ABSTRACT
β-enolase is a heat-labile fish allergen identified in different fish species. In this study, β-enolase gene was cloned from common carp muscle tissue and the target protein was expressed as a fusion with maltose binding protein (MBP-Eno) in E. coli. Recombinant MBP-Eno was reactive with blood serum specimens of fish-allergic patients, confirming that β-enolase is a newly identified allergen of common carp. Two monoclonal antibodies (MAbs) against recombinant β-enolase were generated that showed cross-reactivity with β-enolases of other organisms. Both antibodies recognized β-enolases from other fish species extracts, while MAb 6E4 showed a broad reactivity with pork, chicken, yeast, and E. coli samples. Epitope mapping using MBP-Eno variants revealed that β-enolase region comprising amino acids 623–698 presumably includes MAb 6E4 epitope. The generated broadly reactive MAb 6E4 directed against a conserved epitope of β-enolases may represent a valuable tool for the characterization of allergen extracts and fish allergy diagnostics.
Introduction
Enolase (EC 4.2.1.11), or phosphopyruvate hydratase, is a metalloenzyme that in the presence of Mg2+ ions participates in glucose metabolism by catalyzing the conversion of 2-phosphoglycerate to phosphoenolpyruvate (Nakagawa & Nagayama, Citation1989; Díaz-Ramos et al., Citation2012; Piast et al., Citation2005). This enzyme has been identified in different organisms, from microorganisms to mammals, and its amino acid (aa) sequence among different species is shown to be highly conserved (Simon-Nobbe et al., Citation2000; Morales-Amparano et al., Citation2021). In mammals, the enolase is found in three isoforms (α, β and γ) and is active as a homodimer (α-α, β-β, γ-γ) or heterodimer (α-γ, α-β) (subunits molecular weight (MW) ∼50 kDa) (Díaz-Ramos et al., Citation2012; Kleine-Tebbe & Jakob, Citation2017; Witkowska et al., Citation2005). α-enolase (ENO-1) is found in most tissues, β-enolase (ENO-3) is mainly detected in muscle tissues and γ-enolase (ENO-2) is expressed in neural tissues (Morales-Amparano et al., Citation2021). Enolases play an important role in metabolic pathways of many organisms and participate in the interactions between certain microorganisms and the host cells; however, they can cause allergic reactions to humans (Morales-Amparano et al., Citation2021; Witkowska et al., Citation2005; Marcos et al., Citation2012). It was shown that enolases from various sources, like molds, yeast, plants, animals, or pollens, may cause asthma, rhinitis, urticaria and other allergic reactions to some atopic patients (Morales-Amparano et al., Citation2021).
Allergy to fish is one of the food allergies that affects less than 1% of the world’s population. Clinical symptoms, such as urticaria, vomiting, diarrhea, cough, may occur in patients that are sensitive to one specific fish or to several different fish species. Allergy symptoms can vary depending on selected cooking methods for preparing fish dishes, eating habits, variation in consumed fish species and can affect patients’ skin, respiratory tract, and digestive tract. There are reported cases when fish caused severe allergic reactions that leaded to the anaphylactic shock (Dijkema et al., Citation2020; Klueber et al., Citation2019). Since there is no specific immunotherapy approved to treat fish allergy, sensitized individuals are strictly advised to avoid any kind of fish (Kleine-Tebbe & Jakob, Citation2017). However, a detailed allergological evaluation of the patient could help to determine fish species that can be safely consumed by mono- or oligo-sensitized person not avoiding important nutrients (Dijkema et al., Citation2020; Klueber et al., Citation2019). Parvalbumins are known as the major fish allergens, since most of fish-allergic patients are sensitized to them, while β-enolase, aldolase, collagen, tropomyosin and vitellogenin are considered the minor fish allergens (Matricardi et al., Citation2016; Hilger et al., Citation2017). The first fish enolase was purified and crystalized from a rainbow trout (Oncorhynchus mykiss) in 1966. Later, in 1970 this enzyme was isolated and characterized from two species of Pacific salmon (Oncorhynchus kisutch and Oncorhynchus keta), then in 1983 – from common carp (Cyprinus carpio) and in 1991 from red sea bream (Pagrus major) and Pacific mackerel (Scomber japonicus) (Nakagawa & Nagayama, Citation1989; Cory & Wold, Citation1966; Pietkiewicz et al., Citation1983). In 2013, β-enolase (50 kDa) and aldolase (40 kDa) were purified from the muscle of Atlantic cod (Gadus morhua), Atlantic salmon (Salmo salar), and yellowfin tuna (Thunnus albacares) and were identified as new fish allergens. Based on the cross-inhibition ELISA, it was concluded that both enzymes demonstrated a limited inter-species cross-reactivity. Moreover, β-enolase and aldolase appeared to be native oligomers being sensitive to thermal treatment (Kuehn et al., Citation2013). Another possible cross-reactivity was observed between two β-enolases, prepared from Nile perch (Lates niloticus) and Atlantic cod muscles tissues, where cod-allergic patients were also sensitive to β-enolase from Nile perch (Tomm et al., Citation2013). β-enolase has also been identified from blunt snout bream (Megalobrama amblycephala) and Mozambique tilapia (Oreochromis mossambicus). These data suggest that fish β-enolases are becoming to be recognized as important fish allergens (Liu et al., Citation2011; Liu et al., Citation2012). Currently, there are several β-enolases that are officially recognized as allergens by the World Health Organization/International Union of Immunological Societies (WHO/IUIS) Allergen Nomenclature Database (http://allergen.org): β-enolases isolated from Atlantic cod (Gad m 2), chicken (Gal d 9), striped catfish (Pan h 2), Atlantic salmon (Sal s 2) and yellowfin tuna (Thu a 2). In 2020, common carp β-enolase was identified as a new allergen under the name Cyp c 2 by WHO/IUIS Allergen Database (http://www.allergen.org/viewallergen.php?aid = 1045), after our group showed that several fish-allergic patients were sensitive to recombinant common carp β-enolase.
Common carp belongs to the largest family of freshwater fish, habitat in ponds, lakes, rivers, water reservoirs and is distributed all over the world: from Asia, Europe to Africa, North America, Australia (Rahman, Citation2015; Karnai & Managemet, Citation2018). It is one of the most commonly consumed and produced in aquaculture fish species that can trigger allergy symptoms to fish-allergic patients. Extensive studies on carp parvalbumin demonstrated that this protein contains IgE-binding epitopes, also detected in parvalbumins of various fish species (Baltic cod, chub mackerel, Atlantic salmon). Later, other studies confirmed that parvalbumins are cross-reactive fish allergens and the purified recombinant carp parvalbumin could be used as a tool for the serologic diagnosis of fish allergy (Rahman, Citation2015; Swoboda et al., Citation2002; Sharp & Lopata, Citation2014).
Monoclonal antibodies (MAbs) generated against selected allergen components could be used for more detailed allergen characterization using various methods (immunohistochemistry, radioimmunoassay, Enzyme-Linked ImmunoSorbent Assay (ELISA), Western blot) and could be applied for detection of individual allergens in allergen extracts (Celio et al., Citation1990; Asturias et al., Citation2002). Natural allergen extracts are mixtures of allergens and undefined non-allergenic compounds (proteins, sugars, lipids), obtained from natural allergen sources (Kleine-Tebbe & Jakob, Citation2017). Commercially available allergen extracts are widely used for allergy treatment (in specific immunotherapy) and for allergy testing (in skin prick tests (SPT) and provocation tests). However, these extracts have several disadvantages such as the presence of contaminants, proteases, or unknown non-allergenic components, as well as insufficient amount of certain allergen components. These factors may affect the reactivity of patients with specific allergen components and limit the suitability of allergen extracts for in vivo allergy testing (Ruethers et al., Citation2019; Valenta et al., Citation2018). On the other hand, preparation of standardized allergen extracts from high quality allergen sources could improve their efficacy in specific immunotherapy and their reliability in performed allergy tests (Singh, Citation2018). MAbs against allergen components could be used for more detailed characterization of allergen extracts by detecting the selected component and determination of its total content in the extract. For instance, several different Parietaria judaica extracts were standardized using the MAbs specific to certain Parietaria judaica allergen components (Afferni et al., Citation1995). Another example, MAbs against several Olea europaea pollen-extract components were used to develop a solid-phase radioimmunoassay for the quantitation of the Ole e I allergen in the analyzed extracts (Lombardero et al., Citation1992).
