ABSTRACT
Cypress tree (Chamaecyparis obtusa) bark is well-known for its bio-functional activities and high content of polyphenol and flavonoids. It has previously exhibited antioxidant, anti-pathogenic, and anti-inflammatory activities. Our study aimed to investigate the anti-melanogenic effect of Cypress Tree Bark extract (CBE). We evaluated cellular tyrosinase activity and melanin content in alpha-melanocyte-stimulating hormone (α-MSH) induced B16F10 murine melanoma cells. We analyzed microphthalmia-associated transcription factor (MITF), tyrosinase-related protein (TRP1 and TRP2), and cAMP response element-binding protein (CREB) activation via phosphorylation of AKT and ERK using western blot analysis. Tyrosinase, MITF, TRP1, and TRP2 mRNA expression were examined via real-time polymerase chain reaction. CBE restored melanin content and tyrosinase activity remarkably in α-MSH stimulated melanoma cells. It exhibited an anti-melanogenic effect through suppressing MITF, TRP1, TRP2, tyrosinase mRNA and protein expression in α-MSH-induced B16F10 cells. Furthermore, CBE has significantly inhibited CREB activation by suppressing AKT and extracellular signal-regulated kinase phosphorylation. Our data strongly suggest that CBE has potential effects against melanogenesis.
GRAPHICAL ABSTRACT
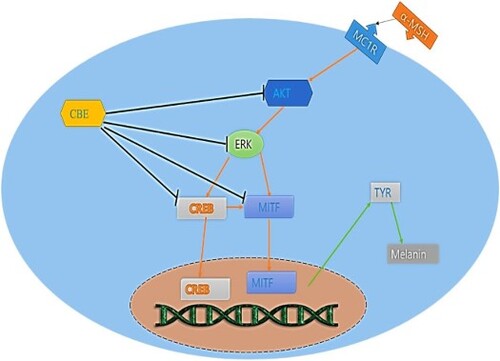
1. Introduction
Cypress tree (Chamaecyparis obtusa) is one of the seven species of Chamaecyparis that is found in East Asia. It is widely cultivated for its high-quality timber and its bark is a dark reddish-brown (Marimuthu et al., Citation2008). An emerging area of research is the investigation of plant materials as sources of natural bioactive compounds that could be used as therapeutic agents against disease (Bayazid et al., Citation2020; Joung et al., Citation2020). Cypress tree bark extract (CBE) has shown many bio-functional activities in previous studies, including antioxidant, anti-cancer, and dermatological activities (Joung et al., Citation2020; Marimuthu et al., Citation2008). CBE is rich in flavonoids, polyphenols, and other bioactive compounds. To the best of our knowledge, this is the first study of CBE on anti-melanogenic effects.
Melanogenesis is a physiological process that synthesizes melanin to protect skin and determines skin, eye, and hair colour. However, excessive melanin synthesis through melanogenesis causes abnormal skin pigmentation, esthetic concerns, and melanoma (Eghbali-Feriz et al., Citation2018; Ko et al., Citation2021). Melanin is produced in melanocytes and distributed among keratinocytes in response to many intrinsic and extrinsic responses. Among these, α-melanocyte stimulating hormone (α-MSH) is the key intrinsic factor in melanogenesis (Jiang et al., Citation2021; Lee et al., Citation2010). α-MSH activates the cAMP response element-binding protein (CREB) pathway that mediates microphthalmia-associated transcription factor (MITF). MITF is a pivotal mediator to stimulate tyrosinase (TYR) through tyrosinase-related proteins (TRP1 and TRP2) that upregulate melanin synthesis (Jiang et al., Citation2021). Furthermore, extracellular signal-regulated kinase (ERK) and AKT play large roles in melanogenic signalling through activating CREB in the MITF pathway, which perpetuates melanin biosynthesis (Ditlevsen et al., Citation2008; Jiang et al., Citation2021). To study melanogenesis and anti-melanogenic effects, murine melanoma cell lines (B16F10 cells) are widely used (Eghbali-Feriz et al., Citation2018; Jiang et al., Citation2021; Yao et al., Citation2015). Cellular tyrosinase activity inhibition in α-MSH-induced melanocytes could be a potential indicator of anti-melanogenic effects and extensively control hyperpigmentation. To elucidate the molecular mechanism, attenuating TRP-1, and TRP-2 expressions through MITF signalling pathways initiated by upregulating CREB in α-MSH-induced melanocytes could be the potent and robust anti-melanogenic effects of CBE.
