and B2 (TXB2); and prostacyclin (PGI2) in the peripheral blood and liver of poultry and serum, liver, muscle and intestine copper concentration of, and to discuss factors that influence the combination effects. Also, areas that need further research for optimising the use of a combination of phytase and copper in poultry diets are suggested.
Materials and methods
Experimental animals
The experiment was conducted at the Small Animals Teaching and Research Station of the University of Life Sciences in Lublin, Poland. Consent for all research procedures was obtained from the Local Ethics Committee for Animal Testing at the University of Life Sciences in Lublin, Poland (approval no. 37/2011 of May 17, 2011).
A total of 900 1-day-old Ross 308 male chickens were used in experiment. There were 6 dietary treatments, and each treatment had 5 replicates with 30 birds per replicate pen. The dietary treatments were as follows: a basal diet (control group – group I) except copper; basal diet + phytase as a feed additive (group II); basal diet + copper in inorganic form (CuSO4) (group III); basal diet + copper in inorganic form and a phytase supplement (CuSO4 + P) (group IV); basal diet + copper in organic form, in a complex with glycine (Cu-Gly) (group V); and basal diet + copper in a complex with glycine and a phytase supplement (Cu-Gly + P) (group VI). The composition of the basal diet is presented in . The basal diet (control) was formulated to meet NRC (Citation1994) requirements and dietary recommendations for Ross 308 broiler chickens (Aviagen, Broiler Ross Nutrition Supplement). The nutrient content of the diets was calculated on the basis of the chemical composition of the raw feedstuffs and metabolizable energy value. For groups III-VI, 16 mg kg−1 of copper was added to the feed. The copper requirement of Ross 308 broilers is 16 mg kg−1 feed (Aviagen, Broiler Ross Nutrition Supplement, 2013). According to these recommendations, the content of Cu should be identical at each rearing stage, which was taken into account during the experiment. For groups II, IV and VI, phytase was added to the diets in the amount of 500 phytase activity units (FTU)/kg (RONOZYME® HiPhos, DSM Nutritional Products Sp. z o.o., Mszczonów, Poland). GLYSTAR FORTE chelate (2:1 glycine-metal ratio) manufactured by ARKOP Sp. z.o.o, Bukowno, Poland was added to the diets of groups V and VI.
Table 1. Raw material composition (%) and nutritional value of experimental mixtures.
The chickens had unlimited access to feed and water. They received compound feeds appropriate for each rearing period: starter, S (days 1–21), grower, G (days 22–35), and finisher, F (days 36–42). The starter feed was provided to the chickens in crumble form, and the grower and finisher feeds were pelleted. No coccidiostats or antibiotics were used in the experiment.
The rearing period was 42 days. On the first day of the experiment, when the chicks were one day old, 10 chicks from each group and each replicate were sacrificed by decapitation for the sampling of blood and liver tissue. The remaining chicks were used in the subsequent stages of the experiment. On day 20, 10 chicks from each group and each replicate were sacrificed for the sampling of blood and liver tissue. The remaining chicks continued to receive phytase and copper additives up to day 42 of the experiment. On day 42, the remaining 10 birds from all groups were sacrificed for the sampling of blood and liver tissue.
The experimental birds were housed in pens on wood shavings in a room with controlled temperature and humidity. The pens were equipped with feeding lines and nipple drinkers. The lighting regime was adjusted to the age and diurnal rhythm of the birds. The light intensity was 30–40 lux up to day 7 and 20–30 lux from day 7. Three days before the chickens were placed in the cages, the floor was heated to 29°C and the air to 33°C. The temperature was maintained at 31–33°C up to day 7 and then gradually reduced by 2°C a week until reaching 22–23°C. Relative humidity throughout the experiment was 60% ±10%. The concentration of gases was as follows: ammonia <10 ppm and carbon dioxide <3000 ppm.
Clinical signs and growth performance in the animals
Throughout the experiment, the birds were under clinical observation, with special attention paid to the activity of the birds, their appetite, respiratory symptoms, and the occurrence of digestive disorders in the form of diarrhoea. The health status of the birds was evaluated by determining clinical parameters, anatomopathological changes in dead birds, and the mortality rate (). During the experiment, all chicks were weighed and their initial and final weights were calculated.
Table 2 Evaluation of health and production parameters.
Chemical analysis of feed
Feed samples (n = 3) were analysed for the content of crude protein (procedure 984.13; AOAC, Citation2006) and crude fat (procedure 945.16; AOAC, Citation2006). Crude fibre was determined according to procedure 978.10 (AOAC, Citation2006) using the Ankom 220 Fibre Analyzer (Ankom Technology, Macedon, NY, USA). Cu content in the diets, the following incineration in a muffle furnace at 550°C, with hydrogen peroxide as an oxidizer, was determined by FAAS in a Unicam 939 AA Spectrometer, using Merck standards (Germany), as described by Winiarska-Mieczan and Kwiecień (Citation2015). Total P content was determined by colorimetry according to PN-76/R-64781 in a Helios α-Unicam spectrometer. Content of phytate phosphorus (at a wavelength of λ = 500 nm) was determined using Wade reagent (0.027% FeCl2, 0.254% sulfosalicylic acid) () (Thies, Citation1991).
Table 3. Validation parameters used in the Cu assay.
Blood samples
The material for analysis comprised 2 ml samples of peripheral blood from the wing vein and from one-day-old chicks sacrificed by decapitation. The blood samples were collected in sterile vacuum tubes containing a clot activator and serum separator (Vacuette, Medlab Products, Raszyn, Poland). Blood samples were collected before the start of the study (day 0), on day 20 of rearing, and on day 42, after rearing was completed. At each stage of the experiment, 10 blood samples were collected from each group. The samples were transported to the laboratory at +4°C to +8°C within 1 h. Serum was obtained by centrifuging the blood at room temperature (20–22°C) for 15 min at 4000g. The serum was apportioned and stored at −80°C until analysis.
Assay of CRP, Hp, α-1-AGP, SAA, and TRF in chicken serum
Serum levels of acute phase proteins were determined using ELISA kits specific for chicken CRP (C-reactive protein), Hp (haptoglobin), α-1-AGP (alpha-1-acid glycoprotein), SAA (serum amyloid A) (Biorbyt Ltd, Cambridge, United Kingdom), and TRF (transferrin) (Wuhan Fine Biotech Co., Ltd., East Lake High-tech Development District, Wuhan, Hubei Province, China). All procedures were carried out as recommended by the manufacturer.
Assay of IL-2, IL-6, TNF-α, IL-4, and IL-10 in chicken serum
Serum levels of interleukins were determined using ELISA kits specific for chicken interleukin 2 (IL-2), interleukin 6 (IL-6), tumour necrosis factor α (TNF-α), interleukin 4 (IL-4), and interleukin 10 (IL-10) (Abbexa Ltd, Cambridge, United Kingdom). All procedures were carried out as recommended by the manufacturer.
Assay of TXA2, TXB2, and PGI2 in chicken serum
Serum levels of prostanoids were determined on days 20 and 42 from all birds of all groups using ELISA kits specific for chicken TXA2 (thromboxane A2), TXB2 (thromboxane B2), and PGI2 (prostacyclin) (Wuhan Fine Biotech Co., Ltd., East Lake High-tech Development District, Wuhan, Hubei Province, China). All procedures were carried out as recommended by the manufacturer.
Assay of acute phase proteins CRP, Hp, α-1-AGP, SAA, and TRF, cytokines IL-2, IL-6, TNF-α, ILl-4, and IL-10, and prostanoids TXA2, TXB2, and PGI2 in liver homogenates
Birds were sacrificed on days 1, 20, and 42 of the experiment. The livers were immediately excised, rinsed of blood, and homogenized using a mechanical homogenizer in PBS that contained 0.05% sodium azide and 0.5% Triton 100× (pH 7.4). Liver samples were then sonicated for 10 min. All solid particles were removed by centrifugation of the homogenates at 12,000g for 10 min. Dichloromethane (0.4 ml) was added to 1 ml of supernatant to eliminate all lipid contaminants. This process was followed by a second centrifugation for 10 min at 12,000g, after which the supernatant was removed and immediately assayed.
The CRP, Hp, α-1-AGP, SAA, TRF, IL-2, IL-6, TNF-α, IL-4 and IL-10 ELISA kits were obtained from Abbexa Ltd, Biorbyt Ltd and Wuhan Fine Biotech Co., Ltd. (Abbexa Ltd, Cambridge, United Kingdom; Biorbyt Ltd, Cambridge, United Kingdom; East Lake High-tech Development District, Wuhan, Hubei Province, China). The assays were performed according to the manufacturer’s specifications. Levels of acute phase proteins and cytokines were determined on days 0, 20, and 42 from all birds of all groups. The APP and cytokine content was expressed as ng/mL total protein.
The TXA2, TXB2, and PGI2 ELISA kits were obtained from Wuhan Fine Biotech Co., Ltd. (East Lake High-tech Development District, Wuhan, Hubei Province, China). The assays were performed according to the manufacturer’s specifications. Prostanoid levels were determined on days 20 and 42 from all birds of all groups. Prostanoid content was expressed as ng/mL total protein.