Several monoclonal and polyclonal antibodies have been generated against different enolase isoforms. Rabbit polyclonal antibodies were developed against streptococcal surface enolase (SEN) and human α- and β-enolases, while mouse MAbs were developed against cell surface enolase of Aspergillus fumigatus, streptococcal surface enolase (SEN), human α- and γ-enolases, Plasmodium falciparum enolase and recombinant Chaetomium globosum enolase (Pietkiewicz et al., Citation2018; Yadav & Shukla, Citation2019; Fontán et al., Citation2000; Thomas et al., Citation1987; Lipman et al., Citation2005; López-Alemany et al., Citation2003; Dutta et al., Citation2018; Green et al., Citation2014). To our best knowledge, the MAbs against fish β-enolases and in particular against common carp β-enolase have not yet been reported.
The aim of this study was to clone the β-enolase gene from common carp fish muscle, produce the target protein in Escherichia coli (E. coli) cells, and generate MAbs as a tool for β-enolase detection and investigation. The newly developed MAbs have been characterized using various immunoassays and their cross-reactivity with β-enolases from several fish species and extracts of other organisms has been examined.
Materials and methods
Bacterial strains and cell lines
E. coli strains DH10B (Thermo Fisher Scientific, USA) and Tuner (DE3) (Novagen, Merck, USA), were grown at 37°C in Luria–Bertani (LB) medium (Roth, Germany). LB medium was supplemented with 100 µg/ml ampicillin (Sigma Aldrich, Germany) for recombinant pJET1.2 and 30 µg/ml kanamycin (Sigma Aldrich, Germany) for recombinant pET28-MBP-TEV-based plasmids.
Mouse Sp2/0 myeloma cells used for generation of hybridomas and hybridoma cells were cultured in Dulbecco's modified Eagle's growth medium (DMEM, HyClone, GE Healthcare, USA) supplemented with 9% fetal bovine serum (FBS, Biochrom, UK), 200 μg/mL gentamicin (Carl Roth, Germany) and 2 mM L-glutamine (Sigma Aldrich, Germany), incubated at 37°C and 5% CO2, in a humidified atmosphere.
Isolation of mRNA from common carp tissue and cDNA synthesis
Common carp (Cyprinus carpio) was obtained from a local carp farm. The fish was sacrificed in accordance with the Law on welfare and protection of animals of the Republic of Lithuania and the European Directive 2010/63/UE on the protection of animals used for scientific purposes. mRNA was isolated from a carp skeletal muscle using the Quick-RNA Miniprep Kit (Zymo Research, USA) according to manufacturer’s recommendations. The first strand cDNA was prepared using the RevertAid H Minus First Strand cDNA Synthesis Kit (Thermo Fisher Scientific, USA) according to manufacturer’s instructions.
PCR amplification, cloning and sequencing
The cDNA corresponding to the β-enolase coding gene was amplified to introduce the BglII and SalI sites at 5‘- and 3‘-end of the polymerase chain reaction (PCR) fragment using primers 5’-GAGATCTATGTCCATCAGTAAGATTCACGCTCG and 5’-CGTCGACTCAGAGTTTGGGGTGGCGGAA. PCR was performed with Phusion Flash High-Fidelity PCR Master Mix (Thermo Fisher Scientific, USA) and the amplified DNA fragment was cloned into pJET1.2 vector. Positive colonies were selected and the cloned DNA sequence was verified by sequencing.
Expression of recombinant fusion protein MBP-Eno in E. coli
The recombinant plasmid pJET1.2 bearing the DNA sequence similar to β-enolase-encoding partial sequences (GenBank accession no. LHQP01000860.1 and LHQP01019434.1) was selected. The enolase-encoding DNA fragment was digested with BglII and SalI restriction endonucleases and cloned into BamHI/XhoI-digested pET28-MBP-TEV vector (a gift from Zita Balklava & Thomas Wassmer, Addgene plasmid #69929; http://n2t.net/addgene:69929; RRID:Addgene_69929) (Currinn et al., Citation2016). The resulting construct pET28-MBP-TEV-Cypc2 included the β-enolase encoding gene (abbreviation cypc2) fused to the MBP coding sequence. The synthesis of β-enolase in E. coli Tuner (DE3) cells was induced with 0.1 mM isopropyl β-D-1-thiogalactopyranoside (IPTG) (Sigma Aldrich, Germany). After 4 h of cultivation at 25°C and 37°C, the cells were harvested and disrupted by sonication (Bandelin Sonopuls HD 3100, Bandelin Electronic, Germany). The soluble and insoluble fractions were separated by centrifugation at 32,500 × g for 5 min at 4°C and were analysed on 12% SDS-PAGE under reducing conditions.
Purification of recombinant MBP-Eno
E. coli Tuner (DE3) cells transformed with pET28-MBP-TEV-Cypc2 were cultivated with shaking at 37°C for 2 h until cultures reached OD600 = 0.8. The protein synthesis was induced with 0.1 mM IPTG and the cells were incubated for 4 h at 25°C with shaking. The cells were collected by centrifugation at 4000 × g for 15 min at 4°C, the pellets were washed with buffer (20 mM Tris-HCl, pH 7.4, 200 mM NaCl) followed by centrifugation at 3000 × g for 15 min at 4°C and kept frozen at −20°C.
A total of 1 g wet biomass was suspended in 5 ml of lysis buffer (20 mM sodium phosphate, pH 7.4, 200 mM NaCl, 1 mM EDTA, 1 mM 1,4-dithiothreitol (DTT), 1 mM phenylmethylsulphonyl fluoride). The cells were disrupted by sonication. The supernatant was clarified by centrifugation at 20,000 × g for 20 min at 4°C and diluted (ratio 1:6) with the 20 mM sodium phosphate buffer (pH 7.4) containing 200 mM NaCl and 1 mM EDTA. The supernatant was filtered through 0.45 μm hydrophilic polyvinylidene difluoride (PVDF) membrane (Merck Millipore, Hertfordshire, UK). Purification of recombinant MBP-tagged enolase protein was performed on the AKTA purifier 100 chromatography system equipped with the sample pump P-960 and the fraction collector Frac-920 (GE Healthcare Bio-Sciences AB, Uppsala, Sweden). The protein was loaded on 1 ml MBPTrap HP column prepacked with Dextrin Sepharose High Performance (GE Healthcare, USA), equilibrated with binding buffer (20 mM sodium phosphate, pH 7.4, 200 mM NaCl, 1 mM EDTA). The protein was eluted with binding buffer containing 10 mM maltose. Fractions containing the highest amount of the pure protein were pooled and transfered to the storage buffer (binding buffer with added 10 mM maltose). The concentration of the purified protein was determined by the Bradford assay using bovine serum albumin as a standard. The protein was stored at 4°C.
Expression of truncated MBP-Eno variants in E. coli
Three truncated overlapping recombinant MBP-Eno variants, MBP-Eno-C1, MBP-Eno-C2, and MBP-Eno-C3, were produced in E. coli. For MBP-Eno-C1, a synthetic DNA fragment 5’-TCGAGTAAGCGGCCGCTT-3’ was inserted into BamHI-cleaved pET28-MBP-TEV-Cypc2 (open access: https://bccm.belspo.be/catalogues/lmbp-plasmids-plasmid-details?NM = pET-28a-MBP-TEV-Cypc2) plasmid. The resulting plasmid pET28-MBP-TEV-Cypc2-C1 was transformed into E. coli Tuner (DE3) strain. The synthesis of MBP-Eno-C1 was induced with 0.1 mm IPTG. After 4 h of cultivation at 25°C, the cells were collected by centrifugation at 0.8 × g for 5 min.
For MBP-Eno-C2, XhoI-cleaved pET28-MBP-TEV-Cypc2 plasmid was ligated with the synthetic DNA fragment 5’-GATCCATAAGCGGCCGCT-3’. The resulting plasmid was named pET28-MBP-TEV-Cypc2-C2.