In this study, we investigated the potential effects of CBE against melanogenesis and hyperpigmentation in α-MSH-induced B16F10 cells; these effects have envisaged promising outcomes for further study. The cellular safety of 70% ethanolic extract of CBE has been evaluated by MTT assay. The melanin content and tyrosinase activity have been evaluated with underlying molecular elucidation through TRP-1, TRP-, MITF, and CREB signalling pathways. The quercetin and hinokinin content of CBE are potentially highly bioactive compounds examined by high pressured high-performance liquid chromatography (HPLC) analysis.
2. Materials and methods
2.1. Chemicals and reagents
α-Melanocyte stimulating hormone (α-MSH), and 3-(4,5-dimethylthiazol-2-yl)-2,5 diphenyltetrazolium bromide (MTT) were purchased from Sigma Aldrich. DMEM (Dulbecco’s Modified Eagle’s Medium), DPBS, Penicillin Streptomycin (P.S), and Trypsin EDTA were purchased from WELGENE Inc. (Seoul, Korea). Western blot primary and secondary antibodies were collected from Cell Signalling Technology or Abcam (Cambridge, UK). All other chemicals were analytical-grade and used without any further purification.
2.2. Sample preparation
Dried cypress tree bark (600 g) was extracted with 99% ethanol (1:9 v/v) three times for 24 h at room temperature (22–24°C). The solution was passed through a paper filter (Whatman No. 2; GE, Tokyo, Japan), the extract was concentrated under reduced pressure using a vacuum rotary evaporator (N-3010; EYELA, Tokyo, Japan), and lyophilized (MCFD8508; IlShinBioBase, Dongducheon, Korea) for 96 h. A total of 86.04 g of cypress bark ethanol-extract powder (yield of 14.34%) was obtained. The extracted sample was stored at −20°C before use.
2.3. Cell culture
B16F10 cells are a murine melanoma cell line obtained from the Korean cell line bank. B16F10 cells were maintained in DMEM supplemented with 10% FBS and 1% P.S and incubated in a controlled humidified atmosphere at 37°C with 5% CO2.
2.4. Cell viability
The cell viability assay of cypress bark extract (CBE) was evaluated in the B16F10 cell line by MTT assay. B16F10 cells were seeded as 1 × 104 cells per well in a 96-well plate for 24 h to assess the cell viability of CBE as previously described (Bayazid et al., Citation2021; Mijan et al., Citation2019) with slight modifications. After 24 h of incubation, the cells were treated with various concentrations of CBE with or without α-MSH (200 nM), and the normal group was treated with only media for another 24 h. Then, MTT was added as 500 µg/mL as the final concentration and incubated for 2 h. Then the formazan blue was dissolved in DMSO and absorbance was taken at 570 nm in the well-plate reader (Molecular Device, USA).
2.5. Cellular tyrosinase activity
The cellular tyrosinase activity assay was performed as previously described (Eghbali-Feriz et al., Citation2018) with some changes. The cells were seeded as 1 × 106 cells/ well in a 6-well plate. The cells were pre-treated for 1 h with various concentrations of CBE (5, 10 and 20 µg/mL) and then incubated with α-MSH (200 nM) for another 48 h. Then the cellular tyrosinase activity was analyzed by L-DOPA substrate and absorbance was taken at 475 nm (Yao et al., Citation2015).
2.6. Melanin content
Melanin content of CBE was investigated in α-MSH-induced B16F10 cells as reported in previous studies (Bayazid & Jang, Citation2021; Wolnicka-Glubisz et al., Citation2015) with some changes. B16F10 cells were seeded as 1 × 106 cells per well in 6-well plate. The following day, the cells were pre-treated with various concentrations of CBE (5, 10 and 20 µg/mL) for 1 h and then incubated with α-MSH (200 nM) for another 48 h. After PBS washing, cell lysates were collected and analyzed for the melanin content.