Determination of the copper concentration in serum and tissues by flame atomic absorption spectrometry (FAAS)
The Cu concentration was determined on day 42 of the experiment in samples of the liver, intestines, muscles, and serum of the birds in the control (I) and experimental (II–VI) groups. Samples weighing 0.5 g were weighed out to within 1 mg and subjected to wet mineralization with concentrated nitric acid (HNO3) in a closed-loop microwave system using a CEM Mars Xpress digestion oven (USA). The copper concentration was determined by atomic absorption spectrometry using a Varian SpektrAA 280FS spectrometer with an SPS-3 autosampler and SIPS diluter (Australia), with an air-acetylene flame. Due to the use of a diluter, only a stock solution with a concentration of 20 mg/dm3 was prepared for the calibration curve. The stock solution was aspirated and diluted by the device, while the test samples were placed in the autosampler. Validation parameters were estimated for the determinations ().
Statistical analysis
Statistical analysis of the results was performed using Statistica 13.2 PL software (StatSoft, Krakow, Poland). The data gradient was analysed by the Shapiro–Wilk test, and the results were expressed as arithmetic means and standard deviations. The statistical significance (P < .05) of differences in the immune response between the control sample (I) and experimental samples (II–VI) on each test day (0, 20, and 42 days) was analysed by Student’s t-test for independent samples. The effects of different variants of dietary supplementation on the immune response over time were compared by one-way analysis of variance (ANOVA) and Tukey’s post hoc test for P < .05. The grouping variable was the time of analysis. The results were presented in graphic form (), where the same letters indicate a lack of significant differences. The variables (experimental samples) were designated as follows: I – control sample, II–VI – experimental samples.
Figure 1. One-way ANOVA and subsequent Tukey’s test for acute phase proteins (APPs) concentration in chicken serum (a, c, and e), and liver tissue (b, d, and f). Statistical differences (P ≤ .05) are marked with different letters. SAA – serum amyloid A, α-1-AGP – alpha-1-acid glycoprotein, TRF – transferrin. I – control group, II – VI – experimantal groups.
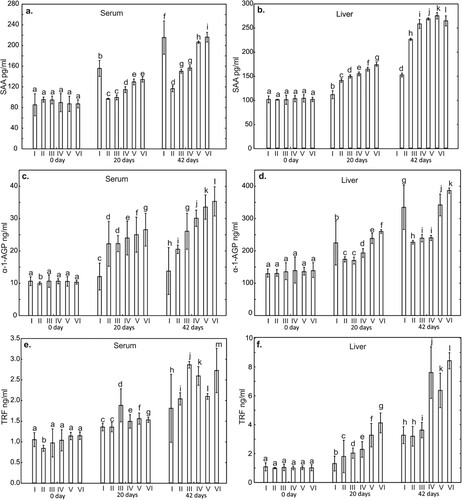
Figure 2. One-way ANOVA and subsequent Tukey’s test for acute phase proteins (APPs) concentration in chicken serum (a and c), and liver tissue (b and d). Statistical differences (P ≤ .05) are marked with different letters. CRP – C-reactive protein, Hp – haptoglobin. I – control group, II–VI – experimantal groups.
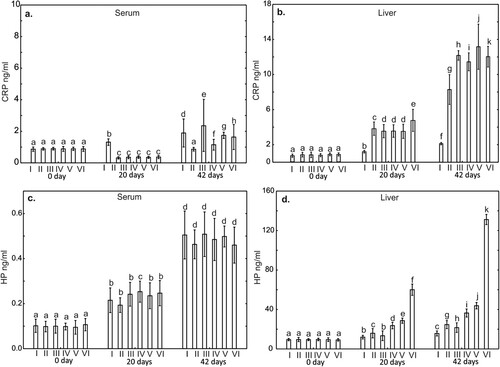
Figure 3. One-way ANOVA and subsequent Tukey’s test for interleukin concentration in chicken serum (a, c, and e), and liver tissue (b, d, and f). Statistical differences (P ≤ .05) are marked with different letters. Il-2 – interleukin 2, TNF-α – tumor necrosis factor alpha, Il-6 – interleukin 6. I – control group, II–VI – experimental groups
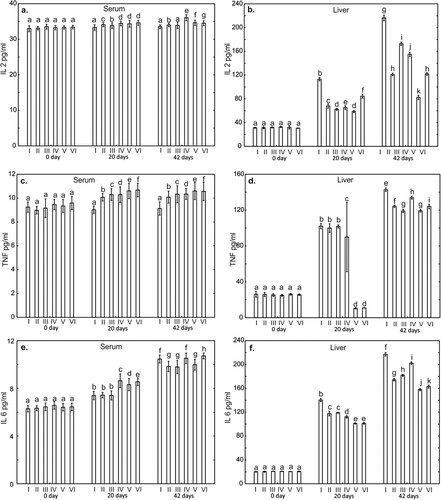
Figure 4. One-way ANOVA and subsequent Tukey’s test for interleukin concentration in chicken serum (a and c), and liver tissue (b and d). Statistical differences (P ≤ .05) are marked with different letters. Il-4 – interleukin 4, Il-10 – interleukin 10. I – control group, II–VI – experimantal groups.
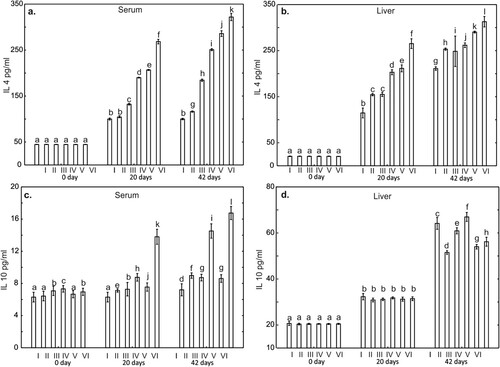
Figure 5. One-way ANOVA and subsequent Tukey’s test for prostanoids concentration in chicken serum (a, c, and e), and liver tissue (b, d, and f). Statistical differences (P ≤ .05) are marked with different letters. TBX B2 – thromboxane B2, TBX A2 – thromboxane A2, Prostacycline (PGI2). I – control group, II–VI – experimental groups.
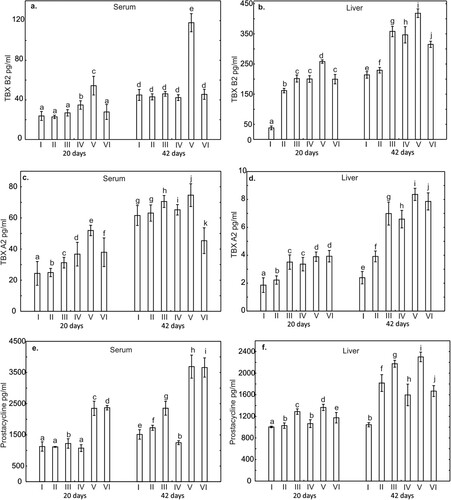
Figure 6. One-way ANOVA and subsequent Tukey’s test for copper concentration in liver tissues (a), serum (b), intestines (c), and muscle tissues (d) in chicken. Statistical differences (P ≤ .05) are marked with different letters. I – control group, II–VI – experimental groups.
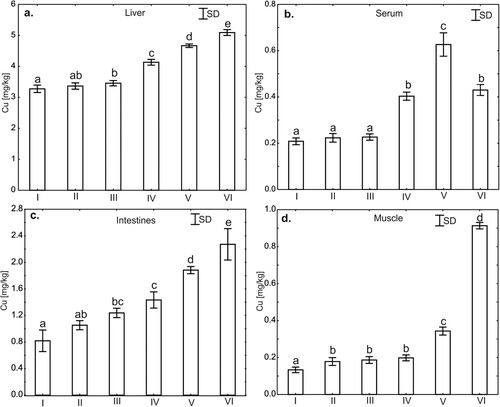
The experimental data on growth performance were analysed with the general linear model (GLM) ANOVA procedure in Statistica 10.0 PL (StatSoft, Krakow, Poland). Statistically significant effects were further analysed, and means were compared using Tukey’s honestly significant difference (HSD) multiple comparison procedure. Statistical significance was determined at P < .05.
Results
Health and production parameters
A statistically significant increase in body weight gain between the experimental groups and the control group was observed in chickens in the groups supplemented with CuSO4 + P, Cu-Gly and Cu-Gly + P. During the experiment, the highest mortality of chickens was observed in the control group (14 birds, which makes up 9.33%). Chickens in the groups supplemented with basal diet + copper in inorganic form and basal diet + copper in organic form, in a complex with glycine did not show gastrointestinal symptoms, while in the remaining research groups and the control group diarrhoea and remitting were observed in chickens. Respiratory symptoms (cough, sneezing, conjunctivitis) were observed in some chickens in all experimental groups and in the control group during the experiment. Among the anatomopathological changes in dead birds, the following were observed: intestinal hyperemia, petechiae in the mucosa of the small intestine, catarrhal enteritis. Detailed data is presented in .
The levels of CRP, Hp, α-1-AGP, SAA, TRF in chicken serum and liver homogenates
The SAA, CRP and HP levels in serum and liver, on day 0 of the experiment in all experimental groups did not differ statistically from the result obtained in the control group. No differences were also observed for liver α-1-AGP and TRF levels in all experimental groups on day 0 compared to the result obtained in the control group. Serum α-1-AGP and TRF levels in the phytase supplemented group on day 0 were statistically significantly lower (P < .05) compared to the control group (–).
The level of CRP in the liver on day 20 of the study in all experimental groups was statistically significantly higher (P < .001), compared to the control group. In contrast, serum CRP levels on day 20 in all supplemented groups were statistically significantly lower compared to the control group. On day 42 of the study, statistically, significantly higher CRP levels were observed only in the liver in all supplemented groups ().