For MBP-Eno-C3, the synthetic cypc2 gene (435 bp, truncated at 5‘-end) coding for Eno-C3 protein was inserted into BamHI/XhoI-digested pET28-MBP-TEV plasmid. The resulting plasmid was named pET28-MBP-TEV-Cypc2-C3. Synthesis of MBP-Eno-C2 and MBP-Eno-C3 was performed as described for MBP-Eno-C1.
Cleavage of recombinant MBP-Eno with TEV protease
For a proteolytic cleavage, 5–10 μg of purified recombinant MBP-Eno protein was incubated with TEV protease (ratio 20:1), reduced glutathione (GSH) (a final concentration 3 mM), and oxidized glutathione (GSSG) (a final concentration 3 mM) at room temperature (RT) overnight. The efficiency of proteolytic cleavage was evaluated by SDS-PAGE and Western blot.
Generation of MAbs against recombinant MBP-Eno
BALB/c mice (6–8 week old, female) were immunized 3 times by a subcutaneous injections of 50 μg of purified recombinant MBP-Eno protein (dissolved in phosphate-buffered saline (PBS), pH 7.4) every 4 weeks: for the primary immunization the antigen was mixed with the complete Freund‘s adjuvant (Thermo Fisher Scientific, USA); for the secondary immunization the antigen was mixed with the incomplete Freund‘s adjuvant (Thermo Fisher Scientific, USA) and the boosted dose was injected without any adjuvant. The maintenance of mice and experimental procedures were performed by certified staff in accordance with FELASA guidelines and conformed to Lithuanian and European legislation in the Department of Biological Models (Institute of Biochemistry, Life Sciences Center, Vilnius University). The permission to use BALB/c mice for immunizations was obtained from the Lithuanian State Food and Veterinary Agency (permission No. G2-117, issued 11 June 2019). Throughout all immunization process the titres of antibodies specific to the antigen were tested by indirect ELISA, allowing to select the immunized mouse with the best response to the antigen. Hybridization was performed essentially as described by Kohler and Milstein (Köhler & Milstein, Citation1975). Three days before cell fusion, the selected mouse was boosted subcutaneously with 50 μg of MBP-Eno protein in PBS. The spleen cells of the immunized mouse were fused with mouse myeloma Sp2/0 cells by incubating cells with polyethylene glycol solution (PEG-4000, Sigma-Aldrich, Germany) in growth medium (fusion ratio 4.5:1). Hybridomas were selected using growth medium supplemented with 15% FBS and hypoxanthine/aminopterin/thymidine (HAT, Sigma-Aldrich, Germany). Within 10–12 days after fusion, when hybrid clones appeared, hybridoma growth medium was screened by an indirect ELISA for antigen-specific antibodies using recombinant MBP-Eno and MBP protein (as a negative control). Hybrid cells producing antibodies specific to MBP-Eno and non-reactive with MBP were cloned by a limiting dilution assay. Within 7–10 days after cloning, viable cell clones were tested by an indirect ELISA and the selected clones were propagated, then cultivated in vitro or frozen for a storage in liquid nitrogen.
Indirect ELISA
This assay was used to analyze blood samples of immunized mice, hybridoma supernatants for selection of positive clones and to investigate MAb specificity. Mutiwell polystyrene plates (MaxiSorp, Thermo Fisher Scientific, USA) were coated either with recombinant protein or allergen extract (50 µl per well) diluted in coating buffer (0.05 M sodium carbonate buffer, pH 9.5), to a concentration of 5 µg/mL or 10 µg/mL, respectively, and incubated overnight at 4°C. Plates were blocked with 200 µl/well of 1x RotiBlock (Roth, Germany) in deioinized water for 1 h at RT and then washed two times with water. Mouse blood samples diluted 1:200–1:145800 in PBS-T (0.1% Tween 20 in PBS), and undiluted hybridoma supernatants were added to the wells (50–150 µl/well) and incubated for 1 h at RT. Then the plates were washed 5 times with PBS-T and incubated for 1 h at RT with goat anti-mouse IgG antibody conjugated to horseradish peroxidase (HRP) (Bio-Rad, USA) (50 µl per well) diluted 1:5000 in PBS-T. After washing the plates 5 times with PBS-T, 50 µl of 3,3′,5,5′-tetramethylbenzidine (TMB) substrate (Clinical Science Products, USA) was added to the wells and incubated for 5–15 min at RT in the dark. The reaction was stopped by adding 25 µl/well of 3.6% sulfuric acid. The optical density (OD) was measured at 450 nm (reference filter 620 nm) using plate spectrophotometer (Multiscan GO, Thermo Fisher Scientific, USA).
To investigate the reactivity of MBP-Eno with IgE from blood serum samples of fish-allergic patients, 96-well plates were coated with the purified recombinant MBP-Eno and MBP proteins (50 µl per well) diluted in coating buffer (a final concentration 5 µg/mL), and incubated overnight at 4°C. After blocking plates with 1x RotiBlock for 1 h at RT, human serum samples from patients with a confirmed fish allergy (PlasmaLab International, USA) diluted 1:10–1:90 in PBS-T were added to the wells and incubated for 2 h at RT. Afterwards, the plates were washed with PBS-T and incubated with mouse anti-human IgE Fc-HRP (SouthernBiotech, USA) diluted 1:1000 in PBS-T for 1 h at RT. Washing of the plates and detection of the enzymatic reaction were performed as above.
Determination of MAb isotypes and the apparent dissociation constant (Kd)
MAbs isotypes were determined using the Mouse Immunoglobulin Isotyping ELISA Kit (BD Biosciences, USA) according to the manufacturer's protocol.
The apparent dissociation constants (Kd) of the MAbs were determined by an indirect ELISA. The 96 well plates were coated with recombinant MBP-Eno protein (5 µg/mL). After blocking, the wells were incubated for 1 h at RT with the purified MAbs (prepared in concentrations ranging from 3.3 × 10−8 M to 1.863 × 10−13 M) diluted in PBS-T. The values of MAb Kd were calculated from titration curves as described previously (Zvirbliene et al., Citation2010), using scientific data analysis and graphing software (OriginPro 9.1, OriginLab).
MAb purification from hybridoma supernatants
MAbs were purified from hybridoma supernatants, diluted with washing/binding buffer (1.5 M glycine, pH 8.9, 3 M NaCl) (ratio 1:3), using AKTA purifier 100 chromatography system equipped with the sample pump P-960 and the fraction collector Frac-920 (GE Healthcare Bio-Sciences AB, Uppsala, Sweden) and 1 ml HiTrap® Protein A High Performance (GE Healthcare, USA) column. The column was equilibrated with washing/binding buffer and protein elution was carried out with elution buffer (100 mM glycine, pH 3.0). Purified antibodies were dialyzed overnight at 4°C against PBS. Collected MAbs samples were sterile filtered, stored at 4°C and antibodies concentrations were determined using NanoDrop 2000 spectrophotometer (Thermo Fisher Scientific, USA).
Western blot
Recombinant proteins, cell lysates and allergen extracts were subjected to sodium dodecyl sulfate-polyacrylamide gel electrophoresis (SDS-PAGE) under reducing conditions.
After SDS-PAGE, proteins were transferred to a polyvinyldifluoride (PVDF) membrane (Carl Roth, Germany). The membranes were blocked with 2% milk powder in PBS for 1 h at RT. After washing with PBS-T, the membranes were incubated with diluted hybridoma supernatants in a ratio of 1:2 or with purified MAb (a final concentration 5 µg/ml) in 2% milk powder in PBS-T for 1 h at RT. After washing, the membranes were incubated with goat anti-mouse IgG antibody conjugated to HRP (Bio-Rad, USA) diluted 1:4000 in 2% milk powder in PBS-T for 1 h at RT. Following a wash cycle, the membrane was treated with 1-Step™ TMB-Blotting Substrate Solution (Thermo Fisher Scientific, USA) for 5–10 min. The enzymatic reaction was stopped by washing membranes in deionized water.