2.7. Morphological staining
B16F10 cells (1 × 104) were dispensed into each well of the Chamber Slide culture chambers (Nunc, Naperville, IL), cultured for 24 h, and then treated with 20 µg/ml of extract and α-MSH (100 nM). After incubation for 48 h, the cells were fixed with 5% formyl saline solution, washed, and reacted at room temperature for 4 h with a 0.1 M PBS (pH 7.2) solution containing 0.1% L-dihydroxyphenylalanine (L-DOPA, Sigma). It was reconstituted with 10% formyl saline, dehydrated with 50%, 70%, 95%, and 100% alcohol, and observed with an optical microscope.
2.8. Western blot analysis
B16F10 cells were seeded as 1 × 106 cells in each well in a 6-well plate for 24 h. Then, cells were pre-treated with CBE for 1 h and α-MSH (200 nM) induced for 48 h (Tyrosinase, TRP-1, TRP-2, β-actin), for 4 h (MITF), and 1 h for Akt and CREB. After fixing by ice-cold PBS, cell proteins were isolated using PRO-PREP buffer (iNtRON Biotechnology) and quantified by Bio-Red protein assay dye. Equal amounts of proteins were electrophorized in SDS-page gel and transferred to a PVDF membrane. The membranes were blocked by 5% BSA for 1 h at room temperature. After blocking the membranes, the membranes were incubated with the corresponding antibody overnight at 4°C, then the membranes were incubated with the secondary antibody (anti-rabbit) at room temperature for 1 h (Alim et al., Citation2019; Bayazid et al., Citation2022). The protein expression images were detected by Bio-Red Chemi-docs XRS UV camera using enhanced chemiluminescence (ECL), and the band intensities were analyzed by ImageJ.
2.9. Real-time polymerase chain reaction (RT-PCR) analysis
B16F10 cells (1 × 106) were dispensed into each well in a 6-well plate. After 24 h of incubation, cells were pre-treated with CBE for 1 h and α-MSH (200 nM) induced for 48 h. Then, the total RNA was extracted by TRIzol (Life Technologies, CA, USA) and an equal amount of RNA was reverse transcribed by cDNA synthesis kit (Bayazid et al., Citation2020, Citation2022; Escoter-Torres et al., Citation2020; Mijan et al., Citation2019; Pervin et al., Citation2016). A real-time polymerase chain reaction (PCR) was performed using PowerUp SYBR Green Master Mix. The primer sequences used in the study are described in .
Table 1. The sequences of the primers of the MITF, TRP-1, TRP-2, tyrosinase and GAPDH for B16 melanoma cells.
2.10. Immunofluorescence assay
B16F10 cells were plated at a density of 1 × 103 cells per well in an 8-well Lab-Tek chamber (NalgeNunc, Rochester, NY, USA) and incubated for 24 h. Cells were then exposed to 200 nM α-MSH for 1 h and then treated with increasing concentrations of the CBE extract for 48 h. The wells were assigned to five different treatment conditions: Normal, α-MSH only, CBE (5, 10, 20 µg/mL) with α-MSH. The samples were then washed three times with PBS, treated with 0.5% Triton X-100 for 10 min, then 1% BSA was added and incubated for 1 h. After incubation with primary anti-MITF (1:500) in blocking solution at 4°C overnight, the cells were treated with the Alexa Fluor 488-conjugated goat anti-mouse IgG secondary antibody (Invitrogen, Carlsbad, CA, USA). Excess unbound secondary antibody was removed by washing three times, and the cells were incubated with 200 μL 4′6-diamidino-2-phenylindole (DAPI) for 1 h (Bayazid et al., Citation2021). After immunostaining, the chamber was removed, the mounting solution was added to the slide, and the cover slip was fixed. The cells were then observed under a fluorescence microscope (Eclipse Ti-U, Nikon, Japan).
2.11. Determination of quercetrin and hinokinin by HPLC analysis
Bioactive compounds of Cypress tree extract were analyzed on Shimadzu Nexera XR series consisting of a C18 analytical column (Zorbax Eclipse Plus C18, 4.6 × 250 mm, 5 µm). To identify bioactive compounds, acetonitrile was used as mobile phase A and water was used as mobile phase B (elution conditions: 0 min, 60% B; 0–18 min, 40% B; 18–20 min, 60% B; flowrate,1.0 mL/min; injection volume, 10 μL; wavelength, 330 nm and column temperature 30°C). Before injecting, standards and sample were dissolved in methanol and filtered through a 0.45 μM membrane filter. All solvents and mobile phases were HPLC-grade. The retention time of quercetrin was 2.9 min. The Hinokinin detection condition of HPLC is described in .