The serum α-1-AGP levels on day 20 of the study in all experimental groups were statistically significantly higher compared to the control group. However, a higher concentration of α-1-AGP in liver samples on day 20 of the study was observed only in the groups supplemented with Cu-Gly and Cu-Gly + P compared to the control group. On day 42 of the study, a statistically significantly higher serum α-1-AGP concentration was observed in all supplemented groups compared to the control group. However, in liver samples only in the groups supplemented with Cu-Gly and Cu-Gly + P the level of α-1-AGP was higher compared to the control group. Both in serum and liver, the level of this protein on day 42 of the study in all groups except for the group supplemented with P, was statistically significantly higher compared to days 0 and 20 ().
A statistically significant lower SAA serum levels was observed in all experimental groups on day 20 compared to the control group. In contrast, SAA levels in liver samples were statistically significantly higher in all experimental groups compared to the control group on both days 20 and 42. Both in serum and liver, the level of this protein on day 42 of the study in all groups was statistically significantly higher compared to days 0 and 20. Higher TRF levels compared to the control group on day 20 of the study were also observed in the liver in all supplemented groups. On day 42, a statistically significantly higher (P < .001), concentration of TRF compared to the control group was observed in the liver in all groups except the groups supplemented with P. Furthermore, both serum and liver levels of this protein at day 42 of the study in all groups were statistically significantly higher compared to days 0 and 20 ().
The serum Hp level on the 20th day of study in all experimental groups did not differ statistically from the control group. However, compared to the control group, a statistically significant increase in Hp levels in the liver was observed in the groups supplemented with P, CuSO4 + P, Cu-Gly and Cu-Gly + P. No statistically significant differences in serum Hp levels were observed in all supplemented groups on day 42 compared to the control group. In contrast, a statistically significant higher level of Hp in the liver compared to the control group was observed in all supplemented groups. An increase in the level of this protein in the serum and liver were also observed in all experimental groups and in the control group on day 42 compared to days 0 and 20, ().
The levels of IL-2, IL-6, TNF-α, IL-4, IL-10 in chicken serum and liver homogenates
There were no statistically significant differences on day 0 of the study in serum and liver concentrations of IL2, IL4, TNF-α and IL6 compared to the control groups. In contrast, the concentrations of IL10 in the serum on day 0 in the groups supplemented with CuSO4, CuSO4+P and Cu-Gly + P were statistically significantly higher (p < .05) compared to the result obtained in the control group. The level of IL10 in the liver on days 0 and 20 of the study did not differ statistically compared to the control group ().
On days 20 and 42 of the study, statistically significantly lower (P < .001) levels of IL2 and IL6 in the liver were observed in all supplemented groups compared to the control group. Also, TNF-α levels on day 20 in the liver in the groups supplemented Cu-Gly and Cu-Gly + P, were statistically significantly lower compared to the control group. On day 42 of the study, the level of TNF-α in the liver was statistically significantly lower in all supplemented groups compared to the control group. In contrast, serum TNF-α levels on day 20 and 42 of the study were statistically higher compared to the control group in all supplemented groups. Serum and liver levels of IL4 on days 20 and 42 of the study were statistically significantly higher compared to the control groups. A statistically significant increase in this cytokine was also observed in serum and liver on day 42 compared to days 0 and 20 (). Serum levels of IL10 on days 20 and 42 were statistically significantly higher in all supplemented groups compared to the control group. On day 42 of the study, liver IL10 levels in the groups supplemented with P, CuSO4, Cu-Gly and Cu-Gly + P were statistically lower compared to the control group. Serum and liver levels of this cytokine at day 42 of the study were higher in all groups compared to days 0 and 20, ().
The levels of prostanoids: TXA2, TXB2, PGI2 in chicken serum and liver homogenates
A statistically significantly higher level (P < .05) of TBX A2 in serum compared to the control group, measured on the 20th day of the study, was demonstrated in all supplemented groups. The highest TBX A2 level on day 20 in serum was observed in the group supplemented with Cu-Gly. On day 42, serum TBX A2 levels were significantly higher in the groups supplemented with CuSO4, Cu-SO4 + P and Cu-Gly when compared to the control group (). Furthermore, the level of this prostanoid in the liver on day 20 in all supplemented groups was also statistically significantly higher (P < .001) compared to the control group. Similarly, on day 42 of the study, TBX A2 levels in the liver were statistically significantly higher in all supplemented groups compared to the control group (Figure B1). In addition, on day 42 of the study, TBX A2 levels in both serum and liver were higher in all groups compared to levels of this prostanoid on day 20 in the same groups ().
A statistically significantly higher level (P < .05) of TBX B2 in serum compared to the control group, measured on day 20 of the study, was demonstrated in groups supplemented with CuSO4+P and Cu-Gly. In contrast, on day 42 of the study, a statistically significantly higher level of TBX B2 in serum compared to the control group was observed only in the group supplemented with Cu-Gly. The level of this prostanoid in the liver on day 20 in all supplemented groups was statistically significantly higher compared to the control group. Similarly, on day 42 of the study, TBX B2 liver levels in all supplemented groups were statistically significantly higher compared to the control group. Furthermore, on day 42 of the study, both plasma and liver TBX B2 levels were higher in all groups compared to the level of this prostanoid on study day 20 in the same groups ().
On day 20, serum prostacycline levels were statistically significantly higher compared to the control group only in groups supplemented with the CuSO4, Cu-Gly and Cu-Gly + P. In contrast, the level of this prostanoid measured on day 20 in the liver was statistically significantly higher compared to the control group in all supplemented groups. On day 42 of the study, compared to the control group a statistically significant higher serum prostacycline level was observed in all groups except the group supplemented with CuSO4 + P. In contrast, liver prostacyclin levels on day 42 of the study in all supplemented groups were statistically significantly higher compared to the control group. Furthermore, on day 42 of the study, both serum and liver levels of prostacycline were higher in all groups compared to levels of this prostanoid on day 20 of the study in the same groups ().
The level of Cu in serum, liver, intestinal and muscle tissues
Serum Cu levels in the groups supplemented with F and CuSO4 do not differ statistically significantly from the control group and are statistically significantly lower than Cu levels in the other supplemented groups. No significant differences in the level of Cu were also observed between the groups supplemented with CuSO4 + P and Cu-Gly + P. The highest serum Cu level was observed in the group supplemented with Cu-Gly ().
The level of Cu in liver tissues compared to the control group does not differ in the group supplemented with phytase (P), but is statistically significantly higher in all other supplemented groups. A similar relationship was observed for the group supplemented with P, the level of Cu in the liver in this group does not differ statistically from the control group and CuSO4, and is statistically significantly lower compared to other supplemented groups. The highest levels of Cu in liver tissues were observed in the groups supplemented with: CuSO4 + P, Cu-Gly and Cu-Gly + P ().
The level of Cu in intestinal tissues compared to the control group does not differ in the group supplemented with phytase (P), but is statistically significantly higher in all other supplemented groups. A similar relationship was observed for the group supplemented with P, the level of Cu in the intestinal tissues in this group does not differ statistically from the control group and CuSO4, and is statistically significantly lower compared to other supplemented groups. Moreover, Cu level in the intestinal tissues in the group supplemented with CuSO4 does not differ statistically from the group supplemented P and CuSO4 + P and is statistically significantly lower compared to other supplemented groups ().
The levels of Cu in muscle tissues in all supplemented groups were statistically significantly higher compared to the control group. However, in the groups supplemented with: P, CuSO4 and CuSO4 + P, the levels of Cu in muscle tissues were at a similar level and no statistically significant differences were observed between these groups. A statistically significantly higher level of Cu in muscle tissues were observed in the groups supplemented with Cu-Gly and Cu-Gly + P compared to the other groups. The highest level of Cu in muscle tissues was observed in the group supplemented with Cu-Gly + P ().
Discussion
Copper is an element with important functions in many life processes in humans and animals, including birds (Jarosz et al., Citation2018; Pesti & Bakalli, Citation1996). Copper also strongly affects the immune system, protecting the body against free radicals and increasing resistance to infection (Chatterjee et al., Citation2009; Das et al., Citation2014; Jarosz et al., Citation2018). An appropriate copper concentration in the body is necessary for normal organ growth and development and ensures the physiological functioning of the immune system, especially in young individuals (Bauerly et al., Citation2005; Fry et al., Citation2012; Liao et al., Citation2017). Studies in animals have shown impairment of humoral and cellular immune mechanisms in conditions of copper deficiency (Gengelbach et al., Citation1994; Hopkins & Failla, Citation1995; Sharma et al., Citation2008), leading to disturbances in the maturation and proliferation of leukocytes and to impairment of neutrophil function. This results in an overall reduction in immunity and thus in the increased incidence of infection and higher mortality (Stabel et al., Citation1993). One of the common strategies for correcting copper deficiencies in humans and animals is rationalization of the diet and the use of copper formulations as diet supplements (Nguyen et al., Citation2020). In poultry, dietary modifications mainly involve the use of compound feed supplemented with organic copper compounds, usually in the form of a glycine chelate (El-Ghalid et al., Citation2019).