Dot blot
Samples (2 μl) of purified recombinant MBP-Eno and MBP proteins (0.1–1 μg, diluted in PBS), either non-denaturated or denaturated by boiling for 10 min at 100°C, were spotted onto the nitrocellulose membrane (Carl Roth, Germany) in a series of small dots. Membranes were dried for 20–30 min at RT and then blocked with 2% BSA in PBS-T for 1 h at RT. After washing the membranes twice with PBS-T, they were incubated with patients’ sera diluted 1:30 in PBS-T supplemented with 2% BSA for 2 h at RT. The membranes were washed with PBS-T and incubated with mouse anti-human IgE Fc-HRP (SouthernBiotech, USA) diluted 1:1000 in PBS-T supplemented with 2% BSA for 1 h at RT. The subsequent membrane washing and treatment with TMB-Blotting Substrate Solution was performed as described for Western blot analysis.
Fish allergen extracts and in-house prepared extracts
Commercial fish extracts were purchased from two different manufacturers (DST, Germany and Labor Dr. Weyers, Germany).
In-house allergen extracts of chicken (Gallus domesticus) and pork (Sus scrofa domesticus) were prepared from chilled muscle tissues purchased from a local store. A piece of muscle tissue (1–3 g) was homogenized in PBS supplemented with 150 mM NaCl (volume of each homogenate is ∼15 ml) using the glass Dounce homogenizer (5 min on ice). The supernatant was clarified by centrifugation at 4000 × g for 15 min at 4°C, and stored at −20°C.
Baker’s yeast extract was prepared by dissolving 300 mg of Baker’s yeast (obtained from a local store) in 1 ml of PBS supplemented with 150 mM NaCl. The prepared extract was stored at −20°C. Protein concentration in the prepared extracts was determined using the Bradford assay.
Results
Expression and purification of recombinant common carp β-enolase fused to maltose binding protein
Common carp β-enolase coding gene was amplified from cDNA synthesized from the mRNA extracted from the skeletal muscle of a common carp (Cyprinus carpio). After cloning the 1316 bp-long PCR fragment into pJET1.2 vector, we selected the recombinant plasmids bearing the cloned sequences that showed high similarity with that of β-enolase partial sequences published in GenBank.
Recombinant β-enolase with the N-terminal MBP (MBP-Eno) was produced in E. coli. Recombinant fusion protein (calculated molecular weight 91.7 kDa) was found in both soluble and insoluble fractions of the cell lysates. A fraction of soluble MBP-Eno obtained after the induction of protein synthesis at 25°C () was subjected to purification via affinity chromatography using MBPTrap HP column prepacked with Dextrin Sepharose (Supplementary Figure S1). The yield of the purified MBP-Eno was about 5 mg from 1 g of wet E. coli biomass.
Figure 1. SDS-PAGE analysis of recombinant MBP-Eno protein in E. coli lysates. After induction of protein synthesis, E. coli cells were grown at 25°C and 37°C. Lane 1: E. coli lysate before induction; lanes 2, 5: soluble fraction of cell lysates after induction; lanes 3, 6: insoluble fraction of cell lysates after induction; lanes 4, 7: total cell lysate after induction; lane 8: protein molecular weight marker (Thermo Fisher Scientific, USA). The arrow indicates the migration position of recombinant MBP-Eno.
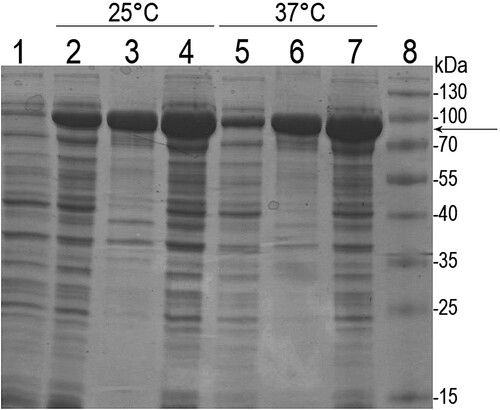
Antigenic characterization of MBP-Eno
To analyze whether common carp β-enolase is an allergen, the reactivity of the purified recombinant MBP-Eno with immunoglobulins E (IgE) present in serum samples of patients with diagnosed fish allergy was tested by ELISA and dot blot. Among 18 well-characterized serum specimens (PlasmaLab International, USA), 4 were found to be reactive with non-denatured recombinant MBP-Eno by ELISA and only 3 specimens by dot blot (). The MBP-Eno-positive serum samples did not react with MBP used as a negative control that confirms the specificity of the assay. The data also demonstrate that common carp β-enolase is a newly identified human allergen. It was included into WHO/IUIS Allergen Database under the name Cyp c 2.
Figure 2. The reactivity of serum IgE of fish-allergic patients with purified recombinant MBP-Eno determined by dot blot (A) and ELISA (B). As a negative control, purified MBP was used. S1–S4: serum specimens of patients with confirmed fish allergy; C1: serum specimen of a patient with other allergies; C2: serum specimen of a healthy (non-atopic) individual. Different amounts of denatured and non-denatured MBP-Eno were used in dot blot. Serum samples analyzed by ELISA were diluted in a ratio of 1:10 and measured without replicate. OD indicates optical density.
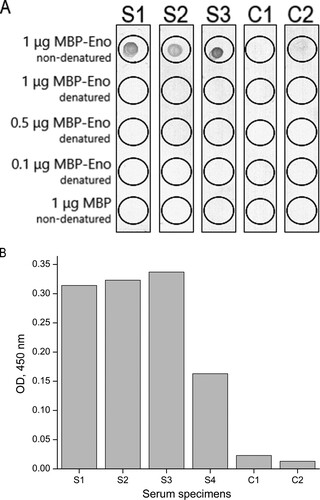
Generation of MAbs against recombinant MBP-Eno
For the production of MAbs, BALB/c mice were immunized with purified recombinant MBP-Eno. Hybridomas were generated by fusion of mouse myeloma cells with spleen cells isolated from the immunized mouse with the highest antibody titer (1:16200). Hybridoma clones were screened by an indirect ELISA using purified recombinant MBP-Eno and MBP protein as a negative control to identify only β-enolase-specific clones. Hybridomas producing antibodies specific to MBP-Eno and non-reactive with MBP were cloned by limiting dilution assay and later tested again for their specificity for β-enolase. In total, two stable hybridoma cell lines producing MAbs of IgG isotype against β-enolase were developed: clones 14F3 and 6E4. The supernatants of these hybridomas were used for the purification of MAbs and their characterization.
Determination of the isotypes and the specificity of the MAbs
The results of an indirect ELISA showed that both antibodies are of IgG2b subtype and react with recombinant MBP-Eno (). The ability of the MAbs to recognize SDS-denatured MBP-Eno was tested by Western blot. In addition, MAb reactivity with recombinant β-enolase (calculated molecular weight 47.6 kDa) cleaved from MBP-Eno by TEV protease was tested by Western blot. Recombinant MBP protein was used as a negative control. Only MAb 6E4 reacted with both MBP-Eno and β-enolase in Western blot (Supplementary Figure S2). Since MAb 14F3 did not show any reactivity with recombinant target antigens under denaturing conditions, it was concluded that this antibody recognizes a conformation-sensitive epitope of β-enolase.
Table 1. Properties of MAbs raised against recombinant MBP-Eno protein.
To determine the affinity of the generated MAbs to recombinant β-enolase, the apparent dissociation constant (Kd) values of each antibody were calculated from the results of an indirect ELISA, indicating that both MAbs are of high affinity with Kd values 3.04 × 10−10 and 7.64 × 10−8 for MAb 14F3 and MAb 6E4, respectively ().
Investigation of the cross-specificity of the MAbs
To investigate the ability of MAbs to detect β-enolase in natural extracts from carp and other fish species (cod, salmon and herring), fish allergen extracts from two different manufacturers were tested by ELISA and Western blot. Both antibodies were shown to be cross-reactive by ELISA: MAb 14F3 recognized β-enolase in carp extract from the manufacturer M2 and reacted with salmon extracts from both manufacturers, while MAb 6E4 showed reactivity to all fish extracts, except to herring extract from the manufacturer M2. Even though these extracts were prepared from the same fish species but by two individual producers, the analyzed MAbs demonstrated different reactivity strength with the target allergen (Supplementary Figure S3). Western blot of heated fish extracts with MAb 6E4 revealed bands at the positions that correspond to the molecular weight of full-length β-enolase and shorter length proteins that correspond to its partially degraded forms in all fish extracts except the herring extract (). These data suggest that both MAb 14F3 and 6E4 are specific not only to common carp β-enolase, but also cross-react with β-enolases of other fish species.