Table 2. Hinokinin HPLC analysis condition.
2.12. Statistical analysis
All the histograms were performed at least three times. The results were exhibited as mean ± SD. Data analysis was conducted with Microsoft Excel and GraphPad Prism (5th edition) using analysis of variance (ANOVA) according to Tukey’s Multiple Comparison Test. p-value lower than 0.05 was considered statistically significant. All experiments were conducted at least three times.
3. Results and discussions
3.1. Effect of CBE on B16F10 cell viability, tyrosinase activity, and melanin content
Cell viability of CBE in B16F10 cells was evaluated by MTT assay to determine the safe dose of CBE for further investigations. The untreated group was considered to have 100% cell viability of control and treatment groups with up to 90% cell viability compared to the untreated group were considered to have received a safe dose of CBE in this study. CBE upto 20 µg/mL exhibited no cytotoxic effects in B16F10 cells, as shown in (a). As a result of observing the activity of tyrosinase in melanocytes using DOPA staining, the α-MSH treatment group significantly increased tyrosinase activity compared to the untreated group, and the α-MSH and extract treatment group shown tyrosinase activity compared to the α-MSH single treatment group. Melanogenesis is the physiological process by which melanin is a pigment responsible for the colour of skin is produced. Excessive melanogenesis leads to hyperpigmentation in the skin through increasing melanin content mediated by cellular tyrosinase activity (Lee et al., Citation2010; Sawant & Khan, Citation2020; Yao et al., Citation2015). Tyrosinase is a pleiotropic enzyme that facilitates melanogenesis through hydroxylase and oxidase (Ullah et al., Citation2019) and, the inhibition of tyrosinase is thus directly related to the reduction of melanin production. α-MSH drastically increased tyrosinase activity and melanin content in melanoma cells ((b, c)). While CBE restored cellular tyrosinase activity and melanin content significantly in a dose-dependent manner. The 5 µg/mL of CBE treatment reduced 80% melanin content of the α-MSH group to about 80% and CBE inhibited melanin production dose-dependently in α-MSH-induced B16F10 cells.
Figure 1. Cytotoxicity of CBE in B16F10 cells. Cells were incubated with 5, 10, 20, 40, 80, and 100 μg/ml of CBE for 48 h. (a) Cell viability was measured using MTT assay and (b) inhibitory effect on cellular tyrosinase activity and melanin contents by CBE treatment in B16F10 melanoma cells. After 1 × 106 cells were seeded. Cells were pre-treated with extract for 1 h, and then incubated with α-MSH for 48 h, (c) melanin content in the pellet was measured. Bars indicate the mean ± standard deviation of triplicate. Mean statistically significant difference at *p < 0.05 compared to the only α-MSH-treated group for figures (b) and (c). Control is untreated samples.
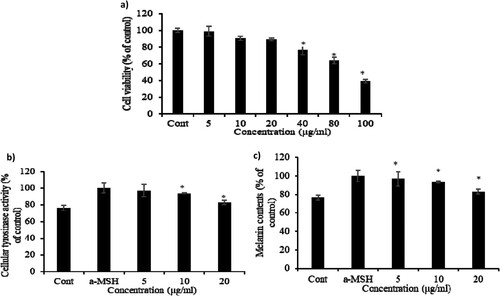
3.2. Effect of CBE on MITF, TRP-1, and TRP-2 protein expression in MSH-stimulated melanoma cells
To investigate the molecular mechanism of CBE in regulating melanin generation, we examined melanogenic protein expressions. Melanin is formed by tyrosinase activity through activating tyrosinase-related proteins (TRP1, TRP2). Microphthalmia-associated transcription factor (MITF) is the pivotal transcription factor (Ullah et al., Citation2019) regulating the transcription of melanogenic enzymes like TRP-11 and TRP-2 (Lee et al., Citation2019). MITF plays the central role in cellular melanin overproduction by regulating TRP expressions (Yu et al., Citation2021). An increase in MITF upregulates melanin synthesis by affecting the biogenesis genes of melanosomes (Shen et al., Citation2018). α-MSH notably increased MITF through the MC1 receptor and perpetually overexpressed the tyrosinase, TRP1, and TRP2 protein expressions demonstrated in , which has detrimental effects in the skin. The 1 h pre-treatment of CBE reversed the MITF, TRP-1, TRP-2 and tyrosinase expression on α-MSH-stimulated B16F10 melanoma cells.