The literature indicates that supplying copper to the body in the form of chelates increases its bioavailability and the efficiency with which it is absorbed and accumulated in tissues (Baker et al., Citation1991; Das et al., Citation2014; Wapnir, Citation1998). These data are confirmed by the results of the present study, in which the highest serum Cu concentration was noted in the group of chickens receiving a copper-glycine chelate. The results are consistent with those previously published by Liao et al. (Citation2018), who showed that supplementation of pig feed with copper in complexes with lysine increased its concentration in the plasma. Similarly, in the present study the highest concentration of copper in the muscle tissue, liver and intestines were noted in the group of birds whose feed contained a copper-glycine chelate with phytase. These results are consistent with those published by Cromwell et al. (Citation1989) and Liao et al. (Citation2017), who reported an increase in copper concentrations in the liver and kidneys of piglets whose feed was supplemented with an organic form of copper. Paik et al. (Citation2000, Citation2001) obtained similar results in research on poultry, in which feed supplementation with copper-methionine chelates increased the concentration of copper in the muscle tissue. The growth and development of muscle tissue resulting from the use of copper chelates as feed additives are linked in part to the effect of copper on the expression of growth hormone genes in the pituitary gland, secretion of neuropeptides in the hypothalamus (Zhou et al., Citation1994), and stimulation of lipid metabolism during growth processes. This hypothesis is indirectly confirmed by the results of our previous research (Jarosz et al., Citation2018), in which weight gains were higher in chickens receiving copper-glycine chelates than in the group of birds whose feed was supplemented with copper sulphates. The copper concentration in various tissues resulting from its administration with feed appears to some extent to affect the metabolic processes that the supplements undergo in the body. This is demonstrated by Paik et al. (Citation2000, Citation2001) who noted higher Cu concentrations in the liver tissue following the use of copper sulphate in the diet of poultry in comparison to chelates. It should be stressed that there has been no extensive research on the effect of copper-glycine chelates on metabolic processes associated with the distribution of copper in the body. However, glycine is known to have the lowest molecular weight of all amino acids, which is conducive to the stability of chelate complexes and counteracts adverse chemical reactions that limit the absorption of ions of elements complexed with glycine in the stomach and intestines (Kulkarni et al., Citation2012). This is confirmed by the results of the present study, which showed a high Cu concentration in the tissues of the intestines, liver and muscles of chickens following the use of a copper-glycine chelate with phytase in the diet. Such accumulation of copper is likely to affect the cells of the tissues in which it accumulates, leading to metabolic changes in these cells and thus increasing the synthesis of immunoregulatory proteins in the liver.
The studies showed that the use of copper in the form of glycine chelate in poultry nutrition resulted in an improvement in production parameters and an increase in the slaughter body weight on the 42nd day of the study compared to the control group (). The obtained results are consistent with the studies previously published by Lim and Paik (Citation2006) and Paik (Citation2001), which showed that the addition of copper in the form of methionine chelate significantly influences the production effects of broilers by increasing the BWG. Similar results were obtained by Hong et al. (Citation2002), who showed that the addition of Cu in the form of Met-Cu or Met-Cu-Zn improves broiler productivity by increasing BWG and FCR (feed conversion rate). The increase in BWG in broilers fed with copper-glycine chelate was explained by the greater bioavailability of these compounds, as previously confirmed by Aoyagi and Baker (Citation1993). The influence of copper-glycine chelates on the synthesis of cytokines, acute phase proteins and prostanoids indicates their modulating effect on the immune system. It is worth emphasizing that glycine in the form of a chelate is characterized by a low molecular weight, which promotes the stability of chelated compounds and prevents unwanted chemical reactions of elements in the stomach and intestines (Kulkarni et al., Citation2011). The literature shows that feed supplementation with Cu chelates stimulates the growth of chickens also through changes in the microflora of the gastrointestinal tract (Arias & Koutsos, Citation2006; Pang et al., Citation2009). The positive effect of these changes is the reduction of ILE lymphocyte infiltration into the stroma of the intestinal cells and better absorption of nutrients. These data are confirmed by our results, in which in the groups of birds receiving Cu in combination with glycine a lower mortality rate was found, as well as no anatomopathological changes indicating an ongoing disease process. Of particular importance in terms of health is the effect of copper on the functioning of the immune system (Das et al., Citation2014; Fry et al., Citation2012; Jarosz et al., Citation2018). Studies in humans have shown that the use of copper-glycine chelates in the diet is associated with stimulation of the Th1 response (Chatterjee et al., Citation2009), which is linked to secretion of pro-inflammatory cytokines IL-2, IFN-γ and TNF-α promoting cellular immune mechanisms. One of the most important of these cytokines is IL-2, which mediates communication between various immune cells and takes part in regulation of the body’s response to infection (Rochman et al., Citation2009). Our study showed a significant increase in the serum concentration of IL-2 on days 20 and 42 of the experiment in the groups of chickens receiving copper sulphate and copper-glycine chelate (IV and V) relative to the control. These results indicate activation of lymphocytes involved in the cellular response and activation of proliferative cytokines and not inflammatory cytokines. The increased IL-2 concentration in conjunction with the high concentration of immunoregulatory α-AGP in both of these groups suggests that copper-glycine chelates also induce the production of regulatory lymphocytes taking part in the suppression of the immune response. In this context it should be noted that a high copper concentration in the body is essential for the production of IL-2 by activated lymphocytes and also, through its effect on T cells, plays an important role in supporting cellular and humoral immunity (Percival, Citation1998; Tapiero et al., Citation2003). The results of the present study in combination with those previously published by Jarosz et al. (Citation2018), which showed an increased percentage of cells with CD3+CD4+, CD3+CD8+ and CD25+ phenotypes in chickens receiving feed supplemented with glycine chelates, confirm that these copper compounds stimulate cellular mechanisms by stimulating Th1 lymphocytes. Contrasting results were obtained for the IL-2 concentration in the liver. The high concentration of this cytokine in combination with the highest concentrations of IL-6 and TNF-α in the control group on day 42 of the study may indicate a developing inflammatory process. An additional confirmation of this phenomenon may be the increasing synthesis of anti-inflammatory IL-10 in the liver in this group. It is interesting that a similar pattern was shown in groups III and IV, in which the birds received copper sulphate with and without the addition of phytase. It seems likely that the high concentration of IL-2 in conjunction with the high concentrations of IL-6 and TNF-α in these groups indicates developing inflammation in the intestines due to the irritant effect of the sulphates. The results of the study indicate, however, that these reactions are suppressed by immunosuppressant IL-10, whose concentration in these groups was significantly higher than in the other experimental groups. The increase in IL-2 in the experimental groups together with the concentrations of acute phase proteins SAA and α-AGP in the serum and liver, therefore, suggest that this cytokine has an immunoregulatory function and is involved in suppressing the immune response.
IL-6, which is also a pro-inflammatory cytokine, is released by circulating immune cells, fibroblasts and endothelial cells in response to inflammation and initiates an acute phase response in the hepatocytes and secretion of the proteins CRP and SAA (Chiba et al., Citation2010; Schneider et al., Citation2001). Our study (Jarosz et al., Citation2018) showed an increase in the serum concentration of this cytokine on days 20 and 42 of the study in the group of birds receiving a copper-glycine chelate, which suggests activation of a pro-inflammatory Th1 response. The high IL-6 concentration together with the high concentration of IL-4 in the serum of birds receiving a copper-glycine chelate suggests that the use of this supplement in the diet of poultry directs differentiation of T cells towards the production of Th2 cytokines. The simultaneous high concentration of IL-10 in this group indicates that it has an anti-inflammatory effect and modulates the immune response towards a balance between the synthesis of Th1 and Th2 cytokines. It should be noted that the present study showed a statistically significantly higher concentration of IL-10 in the liver of birds in the control group, in which it limited developing inflammation.
Analysis of the concentration of pro-inflammatory TNF-α in the serum showed that it increased on days 20 and 42 of the experiment in the groups of birds receiving a copper-glycine chelate, which indicates the promotion of cellular immune mechanisms through enhancement of the activity of Th1 lymphocytes. Our previously published results (Jarosz et al., Citation2018), in which an increase in CD4+ and CD8+ lymphocytes were shown in an analogous group of birds, together with the results of the present study, that is, high concentrations of IL-10, SAA and α-AGP, are indicative of Th1 lymphocyte differentiation and the formation of a subpopulation of Treg cells maintaining Th1/Th2 homeostasis. As in the case of IL-6 and IL-2, the liver concentration of TNF-α on days 20 and 42 of the experiment was significantly higher in the control group than in the experimental groups. This is probably due to the stimulation of systemic immune mechanisms in response to environmental antigens that can lead to infections in this period. TNF-α may induce the release of IL-6 from macrophages in response to infection (Chang et al., Citation2010). This is confirmed by the results of our study, in which the highest IL-6 concentration was noted in the control group on days 20 and 42 of the experiment. The increase in TNF-α and IL-6 in the serum and liver of birds in the experimental groups may also be due to the effect of copper itself, which exerts a pro-inflammatory effect by stimulating the synthesis of these cytokines. This has been confirmed by Cohen et al. (Citation2013). It is puzzling, however, that the liver TNF-α concentrations were very low in groups V and VI on day 20 of the experiment, which may indicate a lack of immune system reactivity during this period.
Anti-inflammatory cytokines, which include IL-4 and IL-10, are antagonistic towards pro-inflammatory cytokines. IL-4 stimulates the growth and differentiation of B lymphocytes (Heeb et al., Citation2020; Rothwell et al., Citation2004). The high concentration of this cytokine noted on days 20 and 42 of the experiment in the serum and liver tissue of the birds in the experimental groups indicates the promotion of humoral immune mechanisms. Similar observations were made by El-kazaz and Hafez Hafez (Citation2020) and by Wang et al. (Citation2011) in studies on poultry, in which administration of organic copper nanoparticles (Cu-NP) to birds with their feed increased the concentrations of serum IgA, IgY and IgM immunoglobulins, which suggests stimulation of the immune system and a reduced risk of infection. The higher concentrations of IL-4 and IL-10 noted in the experimental groups on day 42 of the study may be due to activation of B cells, either as a direct result of reactions of these cells with the copper contained in the feed or as an indirect effect of cytokines released from macrophages or other phagocytes. The simultaneous increase in the IL-6 concentration in the serum and liver tissue of the birds on days 20 and 42 of the experiment may indicate a stimulatory effect of this cytokine on phagocyte proliferation, correlated with increased synthesis of IL-4 and IL-10, which stimulate antibody production. Similar dependencies were reported in a study by Ognik et al. (Citation2018).