Figure 3. The reactivity of MAb 6E4 with heat-inactivated fish extracts obtained from diffferent manufacturers. A: fish extracts from manufacturer M1; B: fish extracts from manufacturer M2. The left panel: SDS-PAGE, the right panel: Western blot with MAb 6E4. Lane 1: protein molecular weight marker; lane 2: the cod fish extract; lane 3: the carp extract; lane 4: the herring extract; lane 5: the salmon extract. The migration position of full-length β-enolase indicated by an arrow.
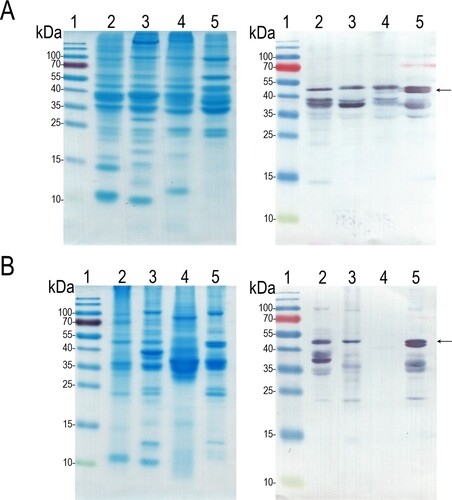
The possible cross-reactivity of MAb 6E4 with enolases from other organisms was investigated by Western blot using E. coli Tuner (DE3) and E. coli DH10B cell lysates, recombinant Alternaria alternata enolase fused with MBP (MBP-Alt a 6), Baker's yeasts extract, and the in-house prepared chicken and pork extracts. In order to eliminate possible non-specific reactivity in Western blot, we also used MAb 19C19 against the recombinant MBP protein as a negative control to prove the binding of MAb 6E4 only to enolase. It was shown, that MAb 6E4 reacted with the recombinant MBP-Eno protein in all analysed E. coli cell lysates that results in the immunostained ∼47 kDa protein corresponding to the E. coli enolase. Moreover, MAb 6E4 reacted with ∼47 kDa proteins and their partially degraded forms in the analyzed yeast extract and in both in-house prepared meat extracts, that corresponded to Saccharomyces cerevisiae enolase, chicken and pork β-enolases, respectively (). MAb 19C19 used as a negative control was reactive with recombinant purified MBP-Eno, MBP-Alt a 6 and MBP proteins, and also recognized MBP-containing proteins in all E. coli cell lysates, but did not show any reactivity with yeast and in-house prepared meat extracts thus confirming the specificity of the assay (data not shown).
Figure 4. The reactivity of MAb 6E4 with enolases from different organisms. A: SDS-PAGE, B: Western blot with MAb 6E4. Lane 1: protein molecular weight marker; lane 2: MBP-Eno in E. coli Tuner (DE3) cell lysate; lane 3: MBP-Alt a 6 in E. coli Tuner (DE3) cell lysate; lane 4: E. coli DH10B cell lysate; lane 5: E. coli Tuner (DE3) cell lysate; lane 6: the Baker‘s yeast extract; lane 7: in-house prepared chicken extract; lane 8: in-house prepared pork extract; line 9: recombinant purified MBP protein. The migration position of enolases indicated by an arrow.
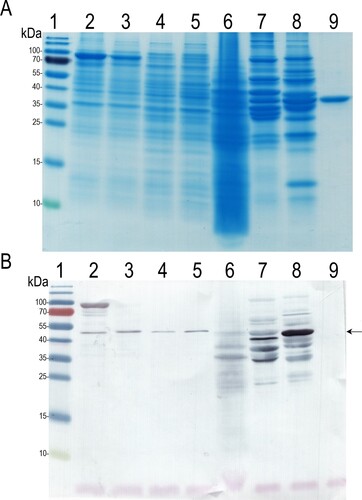
Mapping of the MAb 6E4 epitope
To localize the epitope of recombinant β-enolase (aa 1–836), that is recognized by MAb 6E4, three truncated overlapping recombinant variants of β-enolase were constructed: MBP-Eno-C1 (containing aa 1–698 of β-enolase), MBP-Eno-C2 (aa 1–622) and MBP-Eno-C3 (aa 1–543) (). All these proteins were expressed in E. coli Tuner (DE3) strain. MAb 6E4 reactivity with the lysates of transformed E. coli cells expressing recombinant proteins was investigated by Western blot. It was determined, that antibody MAb 6E4 reacts with recombinant full-length MBP-Eno (91.7 kDa) and recombinant truncated MBP-Eno-C1 (76.3 kDa) protein, but no reactivity was observed with truncated recombinant MBP-Eno-C2 (67.8 kDa) and MBP-Eno-C3 (59.3 kDa) proteins (Supplementary Figure S4). After analysing the overlapping aa sequences of β-enolase in all truncated MBP-Eno proteins it was concluded, that the C-terminally located 76 aa-long fragment of common carp β-enolase spanning aa residues from 623 to 698 (specifically: 623-NNEALELLKSAIEKAGYPDKIIIGMDVAASEFFKNGKYDLDFKSPD DPKRHITGDQLGDLYKSFIKNYPVQSIEDP-698) presumably includes MAb 6E4 epitope (). Sequence alignment of the C-terminal part of different β-enolases revealed a high degree of identity ranging from 70 to 90% across β-enolases of Atlantic salmon, striped catfish and chicken ().
Discussion
Urticaria, rhinitis and diarrhea are the most common allergy symptoms, that could be trigerred by one of the main food allergens – fish. As valuable nutrients, fish dishes become more and more popular in human diets (Kuehn et al., Citation2014; Klueber et al., Citation2019). There are approximately 34,000 described fish species in the world and more than 240 fish allergens are identified and characterized to date (Parenti & Weitzman, Citation2021; Fernandes et al., Citation2015). The amount of consumed fish and the population of certain fish species vary all over the world (Klueber et al., Citation2019). In Europe, the most commonly eaten fish species are cod, salmon and herring, while in Asia – tilapia, barramundi and carp (Kalic et al., Citation2019). Depending on the geographical region, eating habits and fish preparation traditions, allergic patients could be sensitized to one specific fish species or could experience clinical cross-reactions to several different fish species. The only advice for fish-allergic individuals is to avoid eating all fish, even though they would be able to tolerate specific fish species (like tuna, mackerel) (Dijkema et al., Citation2020; Klueber et al., Citation2019). Identification of new and a more detailed study of existing fish allergens is important for developing better fish allergen detection methods, that could be applied for more accurate allergy diagnosis (Stephen et al., Citation2017).
Enolase is a glycolytic enzyme, distributed in various organisms (rabbit, human, chicken, fish, yeast, bacteria and other) (Nakagawa & Nagayama, Citation1989). The first enolase identified as human allergen in 1988 was enolase from Saccharomyces cerevisiae. Later, enolase was shown to be an allergen in other mold and yeast species (Alternaria alternata, Aspergillus fumigatus, Cladosporium herbarum, Penicillium citrinum and ect.) and several recombinant enolases were produced (recombinant Cla h 6, Alt a 6, Asp f 22, Pen c 22, Rho m 1). Moreover, enolase has also been identified and registered as an allergen of plants (Hev b 9) and pollen (Amb a 12, Cyn d 22) along with β-enolase of chicken (Gal d 9) and fish (Gad m 2, Sal s 2, Pan h 2 and Thu a 2) (Simon-Nobbe et al., Citation2000; Morales-Amparano et al., Citation2021). In the current study, we describe production and antigenic characterization of common carp β-enolase, which was registered as the new fish allergen Cyp c 2 by the WHO/IUIS Allergen Database based on its reactivity with serum samples of fish-allergic patients. For more detailed characterization of the newly identified fish allergen, we expressed recombinant common carp β-enolase fused with MBP (MBP-Eno) in E. coli cells, resulting in a soluble protein of ∼91.7 kDa. MBP as a fusion partner was used both to enhance the solubility of the recombinant target protein and as a purification tag because MBP effectively binds to amylose resin (Reuten et al., Citation2016).