Figure 2. (a) Morphological alterations, after treatment with 5, 10 and 20 μg/ml of CBE, were analyzed by light microscopy after 48 h. Magnification, 40×, (b) After 1 × 106 cells were seeded and cells were pre-treated with extract for 1 h, and then incubated for 48 h of alpha-Melanocyte-stimulating hormone (α-MSH) for Tyrosinase, Tyrosinase-related protein 1 (TRP-1) Tyrosinase-related protein 2 (TRP-2), β-actin, and 4 h for Melanocyte Inducing Transcription Factor (MITF). Proteins were detected by Western blotting analysis with their own antibodies. Protein expression is involved in melanin biosynthesis; proteins’ expression was evaluated by Western blot. Mean statistically significant difference at *p < 0.05, compared to the only α-MSH-treated group. Control is untreated samples.
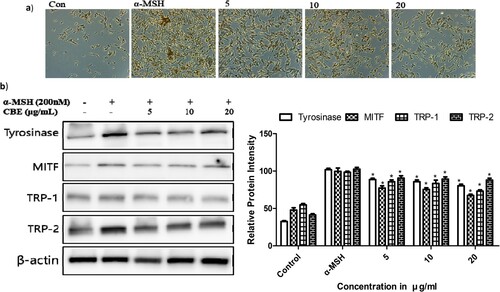
3.3. Effect of CBE on the expression of melanin synthesis genes
We investigated for further confirmation of the effect of CBE on changes in melanogenic gene expressions using RT-PCR. TRP-1, TRP-2, and tyrosinase are known to be involved in melanogenesis, which is regulated by MITF. tyrosinase key regulatory factor of tyrosinase, MITF plays a central role synthesis of hyperpigmentation and melanogenesis. The tyrosinase, TRP1, TRP2, and MITF mRNA expressions were normalized by the GAPDH housekeeping gene. Wherein α-MSH upregulated MITF, TRP-1, and TRP-2 mRNA expressions, and subsequently tyrosinase expression (). CBE significantly reduced MITF, TRP1, TRP2, and tyrosinase expression-key mediators of melanogenesis in α-MSH-stimulated B16F10 cells.
Figure 3. Effect of CBE on mRNA expression of MITF and its downstream genes tyrosinase, TYRP-1 and TYRP-2, upon α-MSH stimulation. After 1 × 106 cells were seeded and cells were pre-treated with extract for 1 h, and then incubated with α-MSH for 48 h (Tyrosinase, TRP-1, TRP-2), and 4 h for (MITF). Mean statistically significant difference at *p < 0.05, compared to the only α-MSH-treated group. Con (control) is untreated samples.
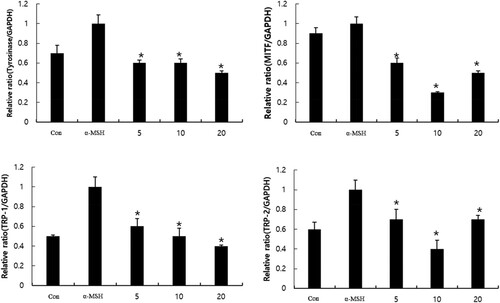
3.4. Effect of CBE on CREB activation through ERK, and AKT phosphorylation
Melanogenesis is a crucial process that remains to be understood. MITF responds to several signals including ERK, AKT, CREB phosphorylation, and so on to facilitate melanogenesis in pigment cells (Jin et al., Citation2012). The transcription factor cAMP response element-binding protein (CREB) stimulates MITF and upregulated melanogenesis by direct binding with MITF (Fu et al., Citation2019). Moreover, CREB activation enhances melanin content (Kim et al., Citation2020). AKT and ERK phosphorylation target CREB to activate, which perpetuates melanogenesis through MITF. ERK, AKT, and CREB phosphorylation increased when α-MSH was induced and downregulated significantly by CBE pre-treatment as shown in .