IL-10, which is released by many types of cells, primarily B cells, is of great importance for the functioning of immune processes in the body (Rothwell et al., Citation2004). The high IL-10 concentration in the liver of birds in the experimental groups on day 42 of the experiment in conjunction with the high concentration of pro-inflammatory cytokines IL-6 and TNF-α indicates ongoing inflammatory processes induced by the copper sulphates and chelates added to the feed. The results may also be indicative of an immunomodulatory function of IL-10 in relation to humoral immune mechanisms, protecting against chronic intestinal inflammation. The high IL-10 concentration noted on days 20 and 42 of the study in the serum of birds receiving a copper-glycine chelate with phytase in conjunction with the high IL-4 concentration during this period indicates an intensification of B cell proliferation and antibody production. The high concentration of IL-2 in these groups additionally suggests sensitization of the B cell pool to the effects of IL-10.
The cytokines analysed in the study initiate and modulate the acute phase response in birds, manifested as synthesis of numerous proteins that can exacerbate or mitigate inflammation (Cray et al., Citation2009; O'Reilly et al., Citation2018). Easily absorbed copper, especially in the form of glycine chelates, is redistributed from the bloodstream to other tissues, including the liver, where it may cause an increase in the synthesis of APPs and thus contribute to a disturbance of homeostasis. In most animal species, including poultry, the main APP is serum amyloid A (SAA) (O'Reilly et al., Citation2018; Sevimli et al., Citation2008; Urieli-Shoval et al., Citation2000). In the present study, a high SAA concentration on day 42 of the experiment was noted in the serum and liver tissue of birds receiving a copper-glycine chelate with their feed. It is likely that SAA synthesis in the liver of chickens in this group proceeded normally; the high concentration of this protein in conjunction with the concentrations of other immunoregulatory proteins, such as α-AGP and Th2 cytokines, demonstrates that no inflammatory process is taking place in the body and that SAA is only involved in the maintenance of homeostasis and modulation of the immune response. The highest serum concentration of this protein was shown on days 20 and 42 in the birds in the control group. The results indicate activation of the acute phase response (APR), most likely as a consequence of developing local inflammation in the intestines. This hypothesis is supported by the high concentrations of pro-inflammatory cytokines at this time, that is, TNF-α and IL-2 in the serum and TNF-α, IL-6 and IL-2 in the liver tissue. The increased SAA concentration in the serum and liver tissue of the birds in groups I–VI is the body’s response to colonization of the gastrointestinal tract by saprophytic microbes, which colonize the intestine during ontogenesis and influence the functioning of the GALT system. Literature data indicate that the SAA concentration in the body may be influenced by a variety of stress factors (Alsemgeest et al., Citation1995). This is confirmed by Alsemgeest et al. (Citation1995), who showed that the use of various copper compounds in calves, due to its irritant effects on the intestinal epithelium, disturbs cellular homeostasis, which is manifested as an increase in the SAA concentration.
Among other acute phase proteins present in poultry, α1-acid glycoprotein (AGP), which is synthesized and secreted by hepatocytes, is considered to be of great diagnostic and prognostic importance (Murata et al., Citation2004; O'Reilly et al., Citation2018). AGP has been shown to have immunomodulatory properties and to take part in regulation of the inflammatory response (Hochepied et al., Citation2003). In the present study, the highest concentrations of AGP in the serum and liver tissue were noted on days 20 and 42 of the experiment in the groups of birds receiving a copper-glycine chelate with and without phytase, which suggests that it has anti-inflammatory and immunoregulatory effects. In this respect, AGP may also influence the functions of T cells by inhibiting their proliferation. Chickens receiving copper-glycine chelates with their feed had a higher percentage of CD3+CD4+, CD3+CD8+ and CD25+ T cells, which stimulate cellular immune mechanisms (Jarosz et al., Citation2018). Some of these cells, however, function as regulator lymphocytes, and therefore the previous results in conjunction with the AGP concentration in the liver suggest that this protein will inhibit inflammatory reactions caused by excessive intake of copper in chelated form, thereby maintaining homeostasis. The high AGP concentration in the chickens from the control group, on the other hand, indicates increased synthesis of this protein. This may be linked to the slow development of local inflammation, the destruction of intestinal epithelial cells, and an increase in colonization of the mucosa with pathogenic microbes. However, the AGP concentration did not exceed 400 μg/mL in any group of birds at any time during the experiment, which means that acute bacterial infection can be ruled out in the chickens in the experimental and control groups. It should be borne in mind, however, that the 42-day rearing period may have been too short for the development of clinical forms of the disease, and inflammatory processing causing an increase in AGP in group I may have developed only in the intestines. It is worth noting that AGP protects the body against the harmful effects of TNF-α, which Libert et al. (Citation1991) showed in a study in mice. In the present study, the high concentration of TNF-α in the serum and liver tissue of birds in the control group in combination with a high AGP concentration indicates that these proteins interact to limit the acute phase response, stimulating the cytotoxicity of immunocompetent cells such as macrophages and heterophils, which counteract infections by pathogenic microbes. The same dependency was shown in groups V and VI, in which the birds received a copper-glycine chelate with and without phytase. This shows that AGP protects against the harmful effects of TNF-α, having a immunoregulatory effect.
Inflammation in birds also involves changes in the concentrations of other proteins, such as transferrin (O'Reilly et al., Citation2018). Our study showed a significant increase in the serum transferrin level on day 42 of the study in the group of chickens receiving copper sulphate with and without phytase or a copper-glycine chelate with phytase. An increase in the concentration of this protein on day 42 of the study was also noted in the liver tissue of birds receiving copper sulphate with phytase and copper-glycine chelate with and without phytase. This increase in the concentration of transferrin may be explained by the irritant effect of copper compounds on the intestinal epithelium and the associated local inflammation. On the other hand, transferrin also exhibits immunomodulatory activity, stimulating the activity of heterophils and macrophages and facilitating tissue remodelling and angiogenesis, which may explain its increased synthesis in the liver tissue under the influence of copper compounds (Rath et al., Citation2009; Xie et al., Citation2002). Our previous research (Jarosz et al., Citation2018) showed that feed supplementation with copper-glycine chelates increases the percentage of phagocytic monocytes and heterophils and the proliferative activity of CD4+ T cells. The results of that study in combination with the concentration of transferrin in the serum and liver indicate that highly bioavailable copper-glycine chelates stimulate nonspecific defence mechanisms and enhance immunoregulatory processes. These results are confirmed by Xie et al. (Citation2002), who demonstrated a modulatory role of transferrin towards macrophages and heterophils, as well as bactericidal and anti-inflammatory effects and maintenance of homeostasis. In the group of birds receiving copper sulphate, a significant increase in the serum transferrin concentration was already noted on day 20 of the experiment, and in the case of copper sulphate with phytase, on day 42. In conjunction with the high concentrations of other acute phase proteins and Th1 cytokines, this suggests the progressive development of local inflammatory changes resulting from the irritant effect of copper sulphates.
Haptoglobin (Hp) is another acute phase protein exhibiting strong anti-inflammatory activity, modulating the synthesis of prostaglandins, and inhibiting chemotaxis of granulocytes and phagocytosis (Cerón et al., Citation2005; O'Reilly et al., Citation2018). The increase in Hp in the serum of birds from all groups on day 42 of the experiment may indicate colonization of the digestive tract by conditionally pathogenic bacteria stimulating local immune mechanisms. The results seem to confirm that the use of copper preparations in birds mobilizes immunocompetent cells of the GALT system, mainly heterophils, to the local synthesis of Hp. In our study, the highest Hp concentration in the liver tissue was shown on days 20 and 42 of the experiment in the group of birds receiving the copper-glycine chelate with phytase. These results may be linked to stimulation of protein synthesis by copper, and in conjunction with increased synthesis of prostanoids, they confirm the immunomodulatory effect of copper chelates on non-specific immune mechanisms.
One of the most important APPs serving as early markers of ongoing inflammation is CRP (Eckersall & Bell, Citation2010). In the present study, the highest serum CRP concentration was noted on day 42 of the study in the group of chickens receiving copper sulphate. This may indicate a developing local inflammatory process induced by the irritant effect of the copper compound or excessive stimulation of enterocytes of the intestinal epithelium and stimulation of local immune mechanisms due to copper intake. The highest CRP concentration in the liver tissue was noted on day 42 of the experiment in the group of chickens receiving a copper-glycine chelate, which suggests stimulation of APP synthesis in response to the intake of highly bioavailable copper in chelated form and is indicative of its immunostimulatory effect. It should be noted that in the other experimental groups the CRP concentration in the liver tissue increased significantly relative to the control group on day 42 of the study. These data allow the result to be interpreted as a consequence of natural synthesis of the protein, associated with stimulation of immunological phenomena in response to stimulation of metabolic processes by copper. An increase in CRP in the liver also stimulates the synthesis and release of TNF-α, which makes it possible to monitor developing inflammation (Beaven & Abreu, Citation2004). The present study showed high concentrations of CRP and TNF-α in the liver on day 42 of the study in the group receiving a copper-glycine chelate with or without phytase. These results demonstrate that excessive copper in poultry feed may be responsible for the multiplication of microbes in the gastrointestinal tract, inducing a GALT response, and may also irritate the enterocytes. In both cases, an increase in these parameters may trigger a cascade of synthesis of cytokines and acute phase proteins in the liver as a systemic response, whereas in conjunction with the concentrations of acute phase proteins and cytokines it indicates mechanisms of immunoregulation of the Th1/Th2 response.