Allergen-specific MAbs could be used for standardization of allergen extracts to improve their quality, for the development of new immunoassays for allergen detection, or isolation of the allergen from prepared food or environment samples (Chapman, Citation1988; Atanasio et al., Citation2021). Immunization of mice with recombinant fused protein MBP-Eno resulted in two hybridoma cell lines producing MAbs specific to recombinant common carp β-enolase. Both antibodies, MAb 14F3 and 6E4, showed high affinity to the recombinant MBP-Eno by ELISA, however, only MAb 6E4 recognized linear epitopes of the target antigen by Western blot. To our best knowledge, the MAbs against fish β-enolase have not yet been described.
Fish β-enolases, like other fish allergens parvalbumins, were shown to share sequence similarity among different fish species (Kuehn et al., Citation2013). The aa sequence of common carp β-enolase (Cyp c 2) was aligned with several aa sequences of other fish β-enolases from WHO/IUIS Allergen Database: Sal s 2 and Pan h 2, using protein Blast from NCBI. The alignment revealed more than 90% identity of aa sequences among these fish β-enolases. Therefore, we reasonably considered that the newly generated MAbs 14F3 and 6E4 could also react with other fish β-enolases. MAb cross-reactivity was tested using commercial fish extracts from two different manufacturers. Both MAbs demonstrated specificity to β-enolases of common carp and other fish species, suggesting that common carp, Atlantic herring, Atlantic salmon and Atlantic cod β-enolases contain common epitopes that are recognized by these MAbs and proving that these allergens share high aa sequence identity. Moreover, MAb 6E4 demonstrated a similar cross-reactivity pattern with heated and SDS-denatured fish extracts in Western blot, providing an important information that the amount of β-enolase in allergen extracts not only differs among different fish species, but also among manufacturers. The content of fish allergens in several commercial fish extracts from different manufacturers was analyzed in previous studies (Ruethers et al., Citation2019). They showed that the amount of fish allergens (parvalbumins, β-enolases, aldolases A, collagen and tropomyosin) also varies in allergen extracts and their content should be standartized to improve their suitability for fish alllergy diagnostics. There are several studies, where allergen-specific MAbs were used for the characterization of allergen extracts of Parietaria judaica, Dermatophagoides pteronyssinus and Dermatophagoides farinae (Afferni et al., Citation1995; Luczynska et al., Citation1989). Since the newly generated MAbs are cross-reactive with β-enolases of several different fish species, they could be highly useful to analyse the amount of β-enolase in commercial or in-house prepared extracts of common carp, Atlantic herring, Atlantic salmon or Atlantic cod fish and improve their quality for further applications.
Besides fish, enolases from fungi, plants and animals also share high sequence identity accross each group: the similarity of enolases among fungi is ≥72%, in plants ≥86% and in animals ≥81% (Morales-Amparano et al., Citation2021). Moreover, enolase isolated from yeast Candida albicans was shown to have a similar aa sequence to Saccharomyces cerevisiae enolase. In other study, IgE cross-reactivity between recombinant Hevea latex enolase (rHev b 9) and rCla h 6 and rAlt a 5 was demonstrated (Ito et al., Citation1995; Wagner et al., Citation2000). Since enolase aa sequences share high similarity among different organisms, we investigated the reactivity of MAb 6E4 with several selected enolases by Western blot and demonstrated, that MAb 6E4 recognizes enolases from E. coli and Saccharomyces cerevisiae, as well as β-enolase from chicken and pork. After aligning aa sequences of Cyp c 2 with E. coli (UniProtKB accession no. P0A6P9) and Saccharomyces cerevisiae (UniProtKB accession no. P00924) we determined that sequence identities are 54% and 65%, respectively, suggesting that MAb 6E4 recognizes a highly conserved aa sequence of these antigens. Moreover, MAb 6E4 was shown to recognize Gal d 9 in the in-house prepared chicken extract. The reactivity of MAb 6E4 with this allergen could be explained by the high homology between Cyp c 2 and Gal d 9 (aa sequences share 84% identity). The cross-reaction between chicken β-enolase (Gal d 9) and Atlantic cod β-enolase (Gad m 2) was demonstrated by Kuehn et al in 2016 (Kuehn et al., Citation2016). Based on the results of our experiments and previous studies, we assume that Gal d 9 could share common epitopes with β-enolases of other animals. Additional studies are needed to evaluate the possible cross-reactivity of antibodies raised against β-enolases from other fish species and enolases from bacteria, yeast and other organisms. Currently, there is no information about the allergenic potential of pork β-enolase, however, the results presented here on fish β-enolases may promote the identification of new meat allergens and further studies on their possible antigenic similarity with fish and chicken allergens.
The final step in our study was to predict the localization of MAb 6E4 epitope shown to be highly conserved accross different enolases. For this purpose, we synthesised three MBP-Eno variants comprising truncated overlapping sequences of β-enolase. MAb 6E4 reacted with MBP-Eno-C1 protein and did not react with other two truncated MBP-Eno variants, allowing us to identify 76 aa-long sequence (aa 623–698) comprising the epitope of MAb 6E4. The aa sequence recognized by the MAb 6E4 was aligned with aa sequences of other fish (striped catfish and Atlantic salmon) and chicken β-enolases, showing similarity more than 90% and 70%, respectively. This high aa sequence identity could explain the cross-reactivity of MAb 6E4 with β-enolases from various organisms and its potential application in the standartization of fish allergen extracts.
In conclusion, common carp β-enolase was sucessfully cloned from fish muscle, produced in E.coli and proven as a new allergen based on its reactivity with blood serum specimens of fish-sensitized patients. Two MAbs were raised against recombinant MBP-Eno and characterized by different immunoassays, confirming MAb specificity to β-enolase. The demonstrated cross-reactivity of the MAbs with β-enolases of other fish species suggest that the newly developed MAbs, in particular the broadly-reactive clone 6E4, could become a promising universal tool for the characterization and standardization of fish allergen extracts.
Supplemental Material
Download MS Word (773 KB)Disclosure statement
No potential conflict of interest was reported by the author(s).
Data availability statement
The authors confirm that the data supporting the findings of this study are available within the article.