Figure 4. Inhibitory effects of CBE on activation of AKT/ERK and CREB signalling proteins of melanogenesis in B16F10 melanoma cells. After 1 × 106 cells were seeded and cells were pre-treated with extract for 1 h, and then incubated with α-MSH for 1 h. Proteins were detected by Western blotting analysis with targeted antibodies, protein expression involved in melanin biosynthesis; proteins’ expression was evaluated by Western blot. Mean statistically significant difference at *p < 0.05, compared to the only α-MSH-treated group. Con (control) is untreated samples.
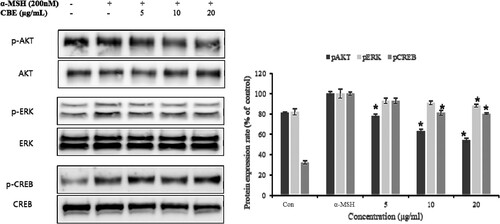
3.5. Effect of CBE on the MITF in α-MSH-induced B16F10 Cells
MITF expression was found to be highest in the α-MSH group and CBE decreased the MITF expression in α-MSH-induced B16F10 cells as shown in . The green fluorescence depicts the MITF and DAPI (blue) indicates the nucleus and merged images have been shown for comparison. α-MSH stimulation increased melanogenesis, whereas CBE suppressed MITF expression in a dose-dependent manner in α-MSH-induced B16F10 cells. In this investigation, we found that CBE restored MITF expression in α-MSH-induced B16F10 cells via Fluorescence microscopy.
Figure 5. Immunofluorescence (IF) assay to evaluate MITF expression. The expression of MITF was evaluated by IF staining using specific anti-MITF antibodies. 4′,6-diamidino-2-phenylidole (DAPI) nuclear staining is shown in blue and fluorescein isothiocyanate (FITC) staining appears in green. 1 × 103 B16F10 cells were plated in serum-free medium for 1 h at 20∼25°C and treated with AE for 48 h. The specimens were photographed using DP Controller software (×200 magnification).
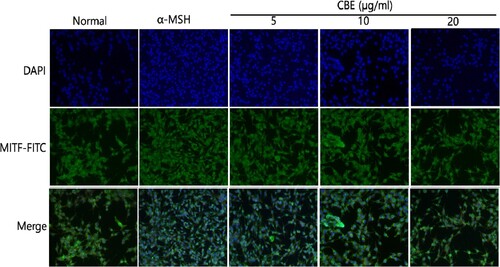
3.6. Detection of quercetrin and hinokinin in CBE by HPLC analysis
Quercetrin and Hinokinin are plant secondary metabolites that exhibit many bio-functional activities. Quercetrin and Hinokinin are both potent bioactive compounds that have demonstrated many dermatological effects (Kaur et al., Citation2017) and Quercetrin has anti-melanogenesis effects (Choi & Shin, Citation2016). However, Quercetrin and Hinokinin were detected using different HPLC methods. We detected quercetrtin is 4.8485 mg per gram of CBE and Hinokinin is about 0.536 mg per gram of extract respectively (shown in ). The presence of Quercetrin and Hinokinin in CBE assures anti-melanogenic effects in addition to other bio-functional activities.
4. Conclusion
In this study, we investigated the anti-melanogenic effects of CBE which contains Quercetrin, Hinokinin, and other bioactive compounds. CBE restored melanin content and tyrosinase activity notably in the α-MSH-induced melanoma cell. CBE also suppressed the MITF and CREB activation. Altogether, our results suggest that CBE could prevent melanogenesis and envisaged promising outcomes in the animal disease model study.
Disclosure statement
No potential conflict of interest was reported by the author(s).