Under the influence of acute phase proteins and various cytokines, such as TNF-α, the synthesis of prostaglandins and leukotrienes is increased, especially during the development of inflammation. Prostaglandins, thromboxanes and prostacyclin, which are produced in the cyclooxygenase (COX) pathway of arachidic acid metabolism, influence numerous biological processes (Camacho et al., Citation2008; Dorris & Peebles, Citation2012; Goetzl et al., Citation1995; Narumiya et al., Citation1999; Nataraj et al., Citation2001), including the development of inflammation and the immune response (Tilley et al., Citation2001; Dennis et al., Citation2003). One of the prostanoids exhibiting mainly pro-inflammatory activity is thromboxane A2 (TBX A2). In the present study, the highest concentration of TBX A2 was obtained on days 20 and 42 in the group of chickens receiving a copper-glycine chelate. Similar results were obtained for TBX B2 and prostacyclin, whose concentrations in the serum and liver tissue were highest on days 20 and 42 in the group of birds receiving a copper-glycine chelate. The results of previous research clearly indicate that these prostanoids affect the functions of lymphocytes and macrophages by stimulating their proliferation, thereby exerting an immunomodulatory effect (Nataraj et al., Citation2001; Snyder et al., Citation1982; Dennis et al., Citation2003). Taken as a whole, the results obtained for the concentrations of acute phase proteins α-AGP and SAA and pro- and anti-inflammatory cytokines in the serum and liver tissue indicate that the use of copper-glycine chelates in poultry results in the regulation of the activity of immune cells and cells involved in the inflammatory response. The increase in activation of immune cells observed in the experiment under the influence of rapidly absorbed bioavailable copper supplied to chickens in chelated form modulates and enhances cellular immune mechanisms, which was also confirmed in our previously published research (Jarosz et al., Citation2018). Prostanoids, whose concentration was determined in the present study, also play an important role in the pathogenesis of immunological damage observed in the intestinal epithelium, as in the case of sulphates as feed additives, which act as an irritant. In these conditions prostanoids exert multifaceted effects, leading to vascular constriction, platelet aggregation, stimulation of biosynthesis of extracellular matrix proteins (Bruggeman et al., Citation1991) and changes in their metabolism (Coffman et al., Citation1998), tissue fibrosis and scarring, and stimulation of secretion by pro-inflammatory cells (Dennis et al., Citation2003). Prostacyclin (PGI2), which was assayed in the experiment, influences specific and non-specific parameters of the immune response, exerting anti-inflammatory or immunosuppressive effects. The high concentration of PGI2 in the group of chickens receiving a copper-glycine chelate, in conjunction with the high concentration of IL-10, confirms its anti-inflammatory effect in the body as a consequence of administration of this supplement to chickens. The high percentage of CD4+ cells and B cells observed by Jarosz et al. (Citation2018) in an analogous group of birds, together with the results of the present study, confirms the role of PGI2 in immunoregulation of the Th1/Th2 response. It should also be emphasized that prostacyclin protects cells against cytokine toxicity, in part by reducing activation of nuclear transcription factor NF-κB, preventing induced synthesis of nitric oxide (iNOS) and limiting activation of caspase-3 in cells (Zhou et al., Citation2007). A low prostacyclin concentration in cells may thus significantly affect programmed cell death. The high prostacyclin (PGI2) level in the group of birds given a copper-glycine chelate, accompanied by high concentrations of pro- and anti-inflammatory cytokines, may also be indicative of its protective role in suppressing an intestinal inflammatory reaction as a consequence of intake of highly bioavailable copper.
Conclusions
Supplementation of poultry feed with copper chelates promotes secretion of pro- and anti-inflammatory cytokines involved in enhancing the immune response. Increased synthesis of the immunoregulatory acute phase proteins SAA and α-AGP and of anti-inflammatory and immunoregulatory prostanoids following the administration of copper-glycine chelates in the diet indicates the stimulatory effect of copper on cellular and humoral immune mechanisms and on processes maintaining Th1/Th2 balance. Excessive intake of copper sulphates and copper-glycine chelates in combination with phytase may lead to the development of local inflammation in the intestines, thus increasing susceptibility to infection. It can be concluded from the results of the experiment that copper-glycine chelates exert a beneficial effect on the growth of broiler chickens, particularly on immune system functions through potentiation and regulation of the immune response in birds. Further research is needed to determine the long-term effects of copper-glycine chelates on the development of local defence mechanisms in the gastrointestinal tract (GALT) and their effect on enterocytes.
Disclosure statement
No potential conflict of interest was reported by the author(s).
References
- Akinleye, S. B., Iyayi, E. A., & Afolabi, K. D. (2008). The performance, haematology and carcass traits of broilers as affected by diets supplemented with or without biomin a natural growth promoter. World Journal of Agricultural Sciences, 4(4), 467–470.
- Alsemgeest, S. P., Lambooy, I. E., Wierenga, H. K., Dieleman, S. J., Meerkerk, B., van Ederen, A. M., & Niewold, T. A. (1995). Influence of physical stress on the plasma concentration of serum amyloid-a (SAA) and haptoglobin (HP) in calves. Veterinary Quarterly, 17(1), 9–12. https://doi.org/10.1080/01652176.1995.9694521
- AOAC. (2006). Official methods of analysis of the association of official analytical chemists (18th ed). Association of Official Analytical Chemists.
- Aoyagi, S., & Baker, D. H. (1993). Nutritional evaluation of copper-lysine and zinc-lysine complexes for chicks. Poultry Science, 72(1), 165–171. https://doi.org/10.3382/ps.0720165
- Arias, V. J., & Koutsos, E. A. (2006). Effects of copper source and level on intestinal physiology and growth of broiler chickens. Poultry Science, 85(6), 999–1007. https://doi.org/10.1093/ps/85.6.999
- Attia, Y. A., Abdalah, A. A., Zeweil, H. S., Bovera, F., El-Din, A. T., & Araft, M. A. (2011). Effect of inorganic or organic copper additions on reproductive performance, lipid metabolism and morphology of organs of dual-purpose breeding hens. Archiv fur Geflugelkunde, 75(3), 169–178.
- Baker, D. H., Odle, J., Funk, M. A., & Wieland, T. M. (1991). Research note: Bioavailability of copper in cupric oxide, cuprous oxide, and in a copper-lysine complex. Poultry Science, 70(1), 177–179. https://doi.org/10.3382/ps.0700177
- Bauerly, K. A., Kelleher, S. L., & Lönnerdal, B. (2005). Effects of cop-per supplementation on copper absorption, tissue distribution, and copper transporter expression in an infant rat model. American Journal of Physiology-Gastrointestinal and Liver Physiology, 288(5), G1007–G1014. https://doi.org/10.1152/ajpgi.00210.2004
- Beaven, S. W., & Abreu, M. T. (2004). Biomarkers in inflammatory bowel disease. Current Opinion in Gastroenterology, 20(4), 318–327. https://doi.org/10.1097/00001574-200407000-00004
- Biehl, R. R., & Baker, D. H. (1997). Microbial phytase improves amino acid utilization in young chicks fed diets based on soybean meal but not diets based on peanut meal. Poultry Science, 76, 355–360.
- Bruggeman, L. A., Horigan, E. A., Horikoshi, S., Ray, P. E., & Klotman, P. E. (1991). Thromboxane stimulates synthesis of extracellular matrix proteins in vitro. American Journal of Physiology, 261(3), 488–494. https://doi.org/10.1152/ajprenal.1991.261.3.F488
- Brugger, D., & Windisch, W. M. (2015). Environmental responsibilities of livestock feeding using trace mineral supplements. Animal Nutrition, 1(3), 113–118. https://doi.org/10.1016/j.aninu.2015.08.005
- Camacho, M., Rodríguez, C., Salazar, J., Martínez-González, J., Ribalta, J., Escudero, J. R., Masana, L., & Vila, L. (2008). Retinoic acid induces PGI synthase expression in human endothelial cells. Journal of Lipid Research, 49(8), 1707–1714. https://doi.org/10.1194/jlr.M700559-JLR200
- Cerón, J. J., Eckersall, P. D., & Martínez-Subiela, S. (2005). Acute phase proteins in dogs and cats: Current knowledge and future perspectives. Veterinary Clinical Pathology, 34(2), 85–99. https://doi.org/10.1111/j.1939-165X.2005.tb00019.x
- Chang, C. H., Chung, C. H., Hsu, C. C., Huang, T. Y., & Huang, T. F. (2010). A novel mechanism of cytokine release in phagocytes induced by aggretin, a snake venom C-type lectin protein, through CLEC-2 ligation. Journal of Thrombosis and Haemostasis, 8(11), 2563–2570. https://doi.org/10.1111/j.1538-7836.2010.04045.x
- Chatterjee, S., Mookerjee, A., Mookerjee Basu, J., Chakraborty, P., Ganguly, A., Adhikary, A., Mukhopadhyay, D., Ganguli, S., Banerjee, R., Ashraf, M., Biswas, J., Das, P. K., Sa, G., Chatterjee, M., Das, T., Choudhuri, S. K., & Rich B. E. (2009). A novel copper chelate modulates tumor associated macrophages to promote anti-tumor response of T cells. PLoS ONE, 4(9), e7048. https://doi.org/10.1371/journal.pone.0007048
- Chiba, Y., Shida, K., Nagata, S., Wada, M., Bian, L., Wang, C., Shimizu, T., Yamashiro, Y., Kiyoshima-Shibata, J., Nanno, M., & Nomoto, K. (2010). Well controlled proinflammatory cytokine responses of Peyer’s patch cells to probiotic Lactobacillus casei. Immunology, 130(3), 352–362. https://doi.org/10.1111/j.1365-2567.2009.03204.x
- Chowdhury, S. D., Paik, I. K., Namkung, H., & Lim, H. S. (2004). Responses of broiler chickens to organic copper fed in the form of copper-methionine chelate. Animal Feed Science and Technology, 115(3-4), 281–293. https://doi.org/10.1016/j.anifeedsci.2004.03.009
- Coffman, T., Spurney, R., Mannon, R., & Levenson, R. (1998). Thromboxane A2 modulates the fibrinolytic system in glomerular mesangial cells. American Journal of Physiology, 275(2), 262–269. https://doi.org/10.1152/ajprenal.1998.275.2.F262
- Cohen, D., Soroka, Y., Ma’or, Z., Oron, M., Portugal-Cohen, M., Brégégère, F. M., Berhanu, D., Valsami-Jones, E., Hai, N., & Milner, Y. (2013). Evaluation of topically applied copper(II) oxide nanoparticle cytotoxicity in human skin organ culture. Toxicology in Vitro, 27(1), 292–298. https://doi.org/10.1016/j.tiv.2012.08.026
- Cray, C., Zaias, J., & Altman, N. H. (2009). Acute phase response in animals: A review. Comparative Medicine, 59(6), 517–526.