Additional information
Funding
References
- Afferni, C., Pini, C., Tinghino, R., Palumbo, S., Biocca, M. M., Bruno, G., Mari, A., & Di Felice, G. (1995). Use of monoclonal antibodies in the standardization of Parietaria judaica allergenic extracts. Biologicals, 23(3), 239–247. https://doi.org/10.1006/biol.1995.0040
- Asturias, J. A., Gómez-Bayón, N., Arilla, M. C., Sánchez-Pulido, L., Valencia A., & Martínez, A. (2002). Molecular and structural analysis of the panallergen profilin B cell epitopes defined by monoclonal antibodies. International Immunology, 14(9), 993–1001. https://doi.org/10.1093/intimm/dxf070
- Atanasio, A., Franklin, M. C., Kamat, V., Hernandez, A. R., Badithe, A., Ben, L.-H., Jones, J., Bautista, J., Yancopoulos, G. D., Olson, W., Murphy, A. J., Sleeman, M. A., & Orengo, J. M. (2021). Targeting immunodominant Bet v 1 epitopes with monoclonal antibodies prevents the birch allergic. Journal of Allergy and Clinical Immunology, 149(1), 200–211. https://doi.org/10.1016/j.jaci.2021.05.038
- Celio, M. R., Baier, W., Schärer, L., Gregersen, H. J., de Viragh, P. A., & Norman, A. W. (1990). Monoclonal antibodies directed against the calcium binding protein calbindin D-28k. Cell Calcium, 11(9), 599–602. https://doi.org/10.1016/0143-4160(90)90014-l
- Chapman, M. D. (1988). Allergen specific monoclonal antibodies: New tools for the management of allergic disease. Allergy, 43(Suppl 5), 7–14. https://doi.org/10.1111/j.1398-9995.1988.tb05042.x
- Cory, R. P., & Wold, F. (1966). Isolation and characterization of enolase from rainbow trout (Salmo gairdnerii gairdnerii). Biochemistry, 5(10), 3131–3137. https://doi.org/10.1021/bi00874a008
- Currinn, H., Guscott, B., Balklava, Z., Rothnie, A., & Wassmer, T. (2016). APP controls the formation of PI(3,5)P(2) vesicles through its binding of the PIKfyve complex. Cellular and Molecular Life Sciences, 73(2), 393–408. https://doi.org/10.1007/s00018-015-1993-0
- Díaz-Ramos, A., Roig-Borrellas, A., García-Melero, A., & López-Alemany, R. (2012). α-Enolase, a multifunctional protein: Its role on pathophysiological situations. Journal of Biomedicine and Biotechnology, 2012, 1. https://doi.org/10.1155/2012/156795
- Dijkema, D., Emons, J. A. M., Van de Ven, A. A. J. M., & Oude Elberink, J. N. G. (2020). Fish allergy: Fishing for novel diagnostic and therapeutic options. Clinical Reviews in Allergy & Immunology, 62(1), 64−71. https://doi.org/10.1007/s12016-020-08806-5
- Dutta, S., Tewari, A., Balaji, C., Verma, R., Moitra, A., Yadav, M., Agrawal, P., Sahal, D., & Jarori, G. K. (2018). Strain-transcending neutralization of malaria parasite by antibodies against Plasmodium falciparum enolase. Malaria Journal, 17(1), 304. https://doi.org/10.1186/s12936-018-2455-6
- Fernandes, T. J. R., Costa, J., Oliveira, M. B. P. P., & Mafra, I. (2015). An overview on fish and shellfish allergens and current methods of detection. Food and Agricultural Immunology, 26(6), 848–869. https://doi.org/10.1080/09540105.2015.1039497
- Fontán, P. A., Pancholi, V., Nociari, M. M., & Fischetti, V. (2000). Antibodies to streptococcal surface enolase react with human alpha-enolase: Implications in poststreptococcal sequelae. The Journal of Infectious Diseases, 182(6), 1712–1721. https://doi.org/10.1086/317604
- Green, B. J., Nayak, A. P., Lemons, A. R., Rittenour, W. R., Hettick, J. M., & Beezhold, D. H. (2014). Production of a Chaetomium globosum enolase monoclonal antibody. Monoclonal Antibodies in Immunodiagnosis and Immunotherapy, 33(6), 428–437. https://doi.org/10.1089/mab.2014.0042
- Hilger, C., van Hage, M., & Kuehn, A. (2017). Diagnosis of Allergy to mammals and fish: Cross-reactive vs. Specific markers. Current Allergy and Asthma Reports, 17(9), 1−12. https://doi.org/10.1007/s11882-017-0732-z
- Ito, K., Ishiguro, A., Kanbe, T., Tanaka, K., & Torh, S. (1995). Characterization of IgE-binding epitopes on Candida albicans enolase. Clinical Experimental Allergy, 25(6), 529–535. https://doi.org/10.1111/j.1365-2222.1995.tb01090.x
- Kalic, T., Morel-Codreanu, F., Radauer, C., Ruethers, T., Taki, A. C., Swoboda, I., Hilger, C., Hoffmann-Sommergruber, K., Ollert, M., Hafner, C., Lopata, A. L., Morisset, M., Breiteneder, H., & Kuehn, A. (2019). Patients allergic to fish tolerate ray based on the low allergenicity of its parvalbumin. The Journal of Allergy and Clinical Immunology: In Practice, 7(2), 500–508.e11. https://doi.org/10.1016/j.jaip.2018.11.011
- Karnai, L., & Managemet, S. (2018). Outlooks and perspectives of the common carp production. Polish Association of Agricultural Economists and Agribusiness - Stowarzyszenie Ekonomistów Rolnictwa i Agrobiznesu (SERiA), XX(1), 64–72. doi:10.5604/01.3001.0011.7230
- Kleine-Tebbe, J., & Jakob, T. (2017). Molecular Allergy diagnostics innovation for a better patient management. Springer.
- Klueber, J., Schrama, D., Rodrigues, P., Dickel, H., & Kuehn, A. (2019). Fish allergy management: From component-resolved diagnosis to unmet diagnostic needs. Current Treatment Options in Allergy, 6(4), 322–337. https://doi.org/10.1007/s40521-019-00235-w
- Köhler, G., & Milstein, C. (1975). Continuous cultures of fused cells secreting antibody of predefined specificity. Nature, 256(5517), 495–497. https://doi.org/10.1038/256495a0
- Kuehn, A., Codreanu-Morel, F., Lehners-Weber, C., Doyen, V., Gomez-André, S.-A., Bienvenu, F., Fischer, J., Ballardini, N., van Hage, M., Perotin, J.-M., Silcret-Grieu, S., Chabane, H., Hentges, F., Ollert, M., Hilger, C., & Morisset, M. (2016). Cross-reactivity to fish and chicken meat - a new clinical syndrome. Allergy, 71(12), 1772–1781. https://doi.org/10.1111/all.12968
- Kuehn, A., Hilger, C., Lehners-Weber, C., Codreanu-Morel, F., Morisset, M., Metz-Favre, C., Pauli, G., de Blay, F., Revets, D., Muller, C. P., Vogel, L., Vieths, S., & Hentges, F. (2013). Identification of enolases and aldolases as important fish allergens in cod, salmon and tuna: Component resolved diagnosis using parvalbumin and the new allergens. Clinical & Experimental Allergy, 43(7), 811–822. https://doi.org/10.1111/cea.12117
- Kuehn, A., Swoboda, I., Arumugam, K., Hilger, C., & Hentges, F. (2014). Fish allergens at a glance: Variable allergenicity of parvalbumins, the major fish allergens. Frontiers in Immunology, 5, 179. https://doi.org/10.3389/fimmu.2014.00179
- Lipman, N. S., Jackson, L. R., Trudel, L. J., & Weis-Garcia, F. (2005). Monoclonal versus polyclonal antibodies: Distinguishing characteristics, applications, and information resources. ILAR Journal, 46(3), 258–268. https://doi.org/10.1093/ilar.46.3.258
- Liu, R., Krishnan, H. B., Xue, W., & Liu, C. (2011). Characterization of allergens isolated from the freshwater fish blunt snout bream (Megalobrama amblycephala). Journal of Agricultural and Food Chemistry, 59(1), 458–463. https://doi.org/10.1021/jf103942p
- Liu, R., Yang, E., Liu, C., & Xue, W. (2012). Tilapia (Oreochromis mossambicus) allergens characterized by ELISA, SDS-PAGE, 2D gels, Western blotting and MALDI-TOF mass spectrometry. International Journal of Food Sciences and Nutrition, 63(3), 259–266. https://doi.org/10.3109/09637486.2011.619966
- Lombardero, M., Quirce, S., Duffort, O., Barber, D., Carpizo, J., Chamorro, M., Lezaun, A., & Carreira, J. (1992). Monoclonal antibodies against Olea europaea major allergen: Allergenic activity of affinity-purified allergen and depleted extract and development of a radioimmunoassay for the quantitation of the allergen. Journal of Allergy and Clinical Immunology, 89(4), 884–894. https://doi.org/10.1016/0091-6749(92)90445-8
- López-Alemany, R., Longstaff, C., Hawley, S., Mirshahi, M., Fábregas, P., Jardí, M., Merton, E., Miles, L. A., & Félez, J. (2003). Inhibition of cell surface mediated plasminogen activation by a monoclonal antibody against alpha-enolase. American Journal of Hematology, 72(4), 234–242. https://doi.org/10.1002/ajh.10299
- Luczynska, C. M., Arruda, L. K., Platts-Mills, T. A., Miller, J. D., Lopez, M., & Chapman, M. D. (1989). A two-site monoclonal antibody ELISA for the quantification of the major Dermatophagoides spp. Allergens, Der p I and Der f I. Journal of Immunological Methods, 118(2), 227–235. https://doi.org/10.1016/0022-1759(89)90010-0
- Marcos, C. M., de Fátima da Silva, J., de Oliveira, H. C., Moraes da Silva, R. A., Mendes-Giannini, M. J. S., & Fusco-Almeida, A. M. (2012). Surface-expressed enolase contributes to the adhesion of paracoccidioides brasiliensis to host cells. FEMS Yeast Research, 12(5), 557–570. https://doi.org/10.1111/j.1567-1364.2012.00806.x
- Matricardi, P. M., Kleine-Tebbe, J., Hoffmann, H. J., Valenta, R., Hilger, C., Hofmaier, S., Aalberse, R. C., Agache, I., Asero, R., Ballmer-Weber, B., Barber, D., Beyer, K., Biedermann, T., Bilò, M. B., Blank, S., Bohle, B., Bosshard, P. P., Breiteneder, H., Brough, H. A., … Ollert, M. (2016). EAACI molecular allergology user's guide. Pediatric Allergy and Immunology, 27(Suppl 23), 1–250. https://doi.org/10.1111/pai.12563
- Morales-Amparano, M. B., Huerta-Ocampo, JÁ, Pastor-Palacios, G., & Teran, L. M. (2021). The role of enolases in allergic disease. The Journal of Allergy and Clinical Immunology: In Practice, 9(8), 3026–3032. https://doi.org/10.1016/j.jaip.2021.04.005
- Nakagawa, T., & Nagayama, F. (1989). Enzymatic properties of fish muscle aldolase. Comparative Biochemistry and Physiology Part B: Comparative Biochemistry, 92(2), 405–410. https://doi.org/10.1016/0305-0491(89)90301-5
- Parenti, L. R., & Weitzman, S. H. (2021, Jun 4). “Fish”. Encyclopedia Britannica. Retrieved October 31, 2021. https://www.britannica.com/animal/fish.