References
- Alim, I., Caulfield, J. T., Chen, Y., Swarup, V., Geschwind, D. H., Ivanova, E., & Ratan, R. R. (2019). Selenium drives a transcriptional adaptive program to block ferroptosis and treat stroke. Cell, 177(5), 1262–1279.e1225. https://doi.org/10.1016/j.cell.2019.03.032
- Bayazid, A. B., & Jang, Y. A. (2021). The role of andrographolide on skin inflammations and modulation of skin barrier functions in human keratinocyte. Biotechnology and Bioprocess Engineering, 26(5), 804–813. https://doi.org/10.1007/s12257-020-0289-x
- Bayazid, A. B., Jang, Y. A., Kim, Y. M., Kim, J. G., & Lim, B. O. (2021). Neuroprotective effects of sodium butyrate through suppressing neuroinflammation and modulating antioxidant enzymes. Neurochemical Research, 46(9), 2348–2358. https://doi.org/10.1007/s11064-021-03369-z
- Bayazid, A. B., Kim, J. G., Azam, S., Jeong, S. A., Kim, D. H., Park, C. W., & Lim, B. O. (2022). Sodium butyrate ameliorates neurotoxicity and exerts anti-inflammatory effects in high fat diet-fed mice. Food and Chemical Toxicology, 159, 112743. https://doi.org/10.1016/j.fct.2021.112743
- Bayazid, A. B., Park, S. H., Kim, J. G., & Lim, B. O. (2020). Green chicory leaf extract exerts anti-inflammatory effects through suppressing LPS-induced MAPK/NF-κB activation and hepatoprotective activity in vitro. Food Agricultural Immunology, 31(1), 513–532. https://doi.org/10.1080/09540105.2020.1742667
- Choi, M.-H., & Shin, H.-J. (2016). Anti-melanogenesis effect of quercetin. Cosmetics, 3(2), 18. https://doi.org/10.3390/cosmetics3020018
- Ditlevsen, D. K., Owczarek, S., Berezin, V., & Bock, E. (2008). Relative role of upstream regulators of Akt, ERK and CREB in NCAM-and FGF2-mediated signalling. Neurochemistry International, 53(5), 137–147. doi:10.1016/j.neuint.2008.06.011
- Eghbali-Feriz, S., Taleghani, A., Al-Najjar, H., Emami, S., Rahimi, H., Asili, J., & Tayarani-Najaran, Z. (2018). Anti-melanogenesis and anti-tyrosinase properties of Pistacia atlantica subsp. mutica extracts on B16F10 murine melanoma cells. Research in Pharmaceutical Sciences, 13(6), 533–545. https://doi.org/10.4103/1735-5362.245965
- Escoter-Torres, L., Greulich, F., Quagliarini, F., Wierer, M., & Uhlenhaut, N. H. (2020). Anti-inflammatory functions of the glucocorticoid receptor require DNA binding. Nucleic Acids Research, 48(15), 8393–8407. https://doi.org/10.1093/nar/gkaa565
- Fu, T., Chai, B., Shi, Y., Dang, Y., & Ye, X. (2019). Fargesin inhibits melanin synthesis in murine malignant and immortalized melanocytes by regulating PKA/CREB and P38/MAPK signaling pathways. Journal of Dermatological Science, 94(1), 213–219. https://doi.org/10.1016/j.jdermsci.2019.03.004
- Jiang, L., Huang, J., Hu, Y., Lei, L., Ouyang, Y., Long, Y., & Yang, Y. (2021). Identification of the ceRNA networks in α-MSH-induced melanogenesis of melanocytes. Aging, 13(2), 2700. https://doi.org/10.18632/aging.202320
- Jin, M. L., Park, S. Y., Kim, Y. H., Park, G., Son, H.-J., & Lee, S.-J. (2012). Suppression of α-MSH and IBMX-induced melanogenesis by cordycepin via inhibition of CREB and MITF, and activation of PI3K/Akt and ERK-dependent mechanisms. International Journal of Molecular Medicine, 29(1), 119–124.
- Joung, Y.-W., Kim, Y.-m., & Jang, Y.-A. (2020). Studies on the antioxidant and whitening effects of Chamaecyparis obtusa extract. Journal of the Korean Applied Science Technology, 37(6), 1496–1506.