- Cromwell, G. L., Stahly, T. S., & Monegue, H. J. (1989). Effects of source and level of copper on performance and liver copper stores in weanling pigs. Journal of Animal Science, 67(11), 2996–3002. https://doi.org/10.2527/jas1989.67112996x
- Das A., Mishra S.K., Swain R.K., Sahoo G., Behura N.C., Sethi K., Chichilich B., Mishra S.R., Behera T., Dhama K., Swain P., (2014). Effects of organic minerals supplementation on growth, bioavailability and immunity in layer chicks. International Journal of Pharmacology, 10(5), 237–247. https://doi.org/10.3923/ijp.2014.237.247
- Dennis, W. T., Rocha, P. N., Nataraj, C., Robinson, L. A., Spurney, R. F., Koller, B. H., & Coffman, T. M. (2003). Proinflammatory actions of thromboxane receptors to enhance cellular immune responses. The Journal of Immunology, 171(12), 6389–6395. https://doi.org/10.4049/jimmunol.171.12.6389
- Dorris, S. L., & Peebles, R. S. (2012). PGI 2 as a regulator of inflammatory diseases. Mediators of Inflammation, 926968. https://doi.org/10.1155/2012/926968
- Eckersall, P. D., & Bell, R. (2010). Acute phase proteins: Biomarkers of infection and inflammation in veterinary medicine. The Veterinary Journal, 185(1), 23–27. https://doi.org/10.1016/j.tvjl.2010.04.009
- El-Ghalid, O. A. H., El Ashry, Ghada M., Soliman, M. S., & Abd El-Hady, A. (2019). Effect of dietary sources and levels of copper supplementation on growth performance, blood parameters and slaughter traits of broiler chickens. Egyptian Poultry Science Journal, 39(4), 897-912 https://doi.org/10.21608/epsj.2019.67513
- El-kazaz, S. E., & Hafez Hafez, M. (2020). Evaluation of copper nanoparticles and copper sulfate effect on immune status, behavior, and productive performance of broilers. Journal of Advanced Veterinary and Animal Research, 7(1), 16–25. https://doi.org/10.5455/javar.2020.g388
- Esenbuğa, N., Macit, M., Karaoglu, M., Aksu, M. I., & Bilgin, O. C. (2008). Effects of dietary humate supplementation to broilers on performance, slaughter, carcass and meat colour. Journal of the Science of Food and Agriculture, 88(7), 1201–1207. https://doi.org/10.1002/jsfa.3199
- Fry, R. S., Ashwell, M. S., Lloyd, K. E., O'Nan, A. T., Flowers, W. L., Stewart, K. R., & Spears, J. W. (2012). Amount and source of dietary copper affects small intestine morphology, duodenal lipid peroxidation, hepatic oxidative stress,and mRNA expression of hepatic copper regulatory proteins in weanling pigs1,2. Journal of Animal Science, 90(9), 3112–3119. https://doi.org/10.2527/jas.2011-4403
- Gengelbach, G. P., Ward, J. D., & Spears, J. W. (1994). Effect of dietary copper, iron, and molybdenum on growth and copper status of beef cows and calves. Journal of Animal Science, 72(10), 2722–2727. https://doi.org/10.2527/1994.72102722x
- Godoy, S., Chicco, C., Meschy, F., & Requena, F. (2005). Phytic phosphorus and phytase activity of animal feed ingredients. Interciencia, 30, 24–28.
- Goetzl, E., An, S., & Smith, W. (1995). Specificity of expression and effects of eicosanoid mediators in normal physiology and human diseases1. The FASEB Journal, 9(11), 1051. https://doi.org/10.1096/fasebj.9.11.7649404
- Heeb, L. E. M., Egholm, C., & Boyman, O. (2020). Evolution and function of interleukin-4 receptor signaling in adaptive immunity and neutrophils. Genes & Immunity, 21(3), 143–149. https://doi.org/10.1038/s41435-020-0095-7
- Hochepied, T., Berger, F. G., Baumann, H., & Libert, C. (2003). α1-Acid glycoprotein: An acute phase protein with inflammatory and immunomodulating properties. Cytokine Growth Factor Review, 14(1), 25–34. https://doi.org/10.1016/S1359-6101(02)00054-0
- Hong, S. J., Lim, H. S., & Paik, I. K. (2002). Effects of Cu and Zn-methionine chelates supplementation on the performance of broiler chickens. Journal of Animal Science and Technology, 44(4), 399–406. https://doi.org/10.5187/JAST.2002.44.4.399
- Hopkins, R. G., & Failla, M. L. (1995). Chronic intake of a marginally low copper diet impairs lymphocyte and neutrophil function in males rats despite minimal impact on conventional indices of copper status. Journal of Nutrition, 125(10), 2658–2668. https://doi.org/10.1093/jn/125.10.2658
- Huyghebaert, G., Ducatelle, R., & van Immerseel, F. (2011). An update on alternatives to antimicrobial growth promoters for broilers. The Veterinary Journal, 187(2), 182–188. https://doi.org/10.1016/j.tvjl.2010.03.003
- Jarosz, Ł, Marek, A., Grądzki, Z., Kwiecień, M., & Kaczmarek, B. (2018). The effect of feed supplementation with a copper-glycine chelate and copper sulphate on selected humoral and cell-mediated immune parameters, plasma superoxide dismutase activity, ceruloplasmin and cytokine concentration in broiler chickens. Journal of Animal Physiology and Animal Nutrition, 102(1), e326–e336. https://doi.org/10.1111/jpn.12750
- Jegede, A. V., Oduguwa, O. O., Oso, A. O., Fafiolu, A. O., Idowu, O. M. O., & Nollet, L. (2012). Growth performance, blood characteristics and plasma lipids of growing pullet fed dietary concentrations of organic and inorganic copper sources. Livestock Science, 145(1-3), 298–302. https://doi.org/10.1016/j.livsci.2012.02.011
- Karimi, A., Sadeghi, A., & Vaziry, A. (2011). The effect of copper in excess of the requirement during the starter period on subsequent performance of broiler chicks. Journal of Applied Poultry Research, 20(2), 203–209. https://doi.org/10.3382/japr.2010-00290
- Klasing, C. K. (1998). Minerals in comparative Avian nutrition. CAB Int. 234-276.
- Kulkarni, R. C., Shrivastava, H. P., & Mandal, A. B. (2012). Effect of copper supplementation on serum trace mineral status and cholesterol in broiler chickens. Indian Journal of Animal Research, 46(3), 313–314.
- Kulkarni, R. C., Shrivastava, H. P., Mandal, A. B., Deo, C., Deshpande, K. Y., Singh, R., & Bhanja, S. K. (2011). Assessment of growth performance, immune response and mineral retention in colour broilers as influenced by dietary iron. Animal Feed Science and Technology, 11(1), 81–90.
- Liao, P., Li, M., Li, Y., Tan, X., Zhao, F., Shu, X., & Yin, Y. (2017). Effects of dietary supplementation with cupreous N-carbamylglutamate (NCG) chelate and copper sulfate on growth performance, serum biochemical profile and immune response, tissue mineral levels and fecal excretion of mineral in weaning piglets. Food and Agricultural Immunology, 28(6), 1315–1329. https://doi.org/10.1080/09540105.2017.1339668
- Liao, P., Shu, X., Tang, M., Tan, B., & Yin, Y. (2018). Effect of dietary copper source (inorganicvs.chelated) on immune response, mineral status, and fecal mineral excretion in nursery piglets. Food and Agricultural Immunology, 29(1), 548–563. https://doi.org/10.1080/09540105.2017.1416068
- Libert, C., Van Bladel, S., Brouckaert, P., Shaw, A., & Fiers, W. (1991). Involvement of the liver, but not of IL-6, in IL-1-induced desensitization to the lethal effects of tumor necrosis factor. Journal of Immunology, 146(8), 2625–2632.