- Piast, M., Kustrzeba-Wójcicka, I., Matusiewicz, M., & Banaś, T. (2005). Molecular evolution of enolase. Acta Biochimica Polonica, 52(2), 507–513. https://doi.org/10.18388/abp.2005_3466
- Pietkiewicz, J., Danielewicz, R., Bednarz-Misa, I. S., Ceremuga, I., Wiśniewski, J., Mierzchala-Pasierb, M., Bronowicka-Szydełko, A., Ziomek, E., & Gamian, A. (2018). Experimental and bioinformatic approach to identifying antigenic epitopes in human α- and β-enolases. Biochemistry and Biophysics Reports, 15, 25–32. https://doi.org/10.1016/j.bbrep.2018.05.008
- Pietkiewicz, J., Kustrzeba-Wójcicka, I., & Wolna, E. (1983). Purification and properties of enolase from carp (Cyprinus carpio). comparison with enolases from mammals’ muscles and yeast. Comparative Biochemistry and Physiology Part B: Comparative Biochemistry, 75(4), 693–698. https://doi.org/10.1016/0305-0491(83)90118-9
- Rahman, M. M. (2015). Role of common carp (Cyprinus carpio) in aquaculture production systems. Frontiers in Life Science, 8(4), 399–410. https://doi.org/10.1080/21553769.2015.1045629
- Reuten, R., Nikodemus, D., Oliveira, M. B., Patel, T. R., Brachvogel, B., Breloy, I., Stetefeld, J., Koch, M., & Riggs, P. D. (2016). Maltose-Binding Protein (MBP), a secretion-enhancing Tag for Mammalian protein expression systems. PLoS One, 11(3), e0152386. https://doi.org/10.1371/journal.pone.0152386
- Ruethers, T., Taki, A. C., Nugraha, R., Cao, T. T., Koeberl, M., Kamath, S. D., Williamson, N. A., O'Callaghan, S., Nie, S., Mehr, S. S., Campbell, D. E., & Lopata, A. L. (2019). Variability of allergens in commercial fish extracts for skin prick testing. Allergy, 74(7), 1352–1363. https://doi.org/10.1111/all.13748
- Sharp, M. F., & Lopata, A. L. (2014). Fish allergy: In review. Clinical Reviews in Allergy & Immunology, 46(3), 258–271. https://doi.org/10.1007/s12016-013-8363-1
- Simon-Nobbe, B., Probst, G., Kajava, A. V., Oberkofler, H., Susani, M., Crameri, R., Ferreira, F., Ebner, C., & Breitenbach, M. (2000). IgE-binding epitopes of enolases, a class of highly conserved fungal allergens. Journal of Allergy and Clinical Immunology, 106(5), 887–895. https://doi.org/10.1067/mai.2000.110799
- Singh, A. B. (2018). Allergen preparation and standardization: An update. Global Journal of Otolaryngology, 17(4), 555968. https://doi.org/10.19080/GJO.2018.17.555968
- Stephen, J. N., Sharp, M. F., Ruethers, T., Taki, A., Campbell, D. E., & Lopata, A. L. (2017). Allergenicity of bony and cartilaginous fish - molecular and immunological properties. Clinical & Experimental Allergy, 47(3), 300–312. https://doi.org/10.1111/cea.12892
- Swoboda, I., Bugajska-Schretter, A., Verdino, P., Keller, W., Sperr, W. R., Valent, P., Valenta, R., & Spitzauer, S. (2002). Recombinant carp parvalbumin, the major cross-reactive fish allergen: A tool for diagnosis and therapy of fish allergy. The Journal of Immunology, 168(9), 4576–4584. https://doi.org/10.4049/jimmunol.168.9.4576
- Thomas, P., Battifora, H., Manderino, G. L., & Patrick, J. (1987). A monoclonal antibody against neuron-specific enolase. Immunohistochemical comparison with a polyclonal antiserum. American Journal of Clinical Pathology, 88(2), 146–152. https://doi.org/10.1093/ajcp/88.2.146
- Tomm, J. M., van Do, T., Jende, C., Simon, J. C., Treudler, R., von Bergen, M., & Averbeck, M. (2013). Identification of new potential allergens from Nile perch (Lates niloticus) and cod (Gadus morhua). Journal of Investigational Allergology and Clinical Immunology, 23(3), 159–167.
- Valenta, R., Karaulov, A., Niederberger, V., Zhernov, Y., Elisyutina, O., Campana, R., Focke-Tejkl, M., Curin, M., Namazova-Baranova, L., Wang, J.-Y., Pawankar, R., & Khaitov, M. (2018). Allergen extracts for In vivo diagnosis and treatment of allergy: Is there a future? The Journal of Allergy and Clinical Immunology: In Practice, 6(6), 1845–1855.e2. https://doi.org/10.1016/j.jaip.2018.08.032
- Wagner, S., Breiteneder, H., Simon-Nobbe, B., Susani, M., Krebitz, M., Niggemann, B., Brehler, R., Scheiner, O., & Hoffmann-Sommergruber, K. (2000). Hev b 9, an enolase and a new cross-reactive allergen from hevea latex and molds. Purification, characterization, cloning and expression. European Journal of Biochemistry, 267(24), 7006–7014. https://doi.org/10.1046/j.1432-1327.2000.01801.x
- Witkowska, D., Pietkiewicz, J., Szostko, B., Danielewicz, R., Masaowski, L., & Gamian, A. (2005). Antibodies against human muscle enolase recognize a 45-kDa bacterial cell wall outer membrane enolase-like protein. FEMS Immunology & Medical Microbiology, 45(1), 53–62. https://doi.org/10.1016/j.femsim.2005.01.005
- Yadav, R. K., & Shukla, P. K. (2019). A novel monoclonal antibody against enolase antigen of Aspergillus fumigatus protects experimental aspergillosis in mice. FEMS Microbiology Letters, 366(3), 1–6. https://doi.org/10.1093/femsle/fnz015.
- Zvirbliene, A., Pleckaityte, M., Lasickiene, R., Kucinskaite-Kodze, I., & Zvirblis, G. (2010). Production and characterization of monoclonal antibodies against vaginolysin: Mapping of a region critical for its cytolytic activity. Toxicon, 56(1), 19–28. https://doi.org/10.1016/j.toxicon.2010.03.007