- Kaur, N., Kaur, B., & Sirhindi, G. (2017). Phytochemistry and pharmacology of Phyllanthus niruri L.: a review. Phytotherapy Research, 31(7), 980–1004. https://doi.org/10.1002/ptr.5825
- Kim, J.-H., Hong, A.-r., Kim, Y.-H., Yoo, H., Kang, S.-W., Chang, S. E., & Song, Y. (2020). JNK suppresses melanogenesis by interfering with CREB-regulated transcription coactivator 3-dependent MITF expression. Theranostics, 10(9), 4017. https://doi.org/10.7150/thno.41502
- Ko, H.-H., Chang, Y.-T., Kuo, Y.-H., Lin, C.-H., & Chen, Y.-F. (2021). Oenothera laciniata Hill extracts exhibits antioxidant effects and attenuates melanogenesis in B16-F10 cells via downregulating CREB/MITF/tyrosinase and upregulating p-ERK and p-JNK. Plants, 10(4), 727. https://doi.org/10.3390/plants10040727
- Lee, J.-H., Jang, J.-Y., Park, C., Kim, B.-W., Choi, Y.-H., & Choi, B.-T. (2010). Curcumin suppresses α-melanocyte stimulating hormone-stimulated melanogenesis in B16F10 cells. International Journal of Molecular Medicine, 26(1), 101–106.
- Lee, J. Y., Cho, Y.-R., Park, J. H., Ahn, E.-K., Jeong, W., Shin, H. S., & Oh, J. S. (2019). Curcumin suppresses α-melanocyte stimulating hormone-stimulated melanogenesis in B16F10 cells. Toxicology Reports, 6, 10–17.
- Marimuthu, P., Wu, C. L., Chang, H. T., Chang, S. T., & Agriculture. (2008). Antioxidant activity of the ethanolic extract from the bark of Chamaecyparis obtusa var. Formosana. Journal of the Science of Food, 88(8), 1400–1405. https://doi.org/10.1002/jsfa.3231
- Mijan, M. A., Kim, J. Y., Moon, S.-Y., Choi, S.-H., Nah, S.-Y., & Yang, H.-J. (2019). Gintonin enhances proliferation, late stage differentiation, and cell survival from endoplasmic reticulum stress of oligodendrocyte lineage cells. Frontiers in Pharmacology, 10, 1211. https://doi.org/10.3389/fphar.2019.01211
- Pervin, M., Hasnat, M. A., Lim, J.-H., Lee, Y.-M., Kim, E. O., Um, B.-H., & Lim, B. O. (2016). Preventive and therapeutic effects of blueberry (Vaccinium corymbosum) extract against DSS-induced ulcerative colitis by regulation of antioxidant and inflammatory mediators. Journal of Nutritional Biochemistry, 28, 103–113. https://doi.org/10.1016/j.jnutbio.2015.10.006
- Sawant, O., & Khan, T. (2020). Management of periorbital hyperpigmentation: An overview of nature-based agents and alternative approaches. Dermatologic Therapy, 33(4), e13717. https://doi.org/10.1111/dth.13717
- Shen, J., Huang, D., Sun, C., Li, J., & Bai, Z. (2018). Cloning of a microphthalmia-associated transcription factor gene and its functional analysis in nacre formation and melanin synthesis in Hyriopsis cumingii. Aquaculture and Fisheries, 3(6), 217–224. https://doi.org/10.1016/j.aaf.2018.09.004
- Ullah, S., Park, C., Ikram, M., Kang, D., Lee, S., Yang, J., & Moon, H. R. (2019). Tyrosinase inhibition and anti-melanin generation effect of cinnamamide analogues. Bioorganic Chemistry, 87, 43–55. https://doi.org/10.1016/j.bioorg.2019.03.001
- Wolnicka-Glubisz, A., Nogal, K., Żądło, A., & Płonka, P. (2015). Curcumin does not switch melanin synthesis towards pheomelanin in B16F10 cells. Archives of Dermatological Research, 307(1), 89–98. https://doi.org/10.1007/s00403-014-1523-1
- Yao, C., Jin, C. L., Oh, J. H., Oh, I. G., Park, C. H., & Chung, J. H. (2015). Ardisia crenata extract stimulates melanogenesis in B16F10 melanoma cells through inhibiting ERK1/2 and Akt activation. Molecular Medicine Reports, 11(1), 653–657. https://doi.org/10.3892/mmr.2014.2697
- Yu, F., Lu, Y., Zhong, Z., Qu, B., Wang, M., Yu, X., & Chen, J. (2021). Mitf involved in innate immunity by activating tyrosinase-mediated melanin synthesis in Pteria penguin. Frontiers in Immunology, 12, 626493. https://doi.org/10.3389/fimmu.2021.626493