- Lim, H. S., & Paik, I. K. (2006). Effects of dietary supplementation of copper chelates in the form of methionine, chitosan and yeast in laying hens. Asian-Australasian Journal of Animal Sciences, 19(8), 1174–1178. https://doi.org/10.5713/ajas.2006.1174
- Mei, S. F., Yu, B., Ju, C. F., Zhu, D., & Chen, D. W. (2010). Effect of different levels of copper on growth performance and cecal ecosystem of newly weaned piglets. Italian Journal of Animal Science, 9(4), 378–381. https://doi.org/10.4081/ijas.2010.e71
- Murata, H., Shimada, N., & Yoshioka, M. (2004). Current research on acute phase proteins in veterinary diagnosis: An overview. The Veterinary Journal, 168(1), 28–40. https://doi.org/10.1016/S1090-0233(03)00119-9
- Narumiya, S., Sugimoto, Y., & Ushikubi, F. (1999). Prostanoid receptors: Structures, properties, and functions. Physiological Reviews, 79(4), 1193–1226. https://doi.org/10.1152/physrev.1999.79.4.1193
- Nataraj, C., Thomas, D. W., Tilley, S., Nguyen, M., Mannon, R., Koller, B., & Coffman, T. (2001). Receptors for prostaglandin E2 that regulate cellular immune responses in the mouse. Journal of Clinical Investigation, 108(8), 1229–1235. https://doi.org/10.1172/JCI200113640
- National Research Council. (1994). Nutrient requirements of poultry, 9th ed. National Academy Press.
- Nguyen, H., Morgan, N., Roberts, J. R., Swick, R. A., & Toghyani, M. (2020). Copper hydroxychloride is more efficacious than copper sulfate in improving broiler chicken’s growth performance, both at nutritional and growth-promoting levels. Poultry Science, 99(12), 6964–6973. https://doi.org/10.1016/j.psj.2020.09.053
- Ognik, K., Sembratowicz, I., Cholewińska, E., Jankowski, J., Kozłowski, K., Juśkiewicz, J., & Zduńczyk, Z. (2018). The effect of administration of copper nanoparticles to chickens in their drinking water on the immune and antioxidant status of the blood. Animal Science Journal, 89(3), 579–588. https://doi.org/10.1111/asj.12956
- O'Reilly, E. L., Bailey, R. A., & Eckersall, P. D. (2018). A comparative study of acute-phase protein concentrations in historical and modern broiler breeding lines. Poultry Science, 97(11), 3847–3853. https://doi.org/10.3382/ps/pey272
- Paik, I. K. (2000). Nutritional management for environment friendly animal production. Asian-Australasian Journal of Animal Sciences, 13, 302–331.
- Paik, I. K. (2001). Application of chelated minerals in animal production. Asian-Australasian Journal of Animal Sciences, 14, 191–198.
- Pang, Y., Patterson, J. A., & Applegate, T. J. (2009). The influence of copper concentration and source on ileal microbiota. Poultry Science, 88(3), 586–592. https://doi.org/10.3382/ps.2008-00243
- Patterson, J. A., & Burkholder, K. M. (2003). Application of prebiotics and probiotics in poultry production. Poultry Science, 82(4), 627–631. https://doi.org/10.1093/ps/82.4.627
- Percival, S. S. (1998). Copper and immunity. The American Journal of Clinical Nutrition, 67(5), 1064S–1068S. https://doi.org/10.1093/ajcn/67.5.1064S
- Pesti, G. M., & Bakalli, R. I. (1996). Studies on the feeding of cupric sulfate pentahydrate and cupric citrate to broiler chickens. Poultry Science, 75(9), 1086–1091. https://doi.org/10.3382/ps.0751086
- Pesti, G. M., & Bakalli, R. I. (1998). Studies on the effect of feeding cupric sulfate pentahydrate to laying hens on egg cholesterol content. Poultry Science, 77(10), 1540–1545. https://doi.org/10.1093/ps/77.10.1540
- Rath, N.C., Anthony, N. B., Kannan, L., Huff, W. E., Huff, G. R., Chapman, H. D., Erf, G. F., & Wakenell, P. (2009). Serum ovotransferrin as a biomarker of inflammatory diseases in chickens. Poultry Science, 88(10), 2069–2074. https://doi.org/10.3382/ps.2009-00076
- Rochman, Y., Spolski, R., & Leonard, W. J. (2009). New insights into the regulation of T cells by γc family cytokines. Nature Reviews Immunology, 9(7), 480–490. https://doi.org/10.1038/nri2580
- Rothwell, L., Young, J. R., Zoorob, R., Whittaker, C. A., Hesketh, P., Archer, A., Smith, A. L., & Kaiser, P. (2004). Cloning and characterization of chicken IL-10 and its role in the immune response to Eimeria maxima. The Journal of Immunology, 173(4), 2675–2682. https://doi.org/10.4049/jimmunol.173.4.2675
- Schneider, K., Klaas, R., Kaspers, B., & Staeheli, P. (2001). Chicken interleukin-6. cDNA structure and biological properties. European Journal of Biochemistry, 268(15), 4200–4206. https://doi.org/10.1046/j.1432-1327.2001.02334.x
- Sevimli, A., Misirlioğlu, D., Yağci, A., Bülbul, A., Yılmaztepe, A., & Altunbas, K. (2008). The role of chicken IL-1β, IL-6 and TNF-α in the occurrence of amyloid arthropathy. Veterinary Research Communications, 32(7), 499–508. https://doi.org/10.1007/s11259-007-9034-6
- Sharma, M. C., Joshi, C., & Das, G. (2008). Therapeutic management of copper deficiency in buffalo heifers: Impact on immune function. Veterinary Research Communications, 32(1), 49–63. https://doi.org/10.1007/s11259-007-9002-1
- Snyder, D. S., Beller, D. I., & Unanue, E. R. (1982). Prostaglandins modulate macrophage Ia expression. Nature, 299(5879), 163–165. https://doi.org/10.1038/299163a0
- Stabel, J. R., Spears, J. W., & Brown, T. T. (1993). Effect of copper deficiency on tissue, blood characteristics, and immune function of calves challenged with infectious bovine rhinotracheitis virus and Pasteurella hemolytica. Journal of Animal Science, 71(5), 1247–1255. https://doi.org/10.2527/1993.7151247x
- Tapiero, H., Townsend, D. M., & Tew, K. D. (2003). Trace elements in human physiology and pathology. Copper. Biomedicine & Pharmacotherapy, 57(9), 386–398. https://doi.org/10.1016/S0753-3322(03)00012-X
- Thies, W. (1991). Determination of the phytic acid and sinapic acid esters in seeds of rapeseed and selection of genotypes with reduced concentrations of these compounds. Fat Science Technology, 93(2), 49–52. https://doi.org/10.1002/lipi.19910930202
- Tilley, S., Coffman, T., & Koller, B. (2001). Mixed messages: Modulation of inflammation and immune responses by prostaglandins and thromboxanes. Journal of Clinical Investigation, 108(1), 15–23. https://doi.org/10.1172/JCI200113416
- Urieli-Shoval, S., Linke, R. P., & Matzner, Y. (2000). Expression and function of serum amyloid A, a major acute-phase protein, in normal and disease states. Current Opinion in Hematology, 7(1), 64–69. https://doi.org/10.1097/00062752-200001000-00012
- Wang, C., Wang, M. Q., Ye, S. S., Tao, W. J., & Du, Y. J. (2011). Effects of copper-loaded chitosan nanoparticles on growth and immunity in broilers. Poultry Science, 90(10), 2223–2228. https://doi.org/10.3382/ps.2011-01511
- Wapnir, R. A. (1998). Copper absorption and bioavailability. The American Journal of Clinical Nutrition, 67(5), 1054S–1060S. https://doi.org/10.1093/ajcn/67.5.1054S
- Winiarska-Mieczan, A., & Kwiecień, M. (2015). The effects of copper-glycine complexes on chemical composition and sensory attributes of raw, cooked and grilled chicken meat. Journal of Food Science and Technology, 52(7), 4226–4235. https://doi.org/10.1007/s13197-014-1510-8
- Xie, H., Huff, G. R., Huff, W. E., Balog, J. M., Holt, P., & Rath, N. C. (2002). Identification of ovotransferrin as an acute phase protein in chickens. Poultry Science, 81(1), 112–120. https://doi.org/10.1093/ps/81.1.112
- Zhao, J., Shirley, R. B., Vazquez-anon, M., Dibner, J., Richards, P., Fisher, T., Hampton, T., Christensen, K. D., Allard, J. P., & Giesen, A. P. (2010). Effects of chelated trace minerals on growth performance, breast meat yield, and footpad health in commercial meat broilers. Journal of Applied Poultry Research, 19(4), 365–372. https://doi.org/10.3382/japr.2009-00020
- Zhou, W., Blackwell, T. S., Goleniewska, K., O’Neal, J. F., Fitzgerald, G. A., Lucitt, M., Breyer, R. M., & Peebles, R. S. (2007). Prostaglandin I2 analogs inhibit Th1 and Th2 effector cytokine production by CD4 T cells. Journal of Leukocyte Biology, 81(3), 809–817. https://doi.org/10.1189/jlb.0606375
- Zhou, W., Kornegay, E. T., van Laar, H., Swinkels, J. W., Wong, E. A., & Lindemann, M. D. (1994). The role of feed consumption and feed efficiency in copper-stimulated growth. Journal of Animal Science, 72(9), 2385–2394. https://doi.org/10.2527/1994.7292385x